Anju Garg, Ritu Nair Misra, Ankita Aggarwal, M. Sarthak Swarup There are dark shadows on the earth but its lights are stronger in the contrast Charles Dickens Contrast agents (CAs) are substances that increase the visibility of inner structures of the body and the differentiation of abnormal from normal tissue, thus enhancing the diagnostic ability of the radiologist. In 1896, inspired air was first recognized as an endogenous radiographic CA in a chest radiograph. Iodine was recognized as a potential exogenous CA, providing high radioopacity as early as 1920. With the increasing use of the imaging modalities for various diagnostic and interventional procedures, the variety of CAs has also increased manifold. Over the years, there have been many developments to improve the safety and tolerability profile of the CAs. An ideal CA needs to be nontoxic, noncarcinogenic, easy to administer, well-tolerated and rapidly eliminated. It should be stable with low osmolality and viscosity, get concentrated in desired area of interest and be cost effective. CAs can be broadly classified according to their use in various modalities: After iodine was recognized as a potential CA in 1920, various compounds of iodine were developed for use in the human body. In 1923, the first angiography and urography were performed using sodium iodide; however, it was found to be very toxic, with poor contrast and therefore unsuitable for intravenous use. The binding of iodine to organic molecules was found to reduce the toxicity, and the first organic salt “uroselectan” was developed in the late 1920s by Dr Moses Swick, which was used in urography. Its major drawback was poor radiographic contrast, as each molecule was bound to only one atom of iodine. A diiodinated N-pyridone compound “diodrast” was next developed and used as the main urographic CA from 1930s till the 1950s. In the 1950s, sodium and meglumine salts of triiodiobenzoic acid came into use as CAs. For the next 20 years, these diatrizoates (Urografin) and iothalamates (Conray) were used as intravascular CAs. These were the ionic CAs and they had a high osmolality (five to eight times that of blood), which led to major shift of fluid into extracellular space, resulting in side effects. In 1974, nonionic CAs were introduced. This was achieved by converting the triiodinated benzoic acid into a nonionic molecule by replacing the ionizing carboxylic acid radical (COOH) with hydrophilic radicals. Metrizamide was introduced in 1977 by Almen as the first nonionic monomer. Later, the current generation of nonionic monomers comprising iohexol, ioversol, etc. were added. In the 1980 and 1990s, nonioniic dimers, e.g. iodixanol, were developed in an effort to reduce the osmolality still further and generate an isoosmolar contrast. Iodinated CAs are divided into four categories according to the ionicity and number of benzene rings (Fig. 1.16.1). These CAs have a common benzene ring with three iodine atoms (triiodinated benzene ring). Iodine is responsible for maximum photoelectric absorption and attenuation of X-rays when exposure is made at 60–80 kVp, the average range utilized for radiography. This is due to its k shell binding energy being equal to 33.2 keV. Ionic monomers: These are the oldest group. They have a single benzene ring with iodine at positions 2,4,6. The carboxyl acid (COOH) group at position 1 makes them water soluble and the short chains of hydrocarbons at positions 3 and 5 reduce toxicity. Sodium or methylglucamine are the cations, and they dissociate to release three iodine atoms for two ionic particles. They are thus referred to as “ratio 1.5 agents”. Due to their high osmolality, they are categorized as high osmolar contrast media (HOCMs). They have high incidence of adverse effects and are therefore no longer utilized for intravascular or intrathecal use. Currently, their primary use is for nonvascular contrast opacification or for evaluation of perforations/extravasation mainly in gastrointestinal and genitourinary tracts. HOCMs are also used as a therapeutic medium in subacute gastrointestinal obstruction or obstipation as they draw water into the gastrointestinal tract (GIT), due to their high osmolality. Ionic dimers: As the name signifies, they have two benzene rings with one ionizable group and other in covalent bonding, thus releasing six iodine atoms for two ionic particles and are referred to as “ratio 3 agents”. They have less osmolality and are commonly called low osmolar contrast media (LOCM). Nonionic monomers: These have modified triiodinated benzene rings which have covalent bonding; the carboxyl group along with the cation is replaced by a nonionizing radical. Lack of COOH group reduces the chances of neural toxicity, and absence of cation results in low osmolality (LOCM). They do not release any ion on dissociation; hence, they are called nonionic. They release three iodine atoms for one particle and thus are called “ratio 3 agents”. These are water soluble due to the presence of multiple hydrophilic hydroxyl groups. Nonionic dimers: They have two nonionic benzene rings and release six iodine atoms for one particle and are thus called “ratio 6 agents”. They have the least osmolality, nearing that of plasma, and are also known as isoosmolar contrast media (IOCMs). The lower the osmolality, the less the side effects and toxicity with better tolerability. Table 1.16.1 summarizes the four categories of ICAs with examples. Modifications that reduce toxicity of iodinated CAs are as follows: After intravenous injection, 95% of the contrast is free, and only about 5% is protein bound. Almost immediately after the injection, contrast starts to diffuse into extravascular space, and in about 5 minutes, the concentration equalizes in the two compartments and reaches a state of equilibrium. This free diffusion, however, is not seen in brain (due to blood–brain barrier), other neural tissue and testes. For this reason, equilibrium phase scanning is done for most of the regions, and delayed scan is useful in areas where there are tight barriers for diffusion of contrast. The iodinated CAs are cleared by kidney through glomerular filtration and very little by tubular reabsorption. They have a half-life of 1–2 h, and almost all is excreted by 24 h. When there is derangement of kidney function, vicarious excretion occurs through bile and bowel. An insignificant amount is excreted via sweat, tears, saliva and gastric juice. Water-soluble nonionic agents such as iohexol and iopamidol are the agents currently in use for computed tomography (CT) myelography, as they are stable in solution and have lesser side effects. Earlier metrizamide (a nonionic monomer) was used but was abandoned due to high incidence of side effects. Oral and intravenous cholangiographic agents are no more used and are therefore only of historical importance. The only oily CA in current use is Lipiodol which contains active substance ethyl esters of iodized fatty acids of poppy seed oil. It is used in interventional procedures such as transarterial chemoembolization. Adequate evaluation of bowel requires its distention with an oral CA. An ideal gastrointestinal CA should be inert, nonabsorbable, nontoxic, inexpensive, insoluble in water or lipids, producing excellent coating of the mucosa. Barium sulphate preparations are used for gastrointestinal imaging. They are usually taken orally or instilled rectally or through stoma for visualization of GIT. Barium has an atomic number of 56, causing high attenuation of X-rays, and hence is highly radioopaque. Barium preparations are inert substances which do not get absorbed through the gut mucosa and thereby are free of any serious side effects. Also they can coat the mucosa uniformly for diagnosing subtle mucosal abnormalities. Barium sulphate is a micropulverized preparation that is commercially available as ready-to-use liquid form or powdered form, which has to be mixed with water. Various formulations are as follows: 100% w/v concentration, high viscosity, used in pharyngeal and esophageal examination (barium swallow). 95% w/v concentration, moderate viscosity, used for esophageal, gastric and small intestinal examination (barium meal upper GI study and barium meal follow-through). 200% w/v concentration, low viscosity. Commercially available high density barium (Microbar HD) provided in powdered formulation and has to be mixed with 70 mL of water to form a suspension. It is mainly used for double-contrast upper GI studies, the negative contrast being produced by an effervescent agent, e.g. tartaric acid or citric acid, provided along with the HD preparation. High density barium is also used for double contrast enema along with air providing the negative contrast. 30% w/v concentration. It is also in powdered form which has to be mixed with saline to form a thin solution. It is mainly used for barium enema. To make it an ideal oral solution, certain additives are used: As the solution has to be taken orally, few sweetening or flavouring agents such as saccharine or fruit essences have to be added. Erythrocin is added for giving it colour. Simethicone or methyl polysiloxone is added to prevent formation of gas bubbles, which may hinder in diagnosis as they mimic polyps in mucosa. Sodium metabisulphates are added to increase the shelf life and prevent any microbe growth. To improve coating of barium and flow of suspension. If it persists for a long duration in the bowel or in blind cavities or tracts, it can inspissate and form hard fecaliths. Other side effects of barium preparations include chemical pneumonitis if aspirated, chemical mediastinitis or peritonitis if extravasation occurs. Contraindications to barium administration include complete intestinal obstruction or paralytic ileus, suspected perforation or fistula, prior to surgery or endoscopy, toxic megacolon and presence of blind ending loops. Oral CAs are required to delineate bowel pathology in various CT examinations such as CT enterography, CT enteroclysis or CT colonography. Opacification of the bowel is also required in abdominopelvic CT examinations to distinguish the bowel from other organs or mass lesions. Oral CAs used for this purpose can be broadly categorized into positive, negative or neutral depending on the attenuation of the bowel lumen produced, as compared with endoluminal water-like contents. Positive oral CAs such as barium sulphate and iodinated compounds, produce high attenuation of the bowel lumen. Iodinated water-soluble CAs are used in concentrations of 2% for this purpose. Initially, these were considered the best for bowel pathology; however, there were two major disadvantages. Firstly, bowel wall enhancement was not well appreciated due to high endoluminal contrast, and secondly, mucosal details could not be well evaluated. Hence, they are primarily administered in abdominopelvic CT scans where extraluminal pathology is suspected. Barium preparations are contraindicated when bowel perforation or GI fistula formation needs to be ruled out. Positive CAs are best avoided in cases of bowel pathology, GI haemorrhage, acute trauma, multiphasic studies for hepatobiliary or pancreatic indications, CT urography and when there is high risk of aspiration. Neutral CAs such as water, milk or very low density barium sulphate suspension (VoLumen/NeuLumEX-0.1%w/w or w/v) produce water like attenuation of the bowel lumen. They are specifically administered when bowel pathology is suspected. They can well delineate abnormal bowel wall thickening or enhancement against low intraluminal contrast. Agents such as mannitol and sorbitol cause greater distention of the bowel lumen, thereby making the bowel wall more conspicuous. In addition, there is no streak artefact as produced by high attenuation of positive oral contrasts. Mannitol is considered excellent for causing bowel distention, delineating the mucosal fold pattern and producing homogenous endoluminal contrast as compared with water or other positive oral CAs. Mannitol is given in concentration of 2.5%, making total volume of up to 2 L to be taken at a steady rate of 150 mL every 5 min till 45–60 min before the examination. The volume administered and the time of scan acquisition vary according to the bowel segment that needs to be opacified. Negative CAs such as air or carbon dioxide, produce low attenuation of the bowel lumen. They have a limited use in CT colonography. The pioneer of contrast in ultrasound was Joyner who observed increased enhancement of intracardiac echoes with saline injection during echocardiography in the year 1960. First case report was published by Gramiak and Shah in 1968 who used intracardiac injection of indocyanine green/saline in contrast echocardiography. The compressed gas in the core of the microbubble caused backscattering of ultrasound waves, resulting in enhancement. However, due to high surface tension, saline microbubbles were highly unstable and air in the core got easily dissolved in blood. In the year 1970, Kremkau et al. injected autologous blood at a rapid rate to produce more stable microbubbles, but still the half-life was not adequate. In the year 1990, Feinstein et al. described first commercially available and FDA-approved USCAs by the name of Albunex. CAs used in ultrasound are basically microbubbles enclosed in a shell. They have become popular as they are safe and radiation free. Two principles play a role in contrast-enhanced ultrasound: one is enhancing the echogenicity of the lesion that is imaged and the second is suppression of the background signal. CAs markedly increase the backscatter due to large difference in acoustic impedance at gas fluid/tissue surface interface. The size of microbubbles is in the range of 3–5 μm so they resonate maximally at the general frequency used in ultrasound. Second effect of background suppression is achieved by a technique called pulse inversion. For this, two similar signals with opposite phases, which are mirror images of each other, are sent through the same scan line, and echoes from both are collected and added by the transducer. Normal tissues that act like linear reflectors produce no net signal, whereas the ones having microbubbles act like nonlinear reflectors and produce some net signal which stands out against a dark background. When ultrasound waves strike these molecules (tissues with microbubbles), they strongly backscatter and increase the echoes by a factor of 500–1000, resulting in enhancement. Microvascular flow rate can also be calculated by calculating the rate at which microbubbles are in the imaging plane. The microbubbles have a core, consisting of a gas which is insoluble in blood, surrounded by a shell made up of biocompatible material, either lipid, protein or polymer. The shell thickness is 10–200 nm and may be hard, as in denatured albumin or soft and flexible as in lipids. The core gas may be air, perfluorocarbon or sulphur hexafluoride. Ideally the core gas should be inert having low diffusibility and solubility with high vapour pressure so as to persist in microcirculation for longer time. Air gets easily dissolved in blood; hence, it is not an ideal core gas. The other two show low solubility in blood and do not diffuse through the shell easily, so they are preferred. Air containing microbubbles are classified as first-generation CAs, whereas those containing different gases are second generation. Composition and use of various USCAs are tabulated in Table 1.16.2. Intracardiac shunts (R to L), tubal patency in sonohysterosalpingography Cardiac imaging 200 mg/mL – transcranial Doppler 300/400 mg/mL – abdominal imaging Abdominal imaging Vascular phase imaging of various organs Cardiac applications Hepatic imaging Sonovue is the most popular CA used in today’s era and is the only one available in India. It is composed of sulphur hexafluoride gas in the core surrounded by a phospholipid shell. The size of the microbubble is almost 3 μm, having a half-life of 6 min, and is excreted via lungs in about 11 min. It is very stable in blood with low solubility of gas and hard covering. Sonovue is a pure blood pool agent and is used for vascular phase imaging of various organs. Its size enhances the backscattering even at lower acoustic power insonation. As the size of microbubbles is between 3 and 5 μm (slightly less than that of red blood cells), they are unable to cross the vessel wall and thus are purely intravascular in nature. The duration of enhancement post contrast injection usually is between 2 and 10 min. USCAs remain in blood for about 5 min giving a vascular phase after which they are dissolved in blood, and the gas is excreted via the lungs, whereas the small amount of shell material is metabolized by liver. Alternatively, when run as an infusion through a saline drip, enhancement can be obtained for up to 20 min. Few of the agents such as Levovist and Sonazoid show late hepatic phase as these are taken up by the Kupffer cells or are concentrated in sinusoids of liver and spleen. The USCAs are injected intravenously in a dose of 0.2–2 mL mixed with 5 mL saline. The soluble mixture is prepared in the vial and shaken to form microbubbles. The area of interest is scanned on B mode. Then with the dual mode on, both B scan and contrast-specific mode are focussed on screen. A three-way large bore cannula is used for delivering the bolus of USCAs, followed by saline flush of 10–20 mL. The area of interest is scanned continuously for evaluation of the lesion in all the phases, and cine loops can be archived for repeat viewing. As the microbubbles get destroyed at high mechanical index (MI), scanning at low mechanical index is preferred for prolonging the half-life of the microbubbles. The ultrasound machine should be equipped with low MI contrast imaging mode. These were developed to reduce the artefact and shadowing of bowel which interferes with abdominal scanning. Simethicone-coated cellulose suspension, called SonoRx, is one such agent which reduces the gas artefacts of bowel and improves visualization. However, these are not available in India. In 1984, Weinmann first published a seminal paper on initial study results for gadopentetate dimeglumine, which presented gadolinium as a potential magnetic resonance imaging CA. Subsequently in the year 1988, gadopentetate dimeglumine (Magnevist) became the first MRI CA, which was approved for clinical use. Since then, many gadolinium-based contrast agents (GBCAs) were produced commercially, and till now eight other gadolinium chelates have been developed and approved in many regions worldwide. Almost 50% of the currently performed MR examinations are contrast-enhanced studies, and there has been steady increase in their rate in the past two decades. Many new MRI CAs other than GBCAs have already been investigated and used in clinical practice. MRI CAs can be classified according to the following: MRI CAs can be described as paramagnetic agents and superparamagnetic agents based upon their magnetic properties. Paramagnetic materials have their own magnetic field, which leads to a reduction in the T1 and T2 relaxation times of the surrounding tissue through interaction of their electron spins with spins of protons in the respective tissues, thereby increasing its signal on T1-weighted images. A number of paramagnetic metal ions can be used as potential MRI CAs, but the transition metal gadolinium (Gd3+) is the most commonly used ion. This is due to a favourable combination of seven unpaired electrons with a long electron spin relaxation time, making it a very efficient relaxation-enhancing agent. Manganese, having relaxation properties similar to gadolinium, is another paramagnetic ion used as MRI CA. All paramagnetic CAs need to be chelated with an appropriate ligand to reduce the in vivo toxicity of free metal ions. Superparamagnetic agents also behave similar to paramagnetic substances. Small or ultrasmall particles of superparamagnetic iron oxide are used as CAs in clinical MRI. The net magnetic moment of these agents greatly exceeds that of typical paramagnetic ions when the iron ions are magnetically oriented within the crystal. This characteristic effect of a large magnetic moment in the presence of an external magnetic field without any remnant magnetic moment when the field is zero is called superparamagnetism. Although the dominant effect of these agents is on T2/T2* relaxation, they can also induce a strong enhancement of the T1 relaxation rate of water, depending on size and composition of their particles. CAs can be principally divided into T1 CAs and T2 CAs according to their primary effect on T1 or T2 relaxation times. The CA can either have a positive effect (increase in signal or T1 enhancement) or a negative effect (signal reduction or T2 enhancement) on the signal intensity of tissue. Mostly, all MR agents affect both T1 and T2 relaxation times, and the distinction relies on many MR specific parameters as well as CA dose. T1 agents typically increase the longitudinal (or spin–lattice) relaxation rates (1/T1) of water protons in tissue more than the transverse (or spin–spin) relaxation rates (1/T2), thereby resulting in increased signal intensity on a T1-weighted image (positive CAs). Paramagnetic CAs such as gadolinium-based contrast agents are most commonly used as T1 CAs due to a predominant shortening of the T1 relaxation time. GBCAs in fact reduce both T1 and T2 relaxation times; however, they have a weak T2 negative enhancing effect. T2 agents largely increase the transverse (or spin–spin) relaxation rates (1/T2) of tissue rather selectively, i.e. they have much greater T2 relaxivity than T1 relaxivity. These agents result in decreases in signal intensity, in areas of uptake/distribution on T2-weighted images, thus representing negative CAs. Superparamagnetic CAs such as iron oxide particles act as T2 CAs, as they have a substantially higher magnetic moment than paramagnetic CAs, with magnetic susceptibility effects dominating. Ferumoxides (Feridex, Endorem) and ferucarbotran (Resovist) are two such agents primarily used for liver imaging and are still available in some countries. Depending upon their biodistribution patterns, the MRI CAs can be classified into extracellular agents, blood pool agents (intravascular) and tissue- or organ-specific agents. Small molecular weight paramagnetic agents, also known as extracellular fluid (ECF) agents, are typically distributed to the extracellular fluid, as they can easily diffuse from the plasma into the interstitium. Most of the GBCAs are included in this category. They are not taken up by cells and are therefore eliminated by renal excretion. Due to their high water solubility, around 98% of particles get eliminated from the body within 24 h. Intravascular agents also known as blood pool contrast agents (BPCAs) have a molecular weight large enough to prevent leakage from the vascular to the extravascular space. These agents remain in the bloodstream for longer duration and possess much longer vascular half-life than the conventional extracellular agents. This results in a broader window, thus generating an excellent enhancement of the vascular space in clinical setting. They are slowly excreted by the kidneys and liver. All iron oxide nanoparticles are intravascular agents. In blood, iron oxide agents with the appropriate composition can produce significant T1 shortening, and several studies have reported the use of nanoparticles for MR angiography. Macromolecular gadolinium compounds are another type of intravascular agents. They have been designed either by linking Gd3+ ions to a macromolecular polymer during synthesis or by making the Gd3+ complex bind to plasma proteins (albumin) after injection, thus forming macromolecules in blood (e.g. Gadofosveset). Tissue-specific agents typically accumulate in a particular organ or tissue type. Intravascular iron oxide nanoparticles are reticuloendothelial organ-specific agents, which are taken up by the Kupffer cells present in the liver, spleen and lymphatic system. In the cells, the nanoparticles are accumulated into larger particulate clusters, and tissue relaxation is then completely dominated by T2/T2* effects. Iron oxides are, thus, both intravascular agents and tissue-specific agents. Mangafodipir trisodium and few gadolinium chelates also act as liver-specific agents as they are taken up by hepatocytes. The GBCAs are the most widely used MRI contrast agents in the routine clinical practice. Gadolinium (Gd) is a rare earth metal of the lanthanide series with strong paramagnetic properties, which are important for MRI enhancement. The unpaired electrons present in Gd attract the nearby protons such as in water. Gd reduces the relaxation time of adjacent protons in the tissue in a magnetic field at relatively low concentrations and thus provides enhanced image quality, which is important for tissue contrast differentiation and disease diagnosis. As the ionized gadolinium carries significant risk of toxicity, GBCAs have been formulated by chelating with various organic ligands to avoid the effects of free gadolinium ions. GBCAs are divided into linear and macrocyclic categories based on the shape of the organic ligand or chelate introduced to detoxify Gd (Fig. 1.16.2). To date, nine GBCAs have been approved by the US Food and Drug Administration (FDA) for MRI use. Commercially available GBCAs are tabulated in Table 1.16.3.
1.16: Contrast media
Iodinated contrast agents
Development
Classification
Group
Ions per Molecule
Iodine Atoms per Molecule
Ratio
Osmolality (mOsm/kg)
Examples
Ionic monomer (HOCM)
2
3
1.5
>1400
Diatrizoate (Urografin), iothalamate (Conray), metrizoate (Isopaque), iodamide
Ionic dimer (LOCM)
2
6
3
∼600
Ioxaglate
NonIonic monomer (LOCM)
1
3
3
500–700
Metrizamide (Amipaque), iohexol (Omnipaque), iopamidol, iopromide (Ultravist), etc.
NonIonic dimer (IOCM)
1
6
6
300
Iotrol, iodixanol (Visipaque)
Pharmacokinetics
Intrathecal use of iodinated contrast
Cholangiographic agents
Oil-based iodinated contrast agents
Gastrointestinal contrast agents
Barium sulphate solution
Preparations
Paste.
Suspension.
High density (HD).
Low density.
Sweetening agent.
Colouring agent.
Antifoaming agents.
Preservatives.
Carboxymethylcellulose.
Side effects and contraindications
Enteric contrast agents for computed tomography examinations
Positive oral CAs
Neutral CAs
Negative CAs
Ultrasound contrast agents
Development
Basic principles
Composition
USCA
Core
Shell
Use
Remarks
FIRST GENERATION
Echovist
Air
Galactose
Albunex
Air
Albumin
Levovist
Air
Galactose + palmitic acid
Hepatosplenic parenchymal phase
Sonavist
Air
N-butyl 2 cyanoacrylate
Hepatic phase
SECOND GENERATION
Sonovue
Sulphur hexafluoride gas
Phospholipid
Only one available in India
Optison
Perfluorocarbon
Albumin
Sonazoid
Perflubutane
Lipids
Prolonged hepatic phase
Pharmacokinetics
Technique
Oral ultrasound contrast agents
Magnetic resonance imaging contrast agents
Development
Classification
Magnetic behaviour
Paramagnetic CAs.
Superparamagnetic CAs.
Image enhancement
T1 agents.
T2 agents.
Biodistribution
Extracellular fluid agents.
Intravascular agents.
Tissue-specific agents.
Gadolinium-based contrast agents
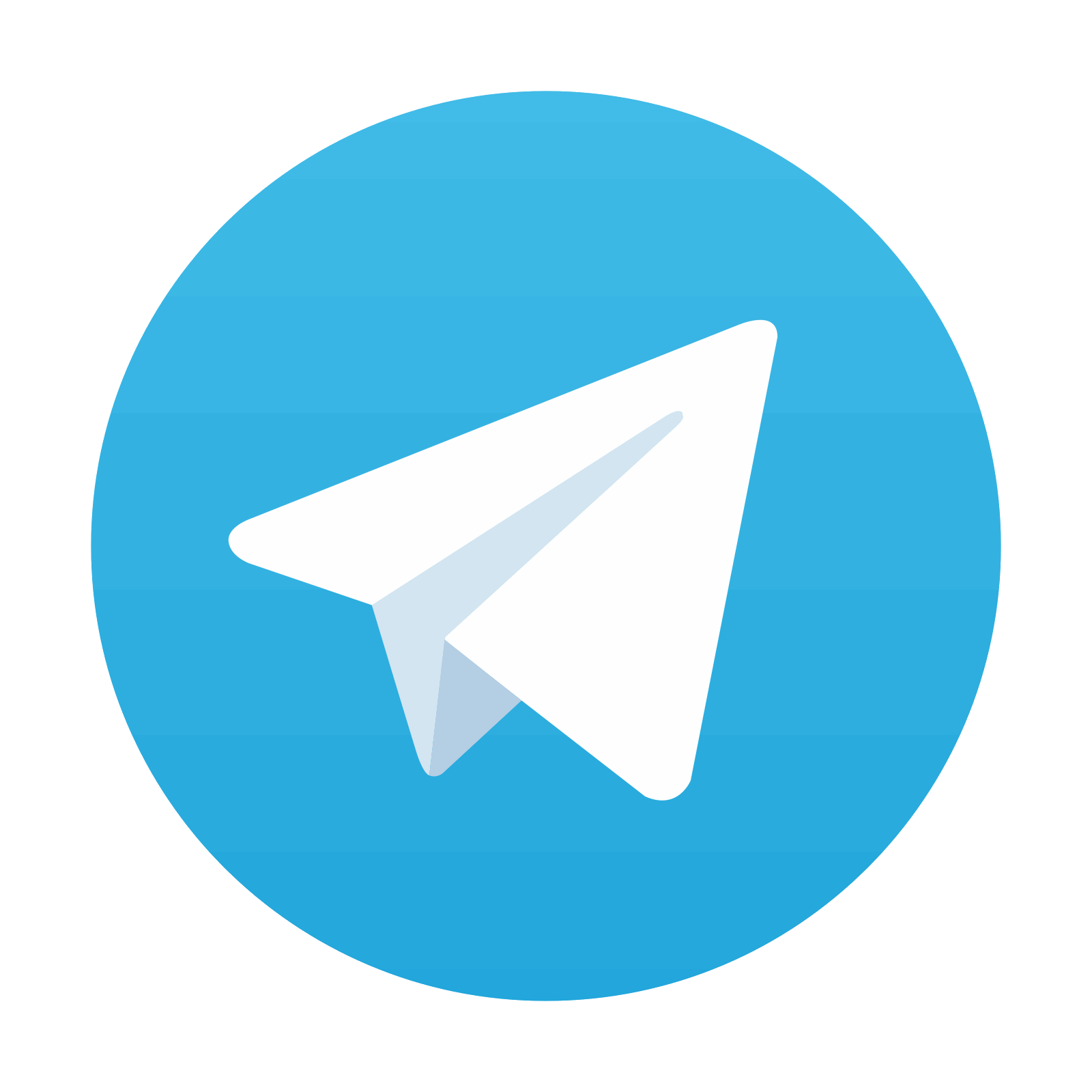
Stay updated, free articles. Join our Telegram channel

Full access? Get Clinical Tree
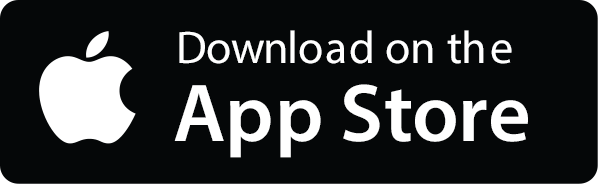
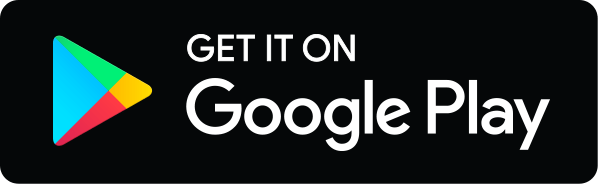