Fig. 9.1
Meta-analysis of randomized trials of percutaneous transluminal coronary angioplasty versus atherectomy, cutting balloon atherotomy, or laser angioplasty. (a) Mortality by treatment assignment up to 30 days. (b) Major adverse cardiac events up to 30 days. (c) Major adverse cardiac event rates up to 360 days. CBA coronary balloon atherotomy, CI confidence interval, DCA directional coronary atherectomy, LA (excimer or holmium) laser angioplasty, OR odds ratio, PTRA percutaneous transluminal rotational atherectomy, PTCA percutaneous transluminal coronary angioplasty (*The ERBAC control groups are identical) (From Bittl et al. [1], with permission.)
Heavily calcified, dilation-resistant fibrotic coronary lesions continue to represent a significant challenge for the coronary interventionalist. These lesions typically reside in vessels with advanced disease sometimes in association with proximal vessel curvature or scattered moderate fibrocalcific disease. Balloon dilation-resistant lesions may also occur unexpectedly in relatively focal disease but with significant superficial calcification. These situations provide technical challenges of two types: dilating severe lesions and delivering stents to those lesions. These lesions typically dilate poorly even with modern noncompliant high-pressure balloons and dissections and perforations may occur. Even if the lesions yield at high pressure, stent delivery may be problematic and refractory to advanced delivery techniques including supportive guides, “buddy” wires, and anchoring methods. Inadequate results occur if a stent is unable to be delivered or well expanded with increased risks of stent thrombosis (Fig. 9.2). Importantly, the excessive vessel manipulation required to treat these lesions may result in vessel dissection and occlusion with poor bailout options. If a target vessel is clearly heavily calcified by either fluoroscopy or IVUS or if there is difficulty advancing a balloon to or through the target lesion or balloon expansion is poor, then it is reasonable to assume that the standard balloon and stent approach will fail.
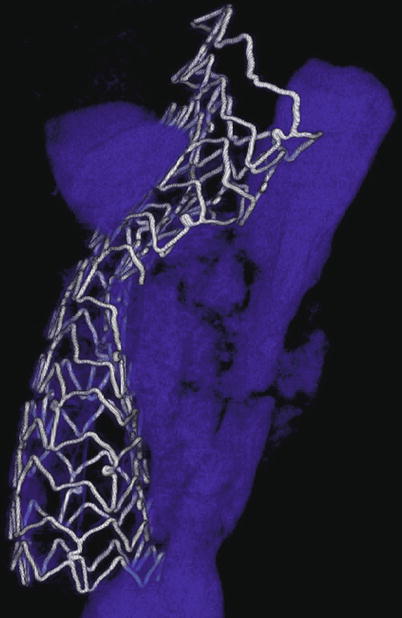
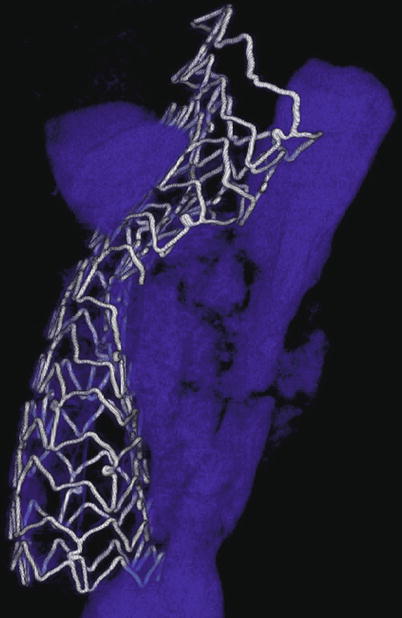
Fig. 9.2
MicroCT of incomplete stent expansion. A 72-year-old man presented with an anterior STEMI and underwent primary PCI of a calcified, proximal LAD lesion but ultimately died of cardiogenic shock. Postmortem microcomputed tomography image of the proximal LAD stent revealed poor stent expansion despite high-pressure balloon inflations with a noncompliant balloon (calcium shown in blue)
Rotational Atherectomy
Rotational atherectomy is currently a reliable, well-tested technique to achieve successful and durable percutaneous revascularization in these circumstances. This method bears little resemblance to the standard techniques commonly used by the coronary interventionalist and thus a different skill set needs to be acquired. Common experience has shown that rotational atherectomy needs to be performed early in the procedure prior to causing significant vessel dissection, which may limit the advisability of rotational atherectomy.
The only available high-speed rotational atherectomy system approved for coronary vessels is the Rotablator (Boston Scientific, Natick, MA) approved by the FDA in 1993. This device employs a diamond chip-coated brass burr able to rotate at high speeds (Fig. 9.3). The burr is attached to a drive shaft able to accommodate a steerable 0.009-in. stainless steel guidewire over which the device is advanced. The distal portion of the burr is embedded with diamond crystals having 5 μm extruding from a nickel coating. The burr has a smooth proximal surface not coated with diamond crystals. The driveshaft is covered by a 4.3F (0.058 in.) Teflon sheath that protects tissue from the spinning driveshaft and allows flush solutions to lubricate the driveshaft and burr and provides vasodilators during use. Typical flush solutions (rotaflush) contain 20 cm3 of Rotaglide Lubricant (emulsion of olive oil and egg yolks), 4 mg of nitroglycerin, 5,000–10,000 units of heparin, and some add 4 mg of verapamil per liter of normal saline. The RotaWire guidewire is a specialized product 330 cm long constructed of stainless steel. Having a 0.014-in. diameter 2.2 cm spring tip, the wire then transitions from 0.005 in. diameter to 0.009 in. on its proximal end. The RotaWire Floppy is used in nearly all cases due to its ability to follow vessel contours with reduced guidewire bias compared to the RotaWire Extra Support (Fig. 9.4). This reduces the possibility of ablation of healthy tissue due to a more asymmetric course taken by the stiffer, extra support wire resulting in greater engagement of the lumen wall by the burr. The stiffer wire was designed to reach distal lesions. The Rotalink burr catheter is available in 1.25–2.5 mm sizes. The Rotalink system allows the burr size to be changed without replacing the entire drive unit. The catheter’s driveshaft is connected to a turbine driven by compressed nitrogen. The burr is activated by a foot pedal, and the rotational speed is measured by a fiber optic cable and controlled by a dial on the device’s monitor.
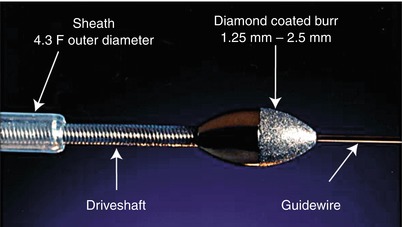
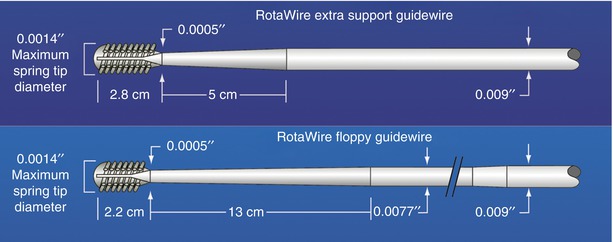
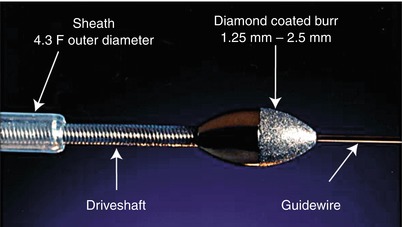
Fig. 9.3
Rotalink burr catheter (Courtesy of Boston Scientific)
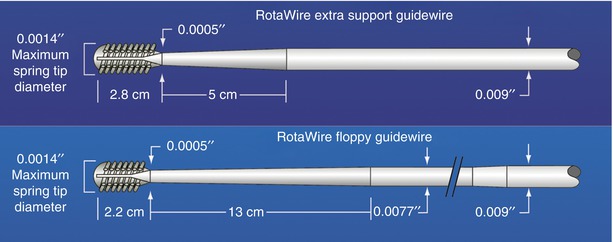
Fig. 9.4
RotaWire specifications
Mechanism
Rotational atherectomy works by mechanical plaque abrasion and pulverization applying the principle of differential cutting and orthogonal displacement of friction. Orthogonal displacement of friction allows the spinning burr to negotiate tortuous segments allowing access to more distal lesions that might impede a stent or even at times a balloon-dilating catheter. Differential cutting spares normal elastic tissue while destroying inelastic fibrocalcific plaques. Inelastic calcified elements are pulverized by the advancing burr while elastic elements are deflected and spared. IVUS studies have shown that fibrocalcific material is removed without arterial expansion [7–9]. In addition, angiographic follow-up studies have shown that adjacent segments are not adversely affected and do not show accelerated atherosclerosis (Fig. 9.5). Plaque pulverization ideally produces particulate debris small enough to pass through the myocardial capillary bed. Some studies have shown that uncomplicated rotational atherectomy can be performed without significant impact on myocardial perfusion. Rotational atherectomy and adjunctive PTCA significantly improved basal and hyperemic blood flow velocity and diastolic predominance of coronary blood flow with some improvement in coronary flow reserve [10]. However, the physiologic effects of rotational atherectomy can result in slow or no reflow in approximately 7–10 % of patients with associated myocardial injury and infarction including persistent ST elevation, CK release, and new Q waves in even higher percentages of treated patients. The mechanism for this is likely multifactorial and includes plaque debris, platelet aggregation, microcavitation, and vascular spasm, with capillary plugging and tissue edema. Using nuclear imaging, Koch et al. found that 87 % of patients having rotational atherectomy had transient perfusion defects which was decreased to 33 % in patients who received abciximab during the procedure [11–13].
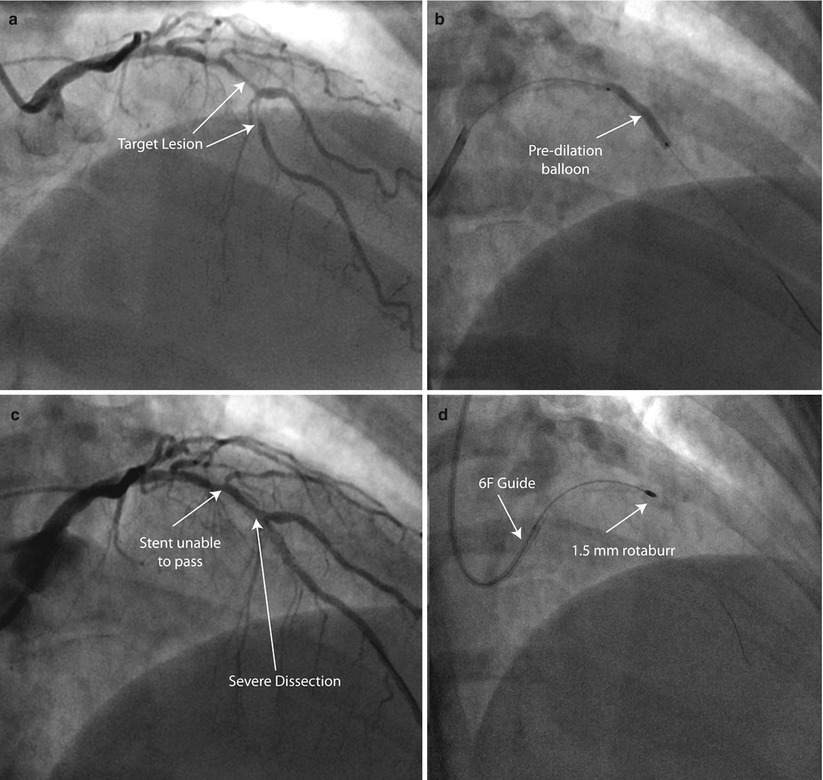
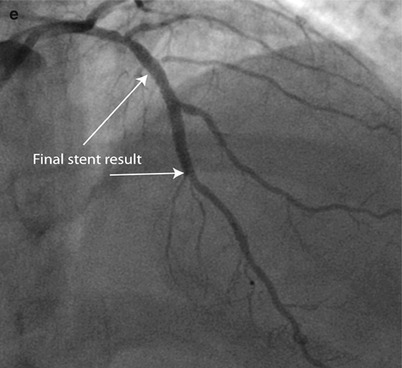
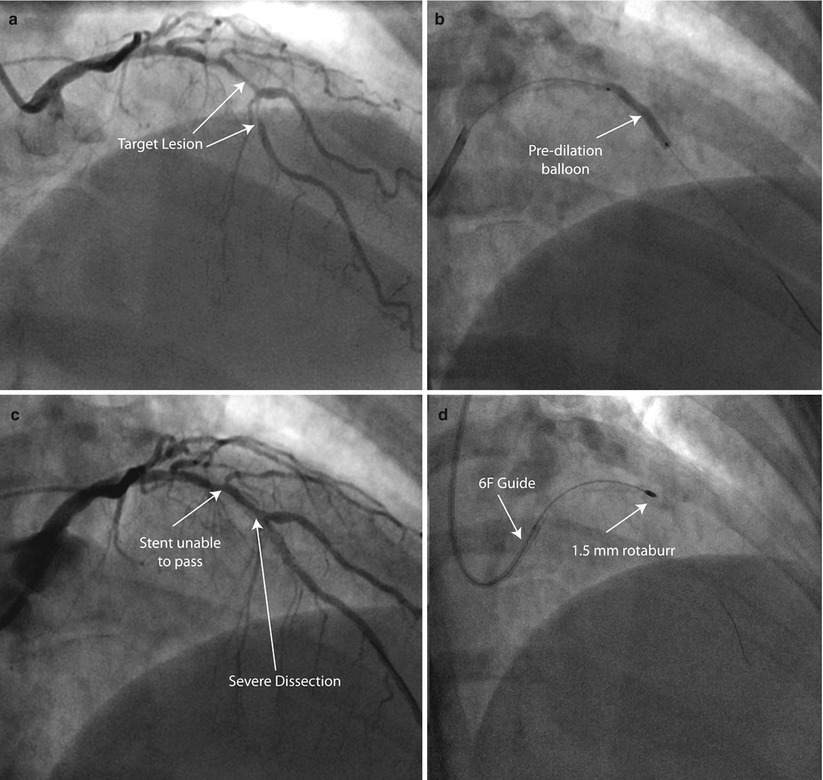
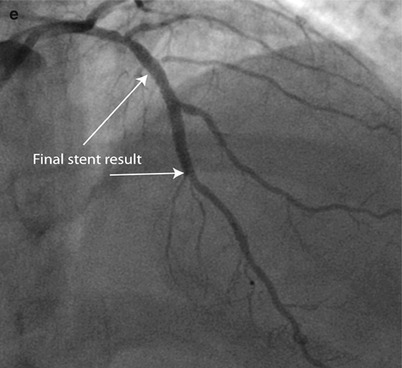
Fig. 9.5
Rota rescue case. (a) A 61-year-old woman presented with unstable angina and (b) developed severe dissection following pre-dilation of the mid LAD lesion. (c) Stents were unable to pass through an undilatable proximal segment until (d) rotational atherectomy was performed (e) allowing successful stent delivery
Procedure
Rotational atherectomy is initiated with conventional angioplasty equipment. A 6F guide is able to accommodate the 1.25 and 1.5 mm burrs. Larger burrs up to 2.15 mm require an 8F guide (Table 9.1). A guide that is coaxial with the vessel and sits easily in the ostium should be chosen. Patients should be well hydrated and not hypotensive prior to initiating rotablation. If the target lesion is in the right coronary artery or dominant circumflex, then a temporary pacing wire should be placed in the right ventricle as significant transient bradycardia is unpredictable and common. Many operators use IIb/IIIa agents which have been shown to reduce burr-induced platelet activation and CK release [12, 14–16]. The presence of a IIb/IIIa agent substantially worsens the prognosis of perforation should that occur. The rotablator guidewire does not steer well and is prone to kinking. Although some operators favor steering this wire directly past the target lesion, it is generally best delivered distal to the target lesion via a transport catheter previously advanced over a “workhorse” 0.014-in. guidewire. The rotablator guidewire is placed distal to the lesion but not in the distal narrow vasculature. This allows the burr to remain centered when activated. The activated burr should not come into contact with the 0.014-in. spring tip. The rotablator guidewire is then back loaded through the rotalink burr catheter and brought through the rotalink advancer unit. A specialized clip torquer is then attached to keep the wire from spinning when the burr is activated. The device’s compressed gas connector, fiber optic cable, and pressurized rotaflush saline solution infusion port are connected, and the burr is advanced over the wire to within a few cm of the guide catheter. Flowing pressurized saline solution is essential to cool and lubricate the spinning parts of the system. With the pressurized saline solution running, the system is activated and tested prior to advancing the burr into the guide catheter. The burr speed is adjusted to 150,000–160,000 rpm; saline flush solution is ensured and the advancer knob and wire brake are tested. Prior to inserting the burr into the guide catheter, the advancer knob is moved forward approximately 2–3 cm and locked in position. With the guide catheter well seated in the vessel ostium, the static burr is then advanced over the wire to a widely patent position in the target vessel at least 1–2 cm proximal to the lesion. The advancer knob is then retracted fully to relieve any tension on the driveshaft. This can be verified fluoroscopically by watching the burr retract slightly. Relieving drive shaft tension keeps the burr from jumping forward into the stenosis when the system is activated. The burr’s rotational speed in the free lumen is checked again and adjusted prior to negotiating the lesion (platforming). The burr is then activated and gently advanced into the lesion with a tapping motion by advancing the controlling knob under fluoroscopic guidance. Intermittently pulling back on the advancer knob will allow improved blood flow during the rotablator run. Care should be taken not to allow decelerations of greater than 5,000 RPM to occur as this is associated with slow or no reflow and myocardial injury. The operator listens to the sound of the turbine, is aware of the RPM display on the monitor, and advances and withdraws the burr under fluoroscopic guidance deciding how firmly the burr can be safely advanced. After a 30-s run, the burr is then promptly withdrawn more proximally in the vessel free of the lesion and immediately deactivated. The patient is assessed both hemodynamically and with a small contrast injection to verify preserved flow and no perforation and to allow the patient time to recover. Small doses of intravenous phenylephrine may be required to treat transient hypotension. In addition, intracoronary calcium blockers such as nicardipine are commonly administered to treat abnormal flow. Once the hemodynamics and coronary flow have recovered, this sequence is repeated. The goal is to be able to pass the burr with minimal resistance and no decrease in the RPM measurement through the length of the lesion. Once this is accomplished, the foot pedal is used to switch to the dynaglide mode, the brake is defeated either manually or with the aid of the wire clip torquer, and the burr is removed maintaining distal wire position. A transport catheter is used to exchange the rotawire for a “workhorse” 0.014-in. wire, and adjunctive balloon angioplasty is typically performed prior to stent placement. Most operators no longer use a stepped burr approach and use only a single burr to facilitate lesion modification and PTCA/stent placement with a burr/artery of 0.5–0.6. In the unusual event the initial burr will not pass at 160,000 RPM, success may be achieved by downsizing to a 1.25 mm burr.
Table 9.1
Rotalink burr catheter and guide selection
Burr (mm) | Diameter (in.) | Recommended guide catheter (French)a | Minimum recommended guide catheter internal diameter (in.) |
---|---|---|---|
1.25 | 0.049 | 6.0 | 0.060b |
1.50 | 0.059 | 6.0 | 0.063 |
1.75 | 0.069 | 7.0 | 0.073 |
2.00 | 0.079 | 8.0 | 0.083 |
2.15 | 0.085 | 8.0 | 0.089 |
2.25 | 0.089 | 9.0 | 0.093 |
2.38 | 0.094 | 9.0 | 0.098 |
2.50 | 0.098 | 9.0 | 0.102 |
Early Studies
Rotablator was approved and used before modern stents were in widespread use. Much of the early experience is of historical significance, as rotational atherectomy is no longer performed without stent placement. The early trials defined acute outcomes and complications, restenosis rates, target vessel revascularization rates, and compared these parameters to other devices available at the time [1]. Early experience with rotational atherectomy was difficult with significant complication rates and no clear advantage over other modalities. Vessel perforation did occur. Adjunctive balloon angioplasty was essential to improve acute success rate [17, 18]. The angiographic restenosis rate ranged from 39 to 62 % which has been eclipsed by drug-eluting stents [19, 20]. The Stratas and Carat trials found no advantage to aggressive debulking with larger burrs (>0.7 burr/artery) and longer ablation times compared to more conservative debulking strategies [21, 22]. Aggressive debulking was associated with more serious angiographic complications and higher angiographic restenosis and target lesion revascularization. The incidence of reduced flow and myocardial injury resulting from rotational atherectomy is technique dependent [21–24]. Kini reported improvement in the incidence of slow flow from 9 to 2 % [24]. With optimized technique the incidence of slow flow and non-Q wave MI can be reduced to a few percent [25]. Several risk factors for the myocardial injury have been identified. These include long burr runs (greater than 30 s), decelerations (greater than 5,000 RPM for 5 s), aggressive burr sizing, and long lesions with presumed greater burden of pulverized debris.
Perforation
The incidence of coronary perforation with rotational atherectomy is 0.7–1 % in highly calcified coronary disease [5, 24, 26]. Al-Lamee et al. reported a 0.23 % incidence of grade III coronary perforations in a study of 24,445 patients undergoing interventions from 1993 to 2009 [27, 28]. Multivariate regression showed the odds ratio for grade III perforation was 5.36 for IVUS-guided procedures, 3.75 for type B2/C lesions, 3.47 if rotational atherectomy was performed, and 1.91 for total occlusions. A similar rate of grade III perforations (0.2 %) was found in another large cohort when no rotablation was performed [29]. Other large series reporting the incidence of coronary perforation list rotational atherectomy as a cause; however, perforation is also found with balloon angioplasty, stent placement, or due to manipulation of hydrophilic guidewires [30, 31]. While the incidence of perforation is higher with rotational atherectomy compared to balloon angioplasty, the high pressures required to expand these calcific lesions is also associated with an increased risk of balloon-induced vessel perforation. If perforation does occur, the presence of IIb/IIIa inhibitors is clearly an issue as more patients require placement of a covered stent or emergency surgery [15]. For this reason, some operators avoid IIb/IIIa inhibitors with rotational atherectomy.
Special Lesion Sets for Rotablator Use
Other than its use to facilitate stent delivery, rotablator has been reported to be useful in treating other lesion sets. Jailed side branches in previously stented vessels may be treated once the stent cell overlying the branch has been successfully balloon dilated but with a significant restenosis rate [32]. Calcified ostial RCA lesions are well treated with rotational atherectomy with durable results [33]. Ostial saphenous vein graft lesions may be treated with rotational atherectomy as well as lesions in internal mammary grafts [34, 35]. There is increasing use of rotational atherectomy in treatment of chronic total occlusion. While this is an evolving application, there is increasing experience and success within the lesion segment and even the subintimal space.
Rotational atherectomy should be avoided in lesions found in the body of saphenous vein grafts, situations with thrombus that cannot be removed, and in the acute MI setting because of the risk of distal embolization from thrombus or soft particulate matter. It is tempting to consider rotational atherectomy for diffuse in-stent restenosis as it does remove intimal hyperplasia compared to balloon dilation which works via tissue extrusion and further stent expansion. Compared to balloon angioplasty in the Artist trial, however, there was no significant benefit to rotational atherectomy for this indication [36]. Rotablator should be avoided in low ejection fraction situations or last vessel situations in which slow or no reflow would be catastrophic. Data from the Protect II trial showed less clinical benefit for patients undergoing high-risk percutaneous revascularization when rotational atherectomy was used in the group with Impella support. This may be related to the more vigorous use of rotational atherectomy in the impella 2.5 arm [37]. Other situations to avoid include eccentric lesions on a severe bend where wire bias is significant and the burr would be deflected away from the lesion toward normal vessel wall. Stand-alone rotablator or rotablator followed by adjunctive balloon angioplasty is not recommended due to unacceptably high restenosis rates.
Rotational Atherectomy in the Drug-Eluting Stent Era
Today, rotablator is used as an adjunct to balloon angioplasty to facilitate successful stent placement (Fig. 9.5). Intravascular ultrasound can be used to define which lesions will require rotablator to allow adequate stent expansion [9, 38, 39]. Superficial calcium deposits of over 180° are best treated with rotational atherectomy prior to stent placement. If vessels appear heavily calcified or if there is significant difficulty passing a balloon to or through a lesion or if a lesion will not yield at moderate pressures, rotational atherectomy is likely to facilitate successful revascularization. In the drug-eluting stent era, best practice parameters have established an excellent safety and outcome profile for rotational atherectomy in heavily calcified arteries [4, 5]. When used favoring a single conservatively sized burr approach followed by adjunctive balloon angioplasty and stent placement, Clavijo et al. found that lesions requiring rotational atherectomy prior to drug-eluting stent placement had similar acute and chronic outcomes to drug-eluting stent placement that did not require rotablator for successful implantation [4]. The primary success rate was 98 % with a low rate of in-hospital ischemic events. The target vessel revascularization rate was less than 5 % in both groups. Rotational atherectomy may be performed without harm in these circumstances as was found in a large multicenter registry of 1,537 stented patients with moderate to severe calcification in whom 7.5 % received rotational atherectomy prior to stent placement [40]. There was no difference in the efficacy and safety outcomes regardless of the use of rotational atherectomy in this study. Rathore et al. found that in a series of patients with heavily calcified coronary disease, rotational atherectomy followed by balloon angioplasty and stenting provided excellent primary success rates (97 %) using a single burr strategy 81 % of the time. In addition, the use of drug-eluting stents resulted in a 50 % reduction in angiographic binary restenosis compared to bare metal stents after rotational atherectomy (11 % vs. 28 %) [5]. Independent predictors of restenosis after rotational atherectomy were the use of bare metal stents, ostial location, chronic total occlusion, and dialysis-dependent chronic renal failure. But, lesion length, minimal lumen diameter, and final lumen area and diameter failed as independent predictors. Restenosis rates with bare metal stents after rotational atherectomy have been reported to be 22–28 % range [5, 41]. Although data are limited, available data favors drug-eluting stents over bare metal stents after rotational atherectomy with significantly less restenosis. The ROTAXUS study randomized 240 patients with complex calcified coronary lesions to either routine rotablation or balloon angioplasty prior to paclitaxel-eluting stent placement. A higher initial success rate was found in the routine rotablation group (92.5 % versus 83.3 %) with a crossover rate of 12.5 % in the balloon angioplasty arm. Routine rotational atherectomy before pacitaxel-eluting stent placement, however, was not superior to balloon agioplasty before stent placement with no difference in 9 month endpoints of binary restenosis (11 %), target lesion revascularization, stent thrombosis or MACE. Ballon angioplasty with only provisional rotational atherectomy is a reasonable default strategy for drug-eluting stent placement in complex calcified coronary lesions [42].
Laser Atherectomy
Excimer laser (XeCl) angioplasty catheters are available and are approved for use in the coronary vasculature (Spectranetics Corporation, Colorado Springs, Colorado) for the treatment of chronic total occlusions that are crossable with a guidewire, saphenous vein graft lesions, ostial lesions, long lesions, moderately calcified lesions, in-stent restenosis prior to the administration of intravascular brachytherapy, and balloon angioplasty dilatation failures. This excimer laser system is a pulsed-wave device operating at a wavelength of 308 nm in the ultraviolet spectrum and delivering pulses of 125 ns duration at a repetitive rate of up to 40 Hz. Laser energy is transmitted as a standing wave of intense monochromatic, coherent light which travels down optical fibers in the laser catheter appropriately positioned in a coronary artery or saphenous vein graft. The biologic effects of laser light depend on the tissue and its surrounding medium’s ability to absorb the laser’s wavelength and the mode of laser operation (pulsed or continuous) including the energy density (fluence). Radiation in the ultraviolet spectrum is absorbed by the atherosclerotic plaque and also has significant interaction with water. Pulsed systems were designed to reduce heating of surrounding tissue. Rather than photochemical effects, which provide enough energy to break molecular bonds with direct breakdown of molecules potentially providing vascular sparing, the excimer laser energy works predominantly through photothermal and photoacoustic effects [43–45]. Each excimer laser pulse generates a rapidly expanding and imploding vapor bubble. Powerful acoustic waves result which can cause tissue injury and dissection.
Excimer laser angioplasty can be performed with conventional guiding catheters. Coaxial guiding catheter alignment is important for successful device delivery. A catheter with a diameter at least 1 mm smaller than the reference vessel diameter is chosen. The Spectranetics Rx excimer laser catheters are available in sizes from 0.9 to 2.0 mm diameter and are 0.014 in. guidewire compatible. The 0.9 mm Rx and OTW catheters (Spectranetics Corporation) are 6F guide compatible and are used to treat lesions that cannot be crossed with a balloon-dilating catheter as long as local dissection has not occurred. With the laser catheter placed at the proximal end of the target lesion, the guide catheter and vessel are flushed with at least 30 ml of saline to eliminate the interaction of laser energy with contrast medium which may result in vessel dissection. Very slow catheter advancement is important. Once the process is complete, adjunctive balloon angioplasty and stenting are performed.
The initial expectation was that laser angioplasty would provide improved outcomes compared to balloon angioplasty. This was not the case based on published data and trials from the 1990s when the perforation rates were 1–2 % [1, 20, 46–48]. In addition, the experience with excimer laser angioplasty for calcified lesions was disappointing in comparison to rotational atherectomy in the ERBAC trial [20], and rotational atherectomy is preferred for advanced calcification. Improvements in the catheter design and technique with slow advancement and saline infusions may have reduced the risk of dissection and perforations [49–51]. The currently available excimer laser device is used to debulk plaque prior to stent placement in niche applications at experienced centers.
Directional Coronary Atherectomy
The only available device to perform directional coronary atherectomy (FlexiCut catheter, Abbott Vascular, Chicago, IL) has been withdrawn without plans for replacement, and thus this review of DCA is from an historical perspective. This original device (AtheroCath) consisted of a metallic housing with a balloon fixed to one side and a nosecone chamber to collect the cuttings provided by a cup-shaped cutter attached to a drive shaft activated by a battery-operated drive unit. The device could be rotated and oriented by the interventionalist to achieve debulking via directional atheroma excision. Some of the lumen gain achieved by the device was attributed to an angioplasty mechanism related to vessel dilation during atheroma excision. The device received its original FDA approval in 1990 and was quite bulky requiring large guiding catheters (10F) with specialized shapes and smooth curves. The AtheroCath was revised, and five generations of improvements were made to the design. The FlexiCut was 8F compatible but gave up some of the performance benefits of the larger catheters. Multiple trials comparing directional coronary atherectomy to balloon angioplasty with and without coronary stenting were performed [52–58]. These trials generated significant controversy in the interventional community about the relative merits of two techniques. After further analysis, routine directional coronary atherectomy was not found to have significant benefit over balloon angioplasty [1]. Acute complications were higher with directional coronary atherectomy including a 1 % risk of perforation. Due to its more technically demanding requirements, many interventionalists did not gain sufficient experience with DCA. In addition, once the benefits of routine stent placement were defined, use of DCA decreased.
Thrombus Extraction
Acute coronary syndromes develop as a result of plaque rupture or erosion with a common pathophysiologic pathway of thrombosis within the coronary artery [59]. The presence of intracoronary thrombus is associated with an increased risk of adverse outcomes after coronary angioplasty [60]. Furthermore, ballooning and stenting these thrombotic lesions can result in embolization of atherothrombotic debris. Significant residual thrombus at the time of stent deployment may result in incomplete late stent apposition and possible increased incidence of stent thrombosis (Fig. 9.6).
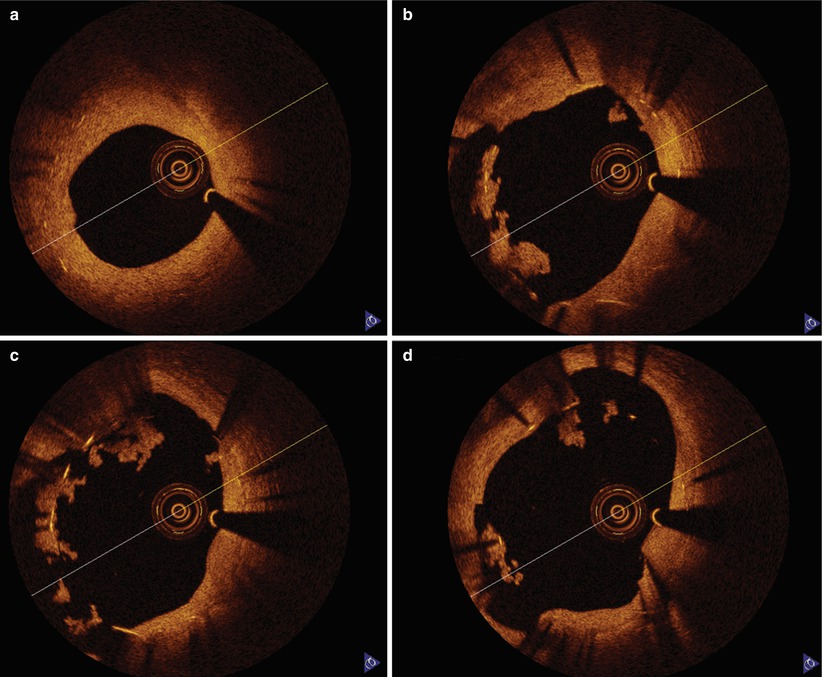
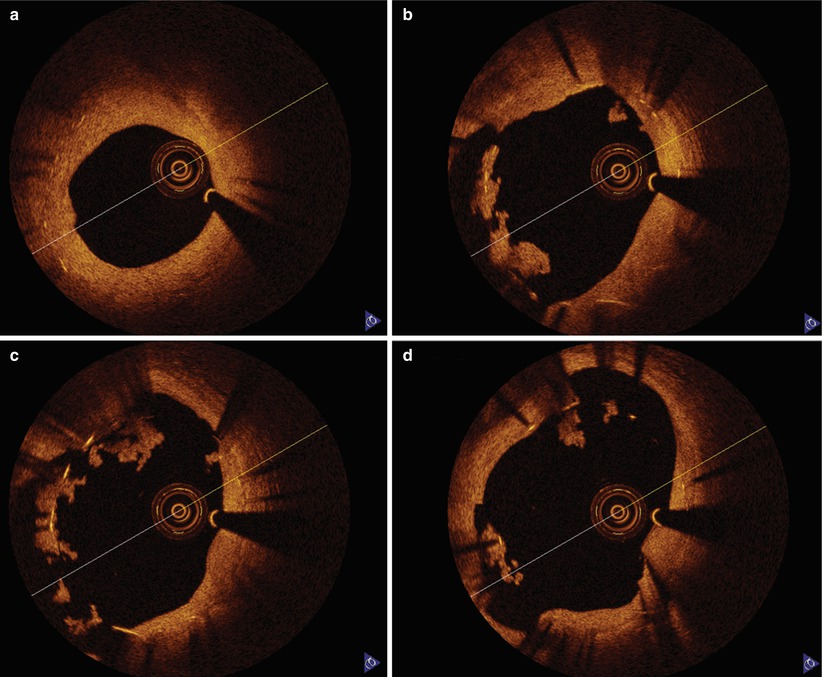
Fig. 9.6
Late stent thrombosis OCT image. A 67-year-old man with bare metal stent implanted in his proximal RCA 6 months prior developed chest pain 5 days after discontinuation of clopidogrel in anticipation of vascular surgery. OCT images demonstrate the bare metal stent with intimal hyperplasia distally (a) and poorly apposed struts with adherent thrombus as the imaging catheter is withdrawn proximally into an area of ectasia (b–d)
Silvain et al. used electron microscopy to analyze the contents of serial manual aspirations from ST elevation MI patients and better defined thrombus composition [61]. Platelet, fibrin, and red blood cell components were found in variable concentrations depending on the ischemic time frame. As the ischemic time increased, the thrombus was primarily a fibrin mesh; however, earlier presentations had a much more platelet dense thrombus (Fig. 9.7).
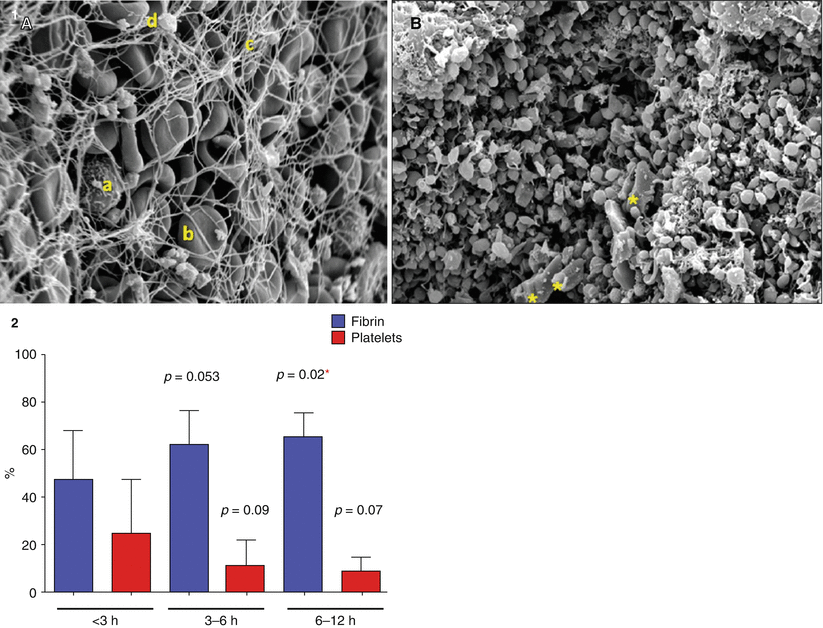
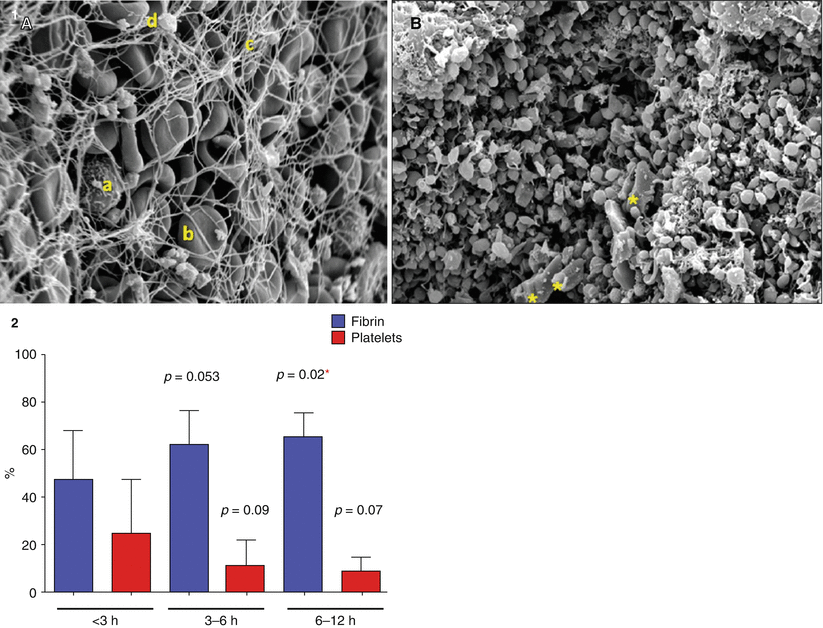
Fig. 9.7
Thrombectomy aspirate composition by ischemic time frame. (1) Scanning Electron Micrograph at 3,000× Magnification. (A) This portion is mainly composed of erythrocytes (b) trapped in a fibrin mesh (c) with few platelet aggregates (d) and rare white cells (a). (B) This portion is mainly composed of platelet aggregates with a few cholesterol crystals (yellow asterisks) (Adapted from Silvain et al. [61], with permission.) (2) Description of the impact of time on thrombus composition demonstrating that the earlier the ischemic time frame, the more platelet rich the extracted thrombus while longer ischemic time frames are associated with more fibrin rich thrombus. The p values are given for comparison with the group <3 h as a reference with multiple Student t test. *p < 0.05. Multiple comparisons were done with the Dunnett’s test, resulting in a p = 0.044 for fibrin and nonsignificant for platelet content
Despite primary PCI, only 35 % of ST elevation MI patients have optimal myocardial reperfusion based on TIMI 3 flow, myocardial blush grades 2 or 3, and greater than 70 % ST segment elevation resolution [62]. The lack of reperfusion of the microvasculature despite appropriate treatment and reperfusion of the epicardial artery is termed slow or no reflow. This phenomenon has a negative impact on short- and long-term outcomes including higher levels of creatinine kinase release, worsened left ventricular function, and worsened 6-month mortality (12.5 % vs. 4.3 % of patients undergoing primary PCI without no reflow) [63
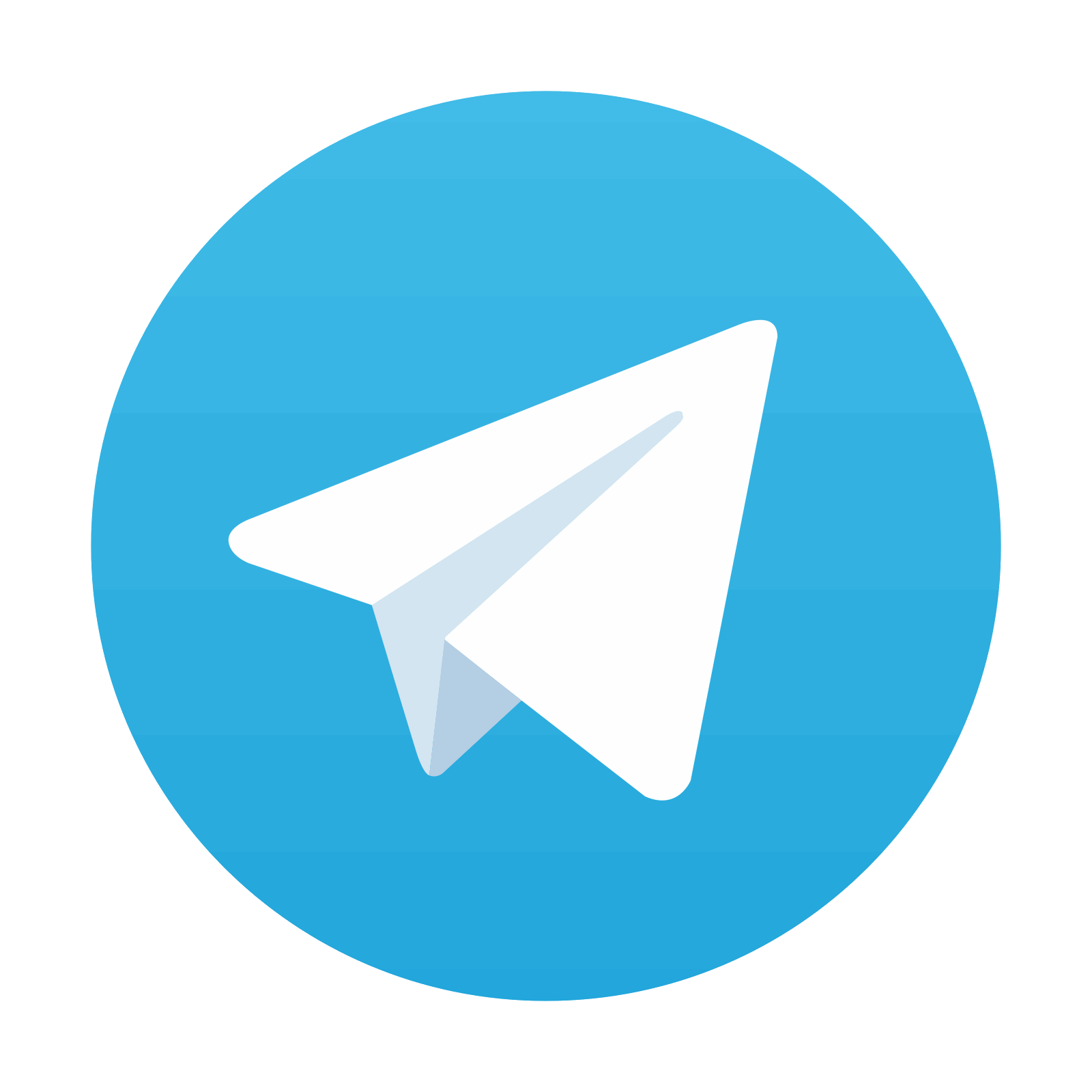
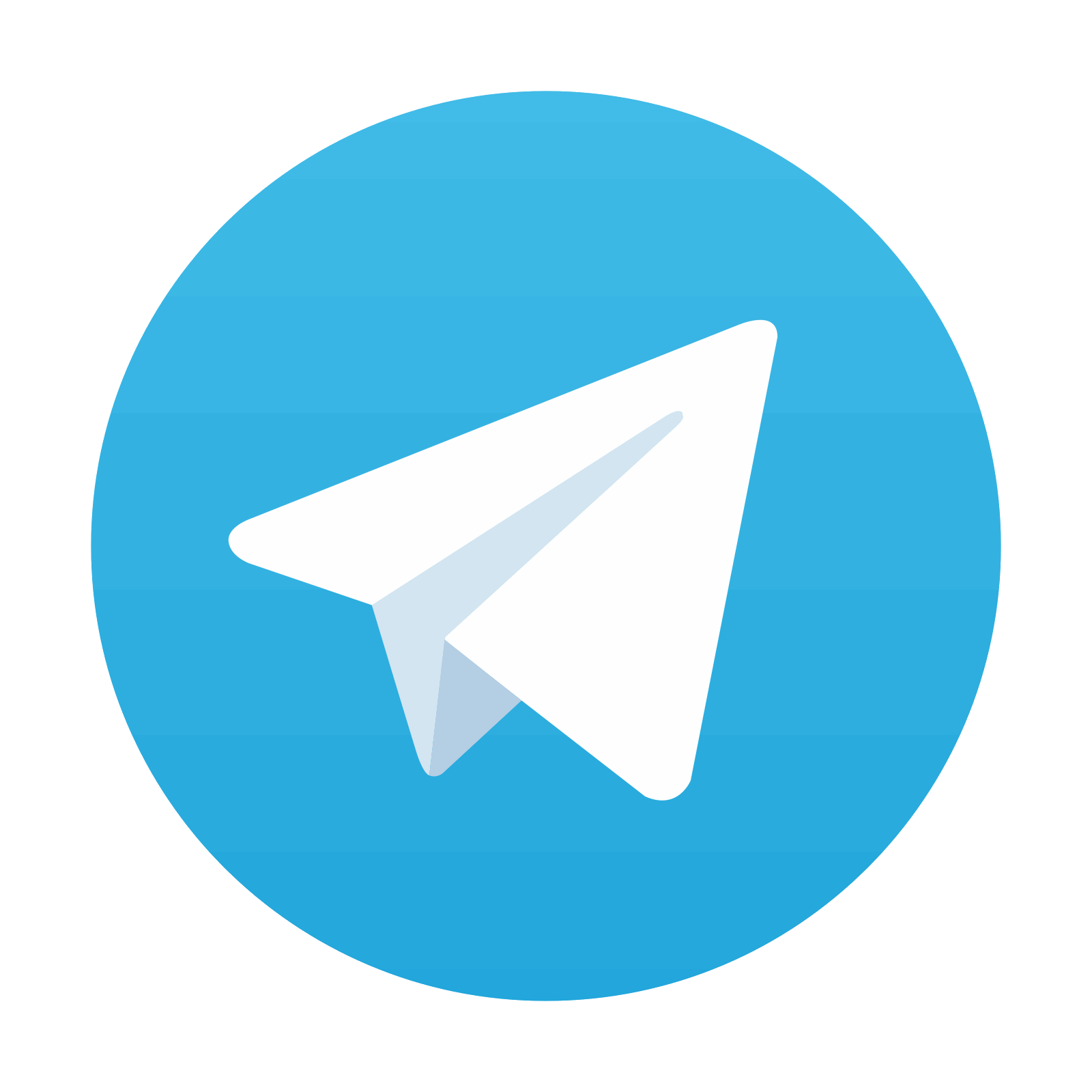
Stay updated, free articles. Join our Telegram channel

Full access? Get Clinical Tree
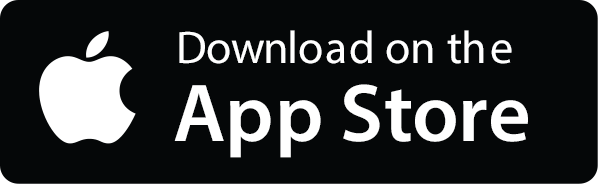
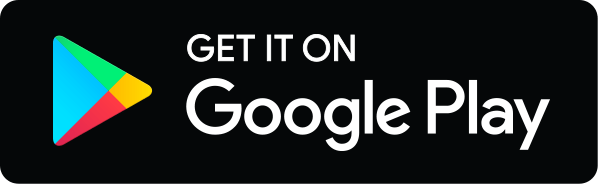