Coronary Fractional Flow Reserve Based on Computed Tomography
Javaid Iqbal, MRCP, PhD
Patrick W. Serruys, MD, PhD
▪ Introduction
Coronary angiography is the standard imaging modality for diagnosis and management of coronary artery disease in contemporary practice. However, its invasive nature and potential limitations have led to the development of noninvasive imaging modalities to investigate the extent and significance of coronary artery disease. Recent advancements in image processing, modeling, and computational fluid dynamics offer calculation of coronary artery pressure and flow from conventional coronary computed tomography (CT) scans. Computed fractional flow reserve (FFRCT) is the ratio of mean coronary artery pressure divided by mean aortic pressure under conditions of simulated maximal coronary hyperemia, thus providing a noninvasive estimate of fractional flow reserve (FFR). Clinical trials have shown that FFRCT improves diagnostic accuracy and discrimination compared to CT angiography alone for the diagnosis of hemodynamically significant coronary artery disease, when compared to invasive FFR as the reference gold standard. This promising new technology provides a combined anatomic and physiologic assessment of coronary artery disease in a single noninvasive test that can help select patients for invasive angiography and revascularization or optimal medical therapy. This chapter will discuss the rationale, technique, clinical application, limitations, and future of noninvasive FFRCT.
▪ Invasive Assessment of Coronary Artery Disease
Coronary Angiography
Sones performed the first selective coronary angiogram in 1957, enabling physicians to visualize the extent and severity of atherosclerotic coronary disease.1 Coronary angiography completely revolutionized cardiovascular medicine and has remained the gold standard for defining coronary anatomy during the last 50 years. It has made a substantial impact on the diagnosis and management of ischemic heart disease. Patients with significant lesions seen on angiography can undergo mechanical revascularization, either coronary artery bypass grafting or percutaneous coronary intervention.2
Limitations of Invasive Angiography
Although the utility of coronary angiography in contemporary clinical practice remains undisputed, it can often underestimate or overestimate the severity of a lesion. Angiography displays coronary cross-sectional anatomy from a planar two-dimensional silhouette of the contrast-filled vessel lumen. However, coronary lesions are often complex, with markedly distorted or eccentric luminal shapes, and angiography can misrepresent the severity of luminal narrowing.3 Additionally, visual interpretation of coronary angiography has a significant intra- and interobserver variability.4,5 The angiographic severity of a lesion poorly correlates to postmortem histology.6,7 More importantly, the relationship between stenosis and myocardial ischemia is complex, and whether the lesion is hemodynamically or functionally significant often cannot be reliably assessed by coronary angiography.8 The nuclear substudy of COURAGE (Clinical Outcomes Utilizing Revascularization and Aggressive Drug Evaluation) trial showed that among patients with ≥70% stenosis, only 32% exhibited severe ischemia and 40% manifested no or mild ischemia according to myocardial perfusion scintigraphy.9
Potential Solutions
The main limitations of coronary angiography, that is, invasive nature and lack of hemodynamic significance, can be solved by noninvasive testing for coronary artery disease and assessment of FFR (Table 6.1). FFR is traditionally assessed invasively, but noninvasive FFR assessment can solve both limitations. A multitude of noninvasive imaging modalities offer functional assessment of coronary artery disease by identifying regional differences in coronary flow reserve or wall motion abnormalities.10,11 Dobutamine stress echocardiography, adenosine stress first-pass perfusion magnetic resonance imaging, and myocardial single photon emission tomography (SPECT) are well-established modalities. While these serve as useful surrogate for ischemia, they do not directly visualize coronary stenoses or assess the hemodynamic significance of individual coronary lesions. Furthermore, these noninvasive stress tests have significant false-positive and false-negative rates when compared with coronary angiography.12 This may result in either unnecessary referral for invasive tests or falsely misclassifying the
patient as low risk. Accordingly, some experts advocate for hybrid imaging with physiologic and anatomic evaluation of CAD by stress testing and CT.13,14 However, this approach requires two tests and is associated with higher costs, time delay, and a greater radiation burden for the patient.14 A long-standing goal of noninvasive coronary imaging has been to develop a single test that identifies highgrade stenosis and determines its functional significance.15,16 The recent developments in CT-derived FFR assessment combined with the anatomic imaging capabilities of CT provide such a combined anatomic-physiologic assessment of coronary artery disease from a single imaging test.17
patient as low risk. Accordingly, some experts advocate for hybrid imaging with physiologic and anatomic evaluation of CAD by stress testing and CT.13,14 However, this approach requires two tests and is associated with higher costs, time delay, and a greater radiation burden for the patient.14 A long-standing goal of noninvasive coronary imaging has been to develop a single test that identifies highgrade stenosis and determines its functional significance.15,16 The recent developments in CT-derived FFR assessment combined with the anatomic imaging capabilities of CT provide such a combined anatomic-physiologic assessment of coronary artery disease from a single imaging test.17
TABLE 6.1 Limitation of Coronary Angiography and Potential Solutions | ||||||||||||
---|---|---|---|---|---|---|---|---|---|---|---|---|
|
Fractional Flow Reserve
FFR measurement is based on the relationship between coronary artery pressure and blood flow. This relationship is quite variable at rest; however, it is almost linear during maximal hyperemia as the peripheral resistance is minimal and constant.18 FFR is thus defined as the ratio of maximal blood flow on either side of a coronary artery stenosis (distal vessel flow divided by proximal vessel flow during maximal coronary hyperemia).19,20 FFR is conventionally measured during invasive angiography by using a guide wire with a pressure-sensing transducer that is placed across the stenotic lesion. After induction of maximal hyperemia by using a vasodilator agent such as intravenous or intra-arterial adenosine, the pressure gradient across the lesion is recorded, and FFR is calculated as the mean distal coronary pressure divided by mean aortic pressure. Coronary stenoses with FFR less than 0.75 are almost always functionally significant, whereas lesions with FFR greater than 0.80 are rarely associated with inducible ischemia.20 Prospective, multicenter, randomized clinical trials have shown that FFR-guided revascularization provides a sustained clinical benefit with improved event-free survival and reduced health care expenditures compared with the traditional strategy of angiographic stenosis guidance.21,22 and 23 FFR has demonstrated its clinical utility in safely deferring coronary revascularization procedures for non-flow-limiting stenoses.24 A subanalysis of the FAME (Fractional Flow Reserve vs. Angiography for Multivessel Evaluation) trial demonstrated that two-thirds of coronary lesions with a diameter stenosis more than 50% were not ischemia producing. Routine measurement of FFR in patients with multivessel coronary artery disease who are undergoing PCI with drug-eluting stents significantly reduces the rate of the composite end point of death, nonfatal myocardial infarction, and repeat revascularization at 1 year.25 Patients with ischemia-causing stenoses benefit from revascularization25, whereas patients with hemodynamically insignificant stenoses require no intervention and experience favorable outcomes on medical therapy alone, with myocardial infarction and mortality rates of less than 1% per year.21,24 FFR is now considered to be the standard of care for guiding percutaneous coronary revascularization in both European and American guidelines.26,27 Despite the solid data for the utility of FFR to guide clinical decision making, adoption into daily clinical practice has been limited, and FFR is currently used in only a minority of coronary revascularization procedures. This may be due in part to the invasive nature of the procedure, the need for pharmacologic vasodilation, an expensive coronary pressure wire, and risks related to instrumentation of the coronary arteries. It has been proposed in the recent years that FFR can be derived from coronary angiography alone without the need for pressure wire or vasodilatation28,29; however, it still requires invasive coronary angiography. Hence, there is a definite need for a noninvasive method to determine the functional significance of individual coronary lesions.
▪ Computed Tomography to Assess Coronary Artery Disease
Noninvasive evaluation of coronary artery disease can be readily performed using CT scanning.
CT Angiography
Computed tomography angiography (CTA) can provide high-resolution anatomic imaging of the coronary arteries.30,31 CTA is emerging as noninvasive tool of choice for the detection, characterization, and quantification of coronary atherosclerotic plaques.32,33 and 34 It is commonly employed for exclusion of coronary artery disease and demonstration of the extent of coronary artery disease. Accuracy of quantitative measurements for lumen, vessel, and plaque volume derived from multislice computed tomography (MSCT) scans in comparison with intravascular ultrasound (IVUS) gray scale was assessed in a cohort of 47 patients: An excellent correlation and a relatively acceptable agreement were shown.33 In another study, Voros et al.34 analyzed the relationship between MSCT- and IVUSderived measures for lumen and plaque area. They concluded that for lumen and vessel areas, good correlations exist with a coefficient higher than 0.7 and a systematic error of about 20%, MSCT values being consistently overestimated. Regarding percentage of
atheroma volume, the correlation was only modest with a coefficient of correlation close to 0.5, and in general, the Bland-Altman plot showed an acceptable mean difference though with relatively wide limits of agreement. The same authors also reviewed the capability of MSCT to characterize tissues, using the classical threshold of more than 150 Hounsfield units (HU) for calcified plaque, 30 to 149 HU for high-density noncalcified plaque, and -100 to 30 HU for low-density noncalcified plaque. Only a modest correlation was found for both calcified and noncalcified plaque areas, and both components were excessively overestimated by CTA with rather poor overall agreement. A number of other prospective, multicenter studies have demonstrated high diagnostic performance for the identification and exclusion of anatomically obstructive coronary artery disease compared to ICA.35,36 and 37 One of the intrinsic limitations of CTA in assessing plaque characteristics is the fact that the contrast density of the lumen impacts on the plaque density in the surroundings of the lumen.38,39 Despite this limitation, computer algorithms have been developed, which can differentiate between diverse types of tissue based on MSCT.34,40,41 and 42
atheroma volume, the correlation was only modest with a coefficient of correlation close to 0.5, and in general, the Bland-Altman plot showed an acceptable mean difference though with relatively wide limits of agreement. The same authors also reviewed the capability of MSCT to characterize tissues, using the classical threshold of more than 150 Hounsfield units (HU) for calcified plaque, 30 to 149 HU for high-density noncalcified plaque, and -100 to 30 HU for low-density noncalcified plaque. Only a modest correlation was found for both calcified and noncalcified plaque areas, and both components were excessively overestimated by CTA with rather poor overall agreement. A number of other prospective, multicenter studies have demonstrated high diagnostic performance for the identification and exclusion of anatomically obstructive coronary artery disease compared to ICA.35,36 and 37 One of the intrinsic limitations of CTA in assessing plaque characteristics is the fact that the contrast density of the lumen impacts on the plaque density in the surroundings of the lumen.38,39 Despite this limitation, computer algorithms have been developed, which can differentiate between diverse types of tissue based on MSCT.34,40,41 and 42
Beyond luminography and plaque characterization, MSCT has so far been unable to provide a functional assessment of the severity of coronary stenosis. Even among severe stenoses identified by CT and confirmed by angiography, less than half are functionally significant when compared to FFR.17,43 This unreliable relationship between severity of stenosis and functional significance has raised concerns that the use of coronary CTA may lead to unnecessary invasive angiography and unneeded revascularization procedures.17,44
CT Myocardial Perfusion Imaging
To overcome the limitation of CTA, one potential strategy is to combine CTA with CT perfusion imaging. Experimental work has validated multidetector CT-based estimates of myocardial perfusion against microsphere-derived measurement of myocardial blood flow in a canine model.45 Recently, several clinical trials have shown the feasibility and diagnostic accuracy of adenosine stress CT perfusion imaging for the detection of perfusion abnormalities, which is comparable to those of SPECT.46,47 and 48 George et al. studied 40 patients with abnormal SPECT perfusion imaging who underwent adenosine stress CT perfusion and coronary CTA.48 In a subset of 27 patients who underwent invasive coronary angiography, CT perfusion imaging, when combined with coronary CTA, accurately predicted coronary stenosis causing perfusion deficits as compared to the combination of quantitative coronary angiography and SPECT as a reference standard with sensitivity, specificity, positive predictive value, and negative predictive value of 86%, 92%, 92%, and 85%, respectively. Blankstein et al. reported the diagnostic accuracy of adenosine stress CT perfusion for the identification of hemodynamically significant stenosis as compared with that of SPECT.47 The study included 34 patients who underwent SPECT and invasive coronary angiography. On a per-vessel basis, CT perfusion alone had a sensitivity of 79% and a specificity of 80% for the detection of stenosis ≥50%, while those for SPECT perfusion imaging were 67% and 83%, respectively (Fig. 6.1). For the detection of vessels with ≥50% stenosis with a corresponding SPECT perfusion abnormality, CT perfusion had a sensitivity of 93% and a specificity of 74%. A distinct benefit of CT perfusion imaging is the ability to simultaneously visualize both anatomical stenosis and its physiologic consequences. However, there are several limitations that are pertinent to CT perfusion imaging. First, although the radiation exposure of comprehensive cardiac CT examination was equivalent to that of SPECT,47 it is still high. Second, in comparison to conventional coronary CTA, a larger volume of iodinated contrast is required as both rest and stress images are acquired. Third, CT perfusion imaging performed during adenosine infusion increases heart rate and thus the likelihood of artifactual reduction in CT attenuation densities caused by cardiac movement, partial volume effects, and beam-hardening artifacts, which can be mistaken for myocardial perfusion deficits. Finally, CT perfusion imaging does not provide lesion-specific ischemia data and cannot distinguish between diffuse disease and stenoses, which may be targets for revascularization.49
CT Fractional Flow Reserve
Over the last decade, research in the field has made major progress and some landmark discoveries, such as the introduction and validation of patient-specific 3D blood flow analysis, the development of adaptive finite element models for simulating cardiovascular blood flow, the definition of physiologically realistic inflow and outflow boundary conditions, as well as the coupled description of blood flow and vessel wall dynamics.50 The recent introduction of a novel computational method has enabled the calculation of FFRCT without the need for additional imaging, medication, or modification of CT acquisition protocols.51
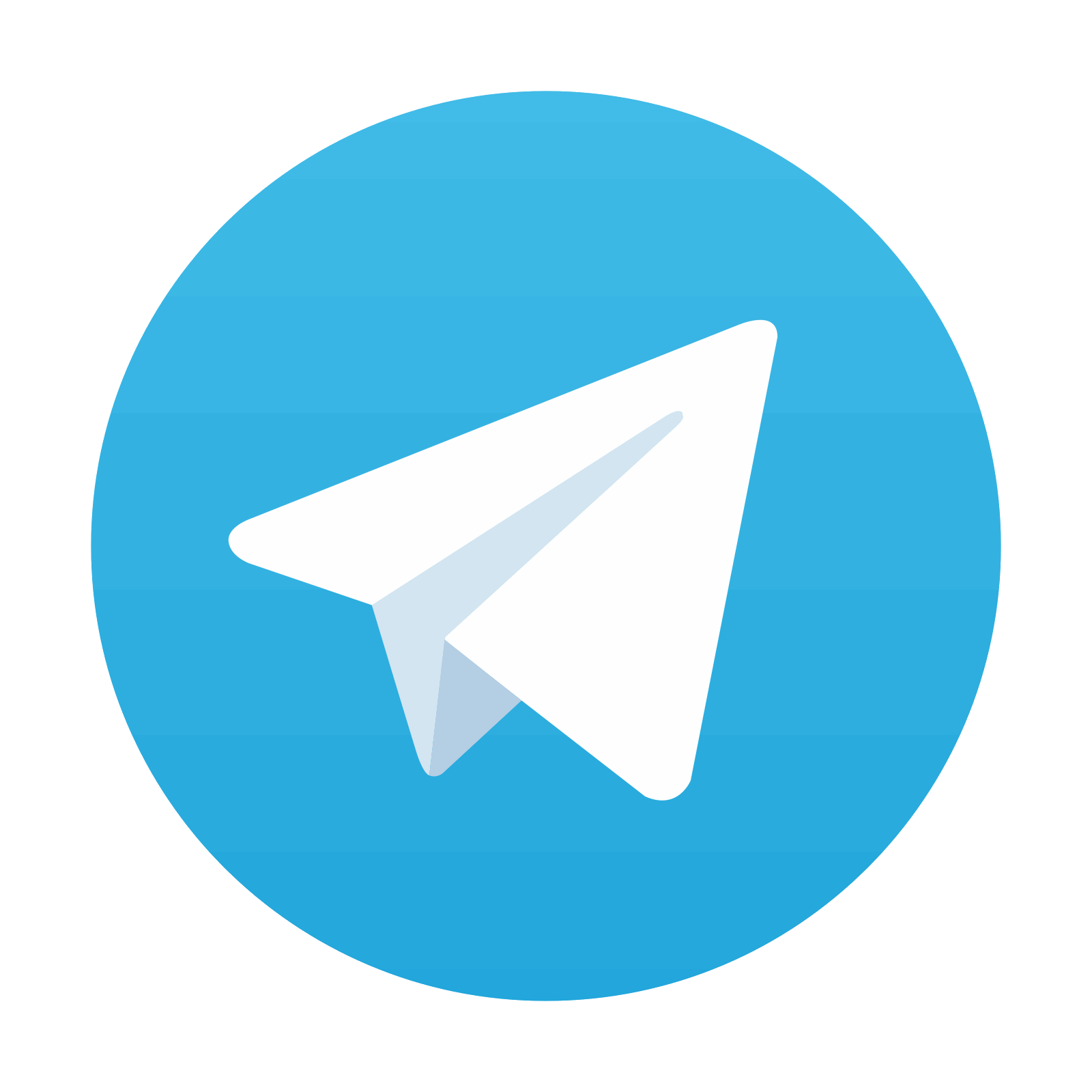
Stay updated, free articles. Join our Telegram channel

Full access? Get Clinical Tree
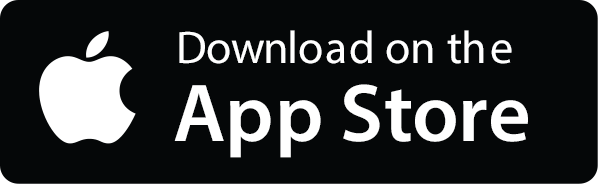
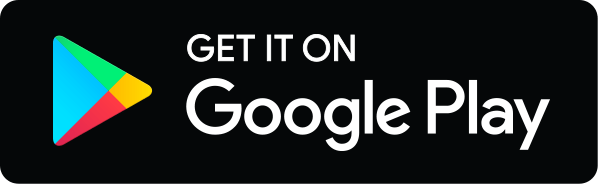