Fig. 2.1
Displaced bowel loops in pregnant patients. Left: A 19-week pregnant patient with Crohn’s disease. The sigmoid colon (arrow) is displaced by the pregnancy upward and to the left. Right: A 32-week pregnant patient with suspected Crohn’s disease. Twin pregnancy displacing the bowel loops upward and to the left. Crohn’s disease was ruled out. Acquisition sequence: FIESTA (steady-state free precession sequence) in coronal plane. (TR/TE = 4.2–5/1.2–2.3 ms, slice thickness 6 mm, no gap, FOV of 36–44 cm2, acquisition matrix of 384 × 384, NEX o = 1, flip angle 60°
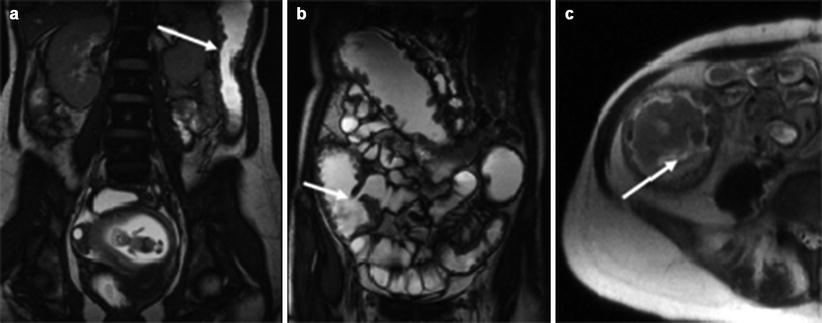
Fig. 2.2
Inflammatory signs in a non-contrast-enhanced study of an 11-week pregnant patient with colitis. (a, b) Colonic mural thickening (arrows) coronal FIESTA. (c) Submucosal edema (arrow) Axial SSFSE T2 w TR 1680–3200, TE 92.7 ms
In the fetus, situs anomalies may occur as part of deranged development (e.g., [73]). The problem is compounded many fold by the inherently changing landscape of fetal size, anatomy, tissue composition, and organ physiology.
The small size of the fetus dictates using the smallest field of view (FOV) which encompasses the region of interest, though decreased signal-to-noise ratio at small FOVs may require an increase, such that the choice of FOV is ultimately sequence dependent. Similar considerations apply to matrix size and slice thickness, which determine the voxel size. While the size of the voxel needs to be appropriate for the target size, SNR is inversely related to matrix size and all of these parameters need to be adjusted so as to minimize imaging time, to reduce motion artifacts (above). Slice thickness as low as 1.6 mm (no gap) has been reported [27] with specific fast precession sequences, but generally slice thickness of less than 3 mm leads to substantial decreases in SNR.
There are no specialized MRI coils available for fetal imaging, so coils need to be used which can be placed in close proximity to the target. Coils used for fetal imaging include the 1.5T HDx GE coil, body coils, and cardiac coils, with best results achievable with flexible multichannel coils. This obviates some of the problem, but lateral decubitus position or obesity of the mother, increasing the distance between the fetal target and the coil, may still result in degraded image quality due to low signal intensity.
In contrast with adult size and anatomy, where the range of “normal” values is restricted, fast changes in fetal organ size and anatomy mean that norms and baselines need to be established for every week of pregnancy, a momentous task and ongoing effort pioneered by Garel and her group [24], who provided biometric data and continue with the creation of a spatiotemporal atlas of MR intensity, tissue probability, and shape of the fetal brain [33].
Tissue composition is also different in fetuses and changes through development. In the fetal brain, water content is relatively high and lipid content is extremely low since myelination is a relatively late-occurring phenomenon, requiring specific sequence adaptation with increasing fetal age as described below.
Several examples of sequences used in the production of the illustrations to this chapter, focusing on the brain, are given in Table 2.1. Additional sequences and protocols can be found in the literature (e.g., [80, 105]) and in subsequent chapters of this book.
Table 2.1
Examples of fetal brain MRI protocols on 1.5 GE OPTIMA, 750-W scanner
Generic name | FOV (mm) | Thickness/gap (mm) | Matrix | TR (ms) | TE (ms) | Flip angle (degrees) | Number of slices | Time (s) |
---|---|---|---|---|---|---|---|---|
SS-FSE T2 | 30 | 4.0/0–3.0/0 | 320/224 | Shortest (800–1200) | 90 | 90 | 23 | 00:26 |
FSE T2 | 30 | 4.0/0–3.0/0 | 256/256 | 11,000 | 120 | 180 | 41 | 01:30 |
DWI | 30 | 4.0/0–3.0/0 | 128/128 | 6200 | min | 90 | 27 | 01:27 |
T1 FLAIR | 30 | 4.0/0–3.0/0 | 256/256 | 1657 | 51 | 160 | 25 | 02:47 |
2.4.1 Structural (T2- and T1-Weighted) Fetal MRI
T2-weighted sequences are the most amenable to studies of fetal anatomy. Single-shot fast spin echo (SSFSE) are very useful in clinical studies of anatomical malformations since the information for an entire image is acquired in less than 1 s, with good contrast and resolution (Fig. 2.3), although sequence parameters need to be continuously adjusted to fetal age, with longer TEs required for younger fetuses with higher water content [13]. When fetal motion is restricted, better contrast can be achieved with fast-recovery fast spin echo T2-weighted sequences (Fig. 2.4). In addition, T2* imaging is useful for evaluating vascular lesions, hemorrhages, and calcifications (Fig. 2.5). Fluid-attenuated inversion recovery (FLAIR) sequences, useful for visualizing white matter pathology in adults, is not very useful in fetuses due to the high water content. With water suppression, it may have some use in differentiating gray and white matter lesions.
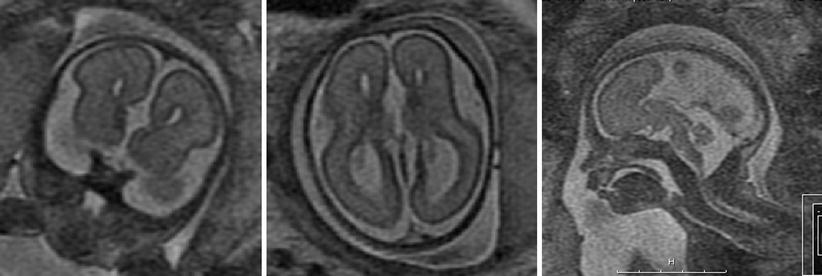
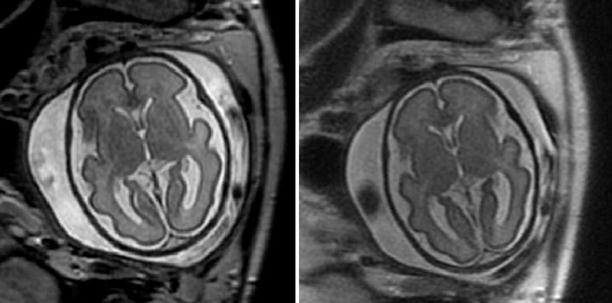
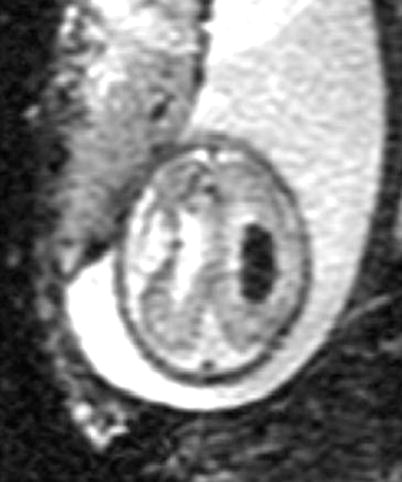
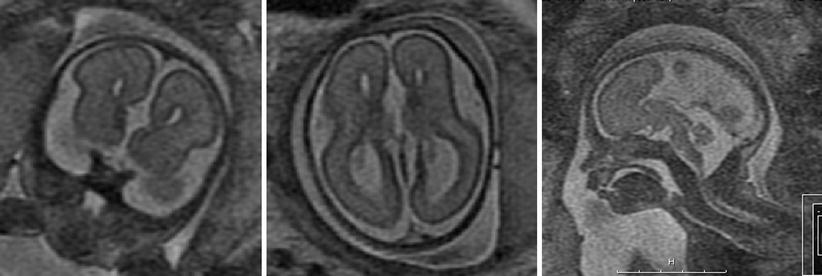
Fig. 2.3
Agenesis of the corpus callosum detected with SSFSE T2-weighted 28-week GA fetus with agenesis of the corpus callosum. Left: coronal view, note parallel lateral ventricles. Middle: axial view. Ventricles are parallel, the atria are dilated. Right: sagittal view. The corpus callosum is missing in the midline and midline sulci extend all the way to the third ventricle
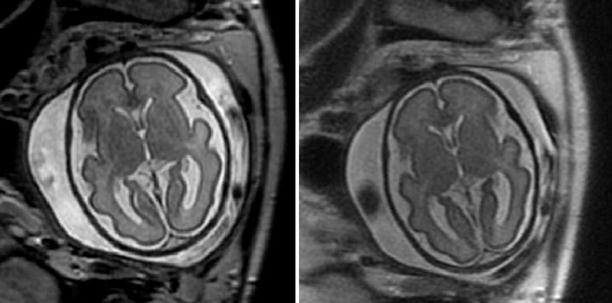
Fig. 2.4
Optimizing gray/white contrast in fetal CNS. Normal fetus, 30 weeks GA. Left: axial image acquired with fast-recovery fast spin echo (FRFSE) T2-weighted sequence, most suitable for detection of cortical abnormalities in fetuses with limited motion. Note the sharp gray/white contrast. Right: same fetus imaged with SSFSE T2-weighted sequence
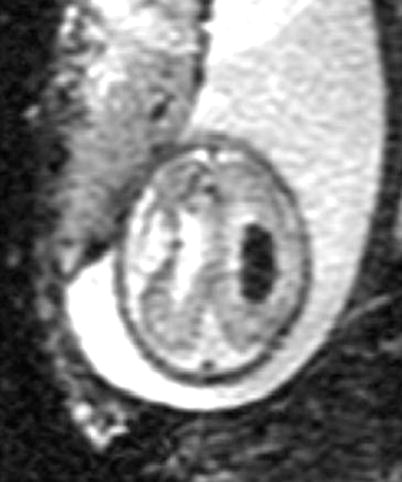
Fig. 2.5
Visualization of blood with T2* imaging. A 30-week GA fetus with ventricular enlargement and grade III intraventricular hemorrhage. Note prominent appearance of blood inside the ventricle
T1-weighted images are of lower signal intensity-to-noise ratio and require longer acquisition times (18 s), with consequent increased susceptibility to both maternal and fetal motion. Consequently, the utility of T1-weighed imaging to fetal MRI is limited relative to adults. Still, T1-weighted imaging is necessary to visualize the small amount of myelinated tissue present in the fetal brain (Fig. 2.6). Fast multiplanar gradient-recalled echo techniques, such as FMPSPGR (fast multiplanar spoiled gradient-recalled acquisition in the steady state), are useful for detection of hemorrhaging or calcification in brain and other organs (e.g., [110], Fig. 2.7). For best results, images are acquired during a single maternal breath hold with the shortest feasible times.
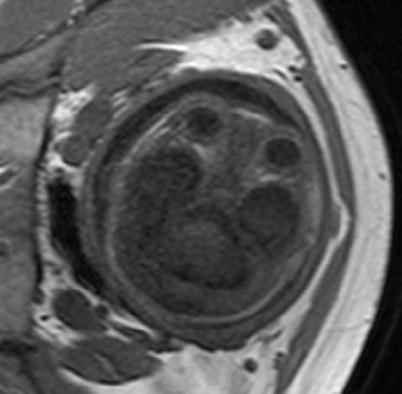
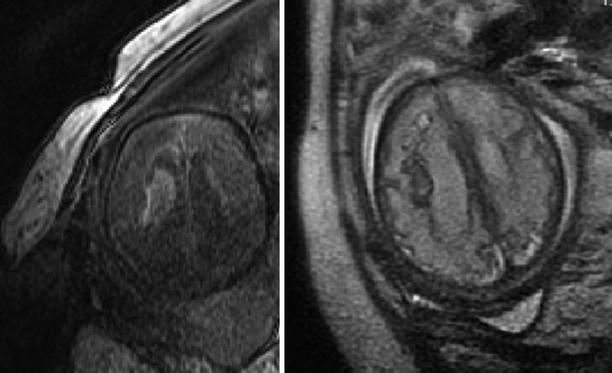
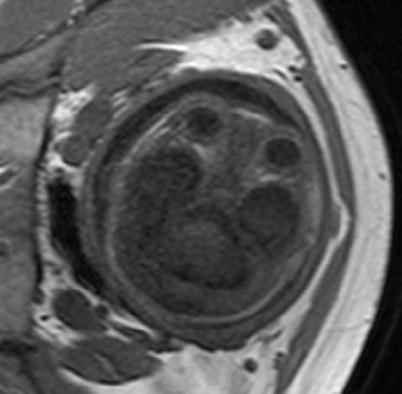
Fig. 2.6
Visualization of myelin with a T1-weighted sequence. A 32-week GA normal fetus. Myelinated white matter (hyperintense area) can be observed in the posterior part of the brainstem
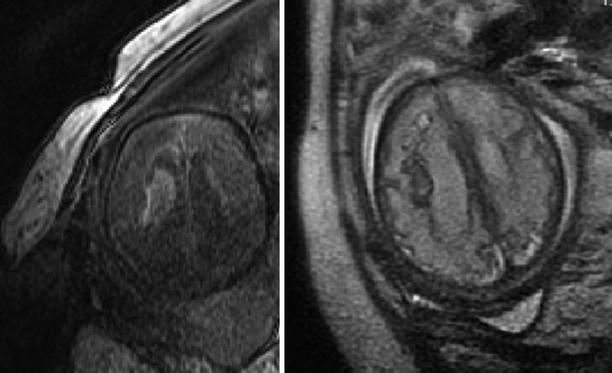
Fig. 2.7
Comparison of T1 and T2 sequences in visualization of fetal AVM. A 35-week GA fetus with AVM (confirmed postmortem after termination of pregnancy). The subacute blood appears hyperintense in the T1 FSPGR sequence (left) and hypointense in the T2 sequence (right)
2.4.2 Diffusion Weighted Imaging
Diffusion weighted imaging is uniquely sensitive to acute ischemia, thus capable of visualizing fetal strokes before they lead to signal changes in T2- and T1-weighted sequences ([4]; Fig. 2.8). Similarly, progressive increases in diffusion anisotropy of white matter tracts from premyelinated to myelinating stages facilitate the visualization of white matter tracts weeks before they are visible on T1- and T2-weighted images [79]. The technique is also useful in detection of blood products (Fig. 2.9) since echoplanar DWI is extremely sensitive to susceptibility effects. Due to high water content in the fetal brain, best contrast of echoplanar DWI images is achieved with relatively low b values, in the range of 400–700 [80].
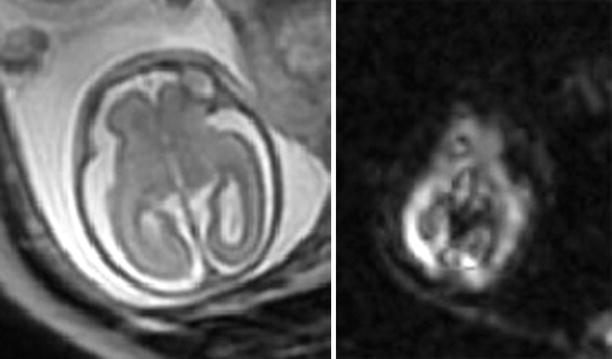
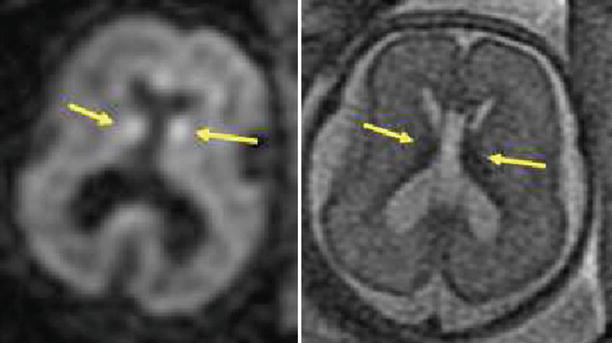
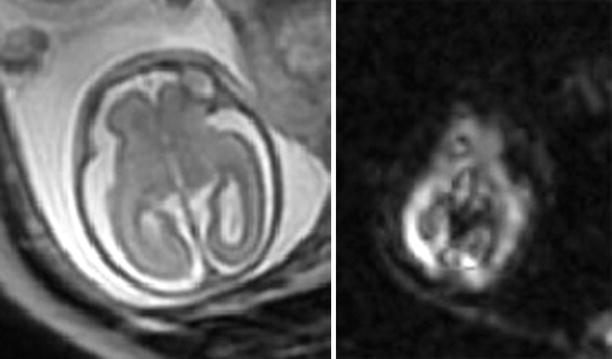
Fig. 2.8
Acute ischemia detected by DWI. A 23-week GA fetus surviving the demise of an MCBA twin was imaged with T2-weighted (SSFSE) sequence 4 days later. MRI (left) as well as US appeared normal. DWI (right) demonstrated abnormal signal in the posterior part of the right hemisphere, consistent with acute ischemic insult
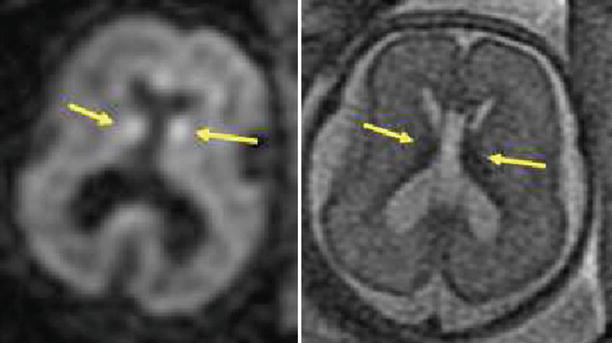
Fig. 2.9
Detection of hemorrhage with DWI. A 23-week GA fetus with small amount of bleeding in the germinal matrix bilaterally, seen as hyperintense spots on DWI (left image, arrows). In the T2 image (right), the blood is hypointense (arrows)
2.4.3 Summary: Clinical Applications
Safety considerations impose limitations on the use of MRI techniques involving contrast agents throughout pregnancy and the use of MRI in general in the first trimester, though MRI is still considered preferable to any imaging involving ionizing radiation. Due to motion, current clinical fetal brain imaging relies heavily on fast T2 sequences, with a more limited but important role for T1- and diffusion-weighted sequences in the detection of specific pathologies. In all cases, sequence parameters need to be optimized for gestational age and indication.
2.5 Where the Future Lies: Quantitative Mapping of Regional and Cellular Patterns of Fetal Maturation
2.5.1 Volumetry
An early study using the Cavalieri method to estimate fetal and whole brain volumes in a small cohort of third trimester fetuses [30] described a linear relationship between gestational age and whole brain volume, with a growth rate averaging 2.3 mL/day. This impression was dispelled as soon as newer sequences and image analysis methods, allowing volumetric measurements of smaller regions, were applied to larger cohorts of second and third trimester normal fetuses ([17, 26, 32, 34, 42, 90]). The findings from these studies consistently demonstrate region-specific, nonlinear growth trajectories which can be obtained with manual drawing of regions of interest on 2D images ([32]; Fig. 2.10) as well as automatic segmentation of motion-corrected, 3D-reconstructed MRI scans [90]. In the clinical setting, volumetry was successfully used to describe changes in brain and body volumes in fetuses exposed to cytomegalovirus (CMV) infection in early pregnancy ([38], Fig. 2.10), as well as fetuses with ventriculomegaly [77, 91] and intrauterine growth restriction [19].
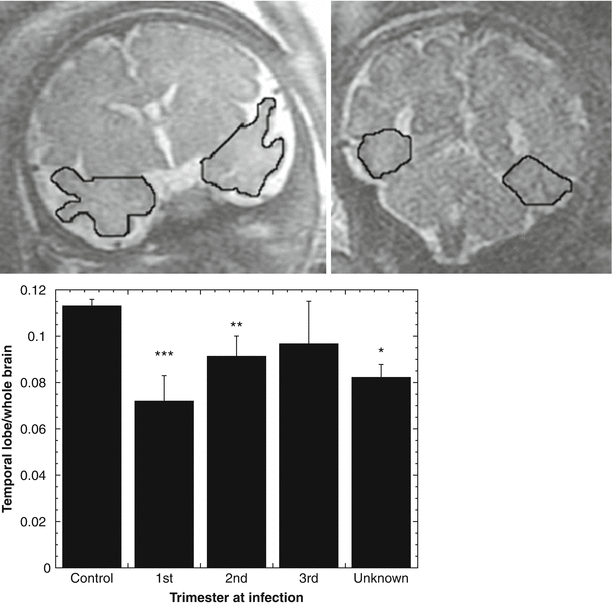
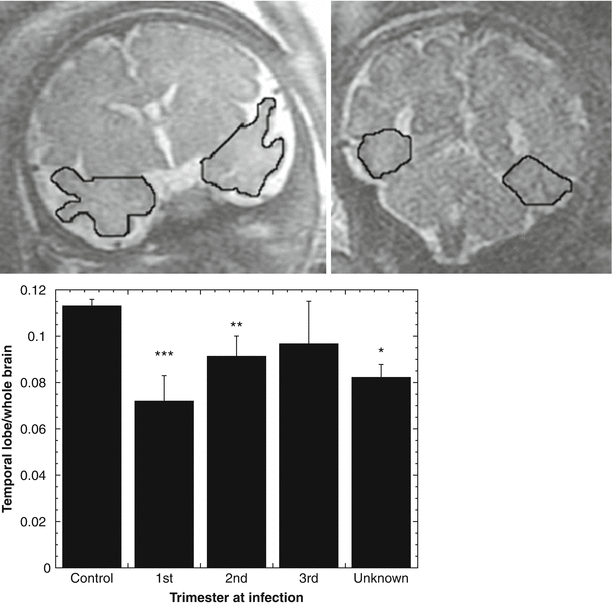
Fig. 2.10
Effect of CMV infection on regional brain volumes. MRI volumetry was employed to measure brain and temporal lobe size in 27 CMV-infected fetuses and 52 gestational age matched controls. The ratio of temporal lobe to whole brain was computed for each fetus. Top: example of manual ROI placement on temporal lobe, used for volume calculation. Bottom: temporal lobe/whole brain volume ratio was significantly reduced in fetuses infected with CMV during the first and second, but not the third trimester
2.5.2 Shape Changes/Cortical Development
Recent studies of fetal cortical development using advanced techniques reiterate the theme of region-specific, nonlinear maturation ([16, 35, 42, 43, 82]). Thus, Hu et al. (2011) provide a regional quantification of cortical shape development reporting faster shape changes in the occipital lobe than in other regions, while findings by Clouchoux et al. [16] demonstrate an exuberant third trimester gyrification process and suggest a nonlinear evolution of sulcal development. The same group also reported on delayed cortical development in fetuses with congenital heart disease [15]. Adaptation of tensor-based morphometry revealed that fetal brain development exhibits a distinct spatial pattern of anisotropic growth, with the most significant changes in the directionality of growth occurring in the cortical plate at major sulci. The authors also report significant directional growth asymmetry in the perisylvian region and the medial frontal lobe of the fetal brain [82].
2.5.3 Measurement of Apparent Diffusion Coefficients (ADC Mapping)
Regional differences and developmental changes in apparent diffusion coefficients (ADC) of normal fetuses have been the subject of several studies conducted and published during the last decade [7, 8, 10, 11, 39, 64, 83, 88]. All of the published studies report absolute values for ADC in a similar range and detect a trend toward a reduction in ADC with increased GA (Figs. 2.11 and 2.12), which could be explained by progressive myelination. However, the relationship between ADC and GA appears to be region dependent and nonlinear. Thus, in a study of 50 normal fetuses between 19 and 37 weeks’ gestation, ADC values remained constant in the basal ganglia, frontal, parietal, temporal, and occipital white matter and in the centrum semiovale while significant decreases were observed in the cerebellum, pons, and thalamus with advancing gestational age [7]. In the clinical setting, ADC values were reported to be decreased in specific brain regions of hydrocephalic [21] and CMV-infected fetuses [108].
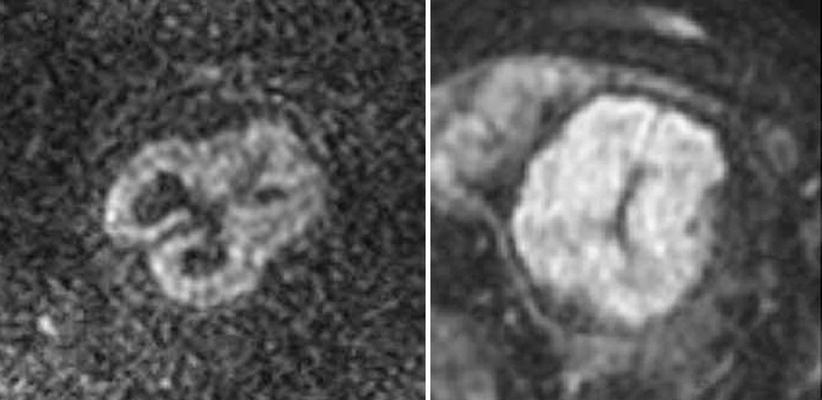
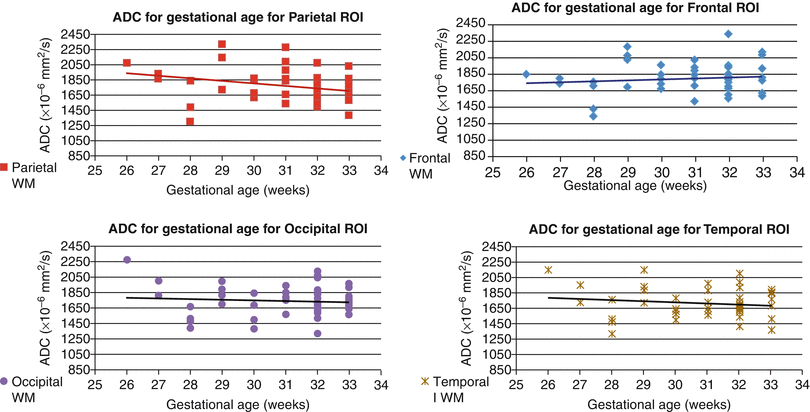
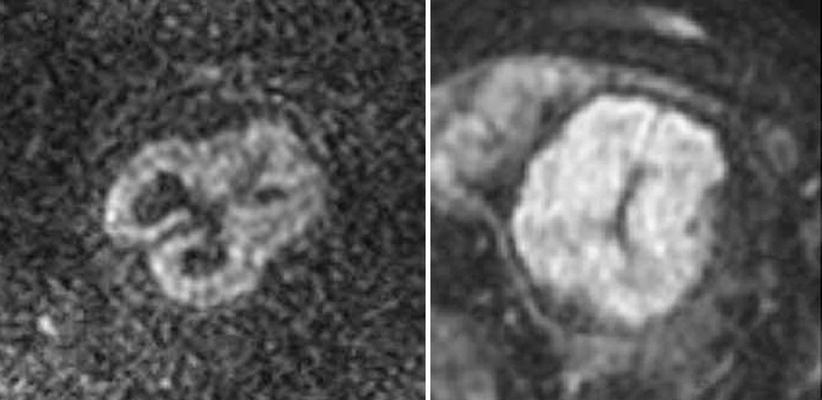
Fig. 2.11
Fetal brain maturation visualized with diffusion weighted imaging. Left: normal fetus, 26 weeks GA, Right: normal fetus, 32 weeks GA, diffusion weighted imaging in the axial plane. Note the change in signal intensity with maturation and decrease in the amount of unrestricted water
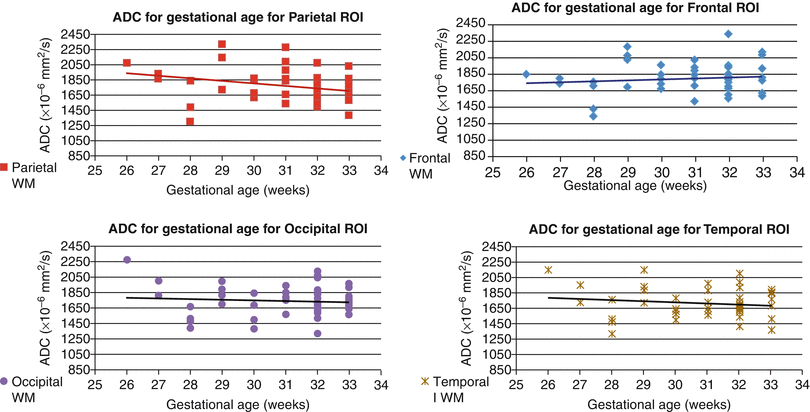
Fig. 2.12
Maturational changes in regional apparent diffusion coefficient of the fetal brain. Diffusion weighted imaging (DWI) was performed in 48 non-sedated third trimester fetuses with normal structural MRI results. ADC was measured in white matter (frontal, parietal, temporal, and occipital lobes)
2.5.4 Magnetic Resonance Spectroscopy
Several groups have demonstrated the ability to obtain useful information on fetal metabolism using H1 spectroscopy (e.g., [37, 55, 56]). Short TEs (~35 ms) are used to detect metabolites such as glutamine, glutamate, glucose, taurine, and lipids, while longer TEs (~140 ms) are useful in the detection of N-acetylaspartate (NAA, considered to be a neuronal marker), choline (Cho, a marker of membrane formation), and creatine (Cr, a marker of mitochondrial activity). Lactate, a marker of anaerobic metabolic, can be detected as well. The relatively long imaging time and large voxel size required for MRS limit the widespread use of this technique in clinical fetal imaging (Fig. 2.13).
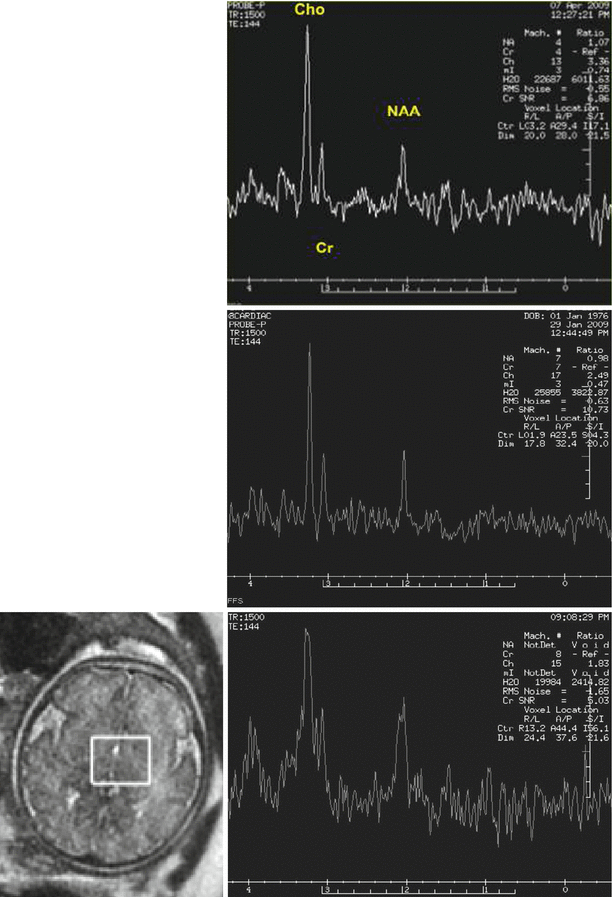
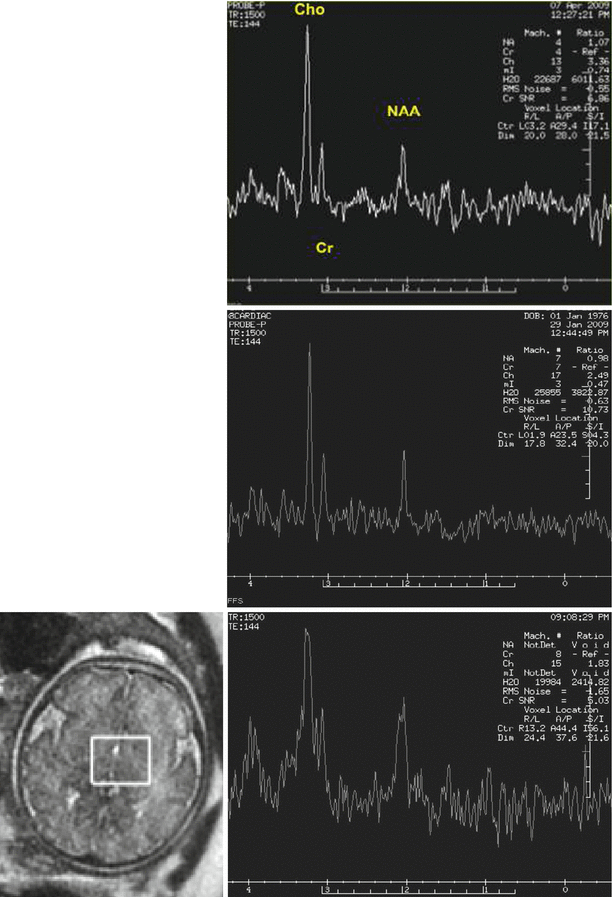
Fig. 2.13
H1 MRS of the fetal brain: Maturational changes and effects of motion. Top: normal fetus, 26 weeks GA. The choline (Cho) peak is very high (3.36 ppm) and NAA is relatively low. Middle: a 31-week GA fetus imaged because of reduced intrauterine motility reported by the mother. Baseline is low and peaks are sharp. Choline is still high (2.48 ppm) but lower than in the younger fetus. Bottom: MRS of 35-week-old fetus showing blurring due to fetal movement. NAA is increased relative to the younger fetuses. Location of voxel is shown on the left
Development of the normal fetal brain in utero using MRS has been studied by a number of groups. The size of the voxel necessary to acquire reliable information limits the possibility of regional measurements, so these studies mostly reflect whole brain maturation. With this caveat, levels of choline (Cho), creatine (Cr), myo-inositol (myo-ins), and N-acetylaspartate (NAA) have been more recently measured in utero in fetuses in the age range of 22–41 weeks [28, 29, 99]. Brain maturation was most clearly reflected by increasing levels of the neuronal marker NAA, with a parallel decrease in choline. In clinical populations, Limperopoulos and colleagues found significantly slower increase in the NAA:choline ratio in fetuses with congenital heart disorder. Predictors of lower NAA:choline included diagnosis, absence of antegrade aortic arch flow, and evidence of cerebral lactate [60]. A study of fetuses with intrauterine growth restriction (IUGR, [2, 12, 84]) detected a lactate peak in the brain of the most severely affected IUGR fetus, which was consistent with low oxygen content and high lactic acid concentration in umbilical blood obtained at delivery.
2.5.5 Diffusion Tensor Imaging (DTI) of Fetal Brain Connectivity
DTI is a relatively new technique, only recently applied to fetal imaging ([44, 49, 52, 54, 69, 71, 81, 86, 97]; Fig. 2.14). It may be used to map and characterize the 3D diffusion of water as a function of spatial location. The diffusion tensor describes the magnitude, the degree of anisotropy, and the orientation of diffusion anisotropy. Estimates of white matter connectivity patterns in the brain from white matter tractography may be obtained using the diffusion anisotropy and the principal diffusion directions. The technique is also highly sensitive to changes at the cellular and microstructural level [81, 86]. Unfortunately, DTI presents an even bigger challenge for in utero fetal imaging relative to other techniques since acquisition times are longer and therefore studies are more susceptible to motion artifacts. Consequently, only a few recent studies provide quantitative data from in utero studies of neuronal pathways. As an example, Kasprian et al. [52] examined a group of fetuses ranging in age from 18 to 37 weeks and reported that only in 40 % of examined fetuses, DTI measurements were robust enough to successfully calculate and visualize bilateral, craniocaudally oriented (mainly sensorimotor), and callosal trajectories in utero. However, the successful studies resulted in a wealth of quantitative information on fiber lengths, ADC, fractional anisotropy, and eigenvalues at different anatomically defined areas with high potential utility in understanding the etiology of neuropsychiatric disorders (e.g., [104]).
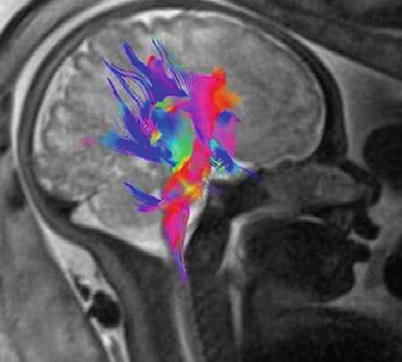
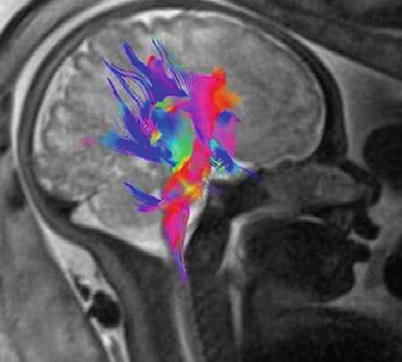
Fig. 2.14
Visualization of developing fiber tracts using diffusion tensor imaging. A 33-week GA normal fetus. DTI was acquired using a single-shot echoplanar spin echo sequence with the following parameters: TE/TR 105 ms/8900 ms, 50 contiguous slices, 2.2-mm thickness; matrix 128 × 128; FOV 256 × 256 mm2. Diffusion gradients were encoded in seven directions with b values of 1000 s/mm2 and an additional image with no diffusion gradient (b = 0 s/mm2). Three sets of DTI data were acquired for average and the total DTI acquisition time was 5 min 47 s. Image shows 3D fiber tracts projected onto the fetal brain. Note the corticopontine/corticospinal projection fiber (red) genu and the corpus callosum fibers (blue). Courtesy of Gabriele Masselli, MD; Sapienza University, Rome, Italy
2.5.6 Functional MRI
MRI allows for the noninvasive imaging of regional activation and functional connectivity. The feasibility of studying fetal brain activity with functional magnetic resonance imaging [96] (fMRI, or BOLD (blood oxygen level-dependent) MRI) was demonstrated by Hykin et al. [45] just before the turn of the century, reporting on fetal brain responses to maternal speech. This was followed by additional studies reporting the detection of responses to various acoustic [23, 47, 48, 70] and visual [22] stimuli, which were detected between 33 and 34 weeks of gestation. Functional connectivity (FC) at rest was subsequently demonstrated in the fetal brain [89, 103] in fetuses from 20 to 36 gestational weeks of age, documenting the presence of bilateral fetal brain FC as well as regional and age-related variation in the strength of FC between homologous cortical brain regions, which increased with advancing gestational age. Sorensen et al. [95] examined the BOLD response in the fetus and placenta following maternal hyperoxia, demonstrating an increased oxygenation in a number of fetal organs and in the placenta while oxygenation of the fetal brain remained constant. These studies together with findings from other modalities like fetal EEG and MEG [1] are truly revolutionary since unlike information on maturation of brain morphology and microstructure/chemistry which can be obtained postmortem, the development of function can only be studied in vivo.
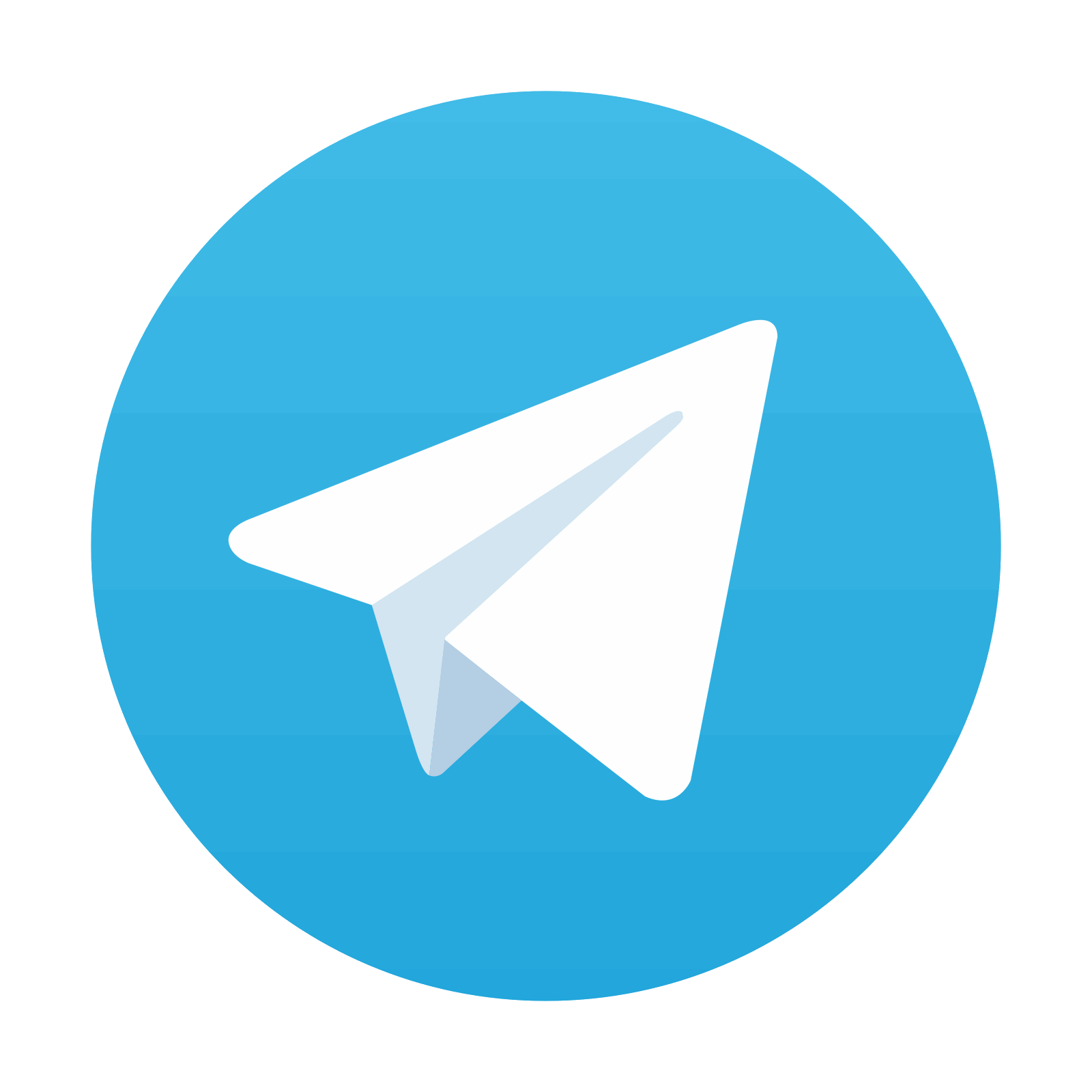
Stay updated, free articles. Join our Telegram channel

Full access? Get Clinical Tree
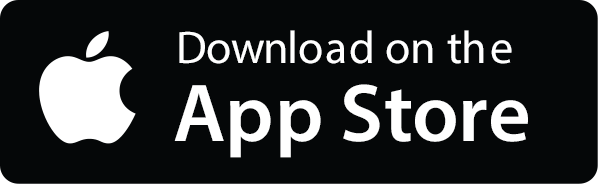
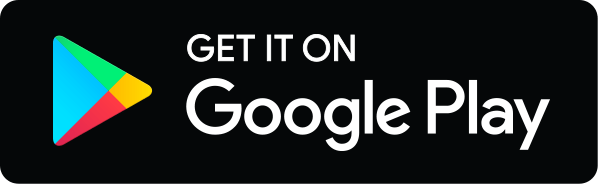