(1)
Cleveland Clinic, Emeritus Staff, Cleveland, OH, USA
Keywords
CyclotronTarget processingRadionuclide productionRadionuclide yieldIntroduction
Nearly 3700 nuclides are known to exist, of which approximately 280 are stable and the remainder are radioactive. The majority of radionuclides are artificially produced in the cyclotron and reactor. In PET technology, only positron-emitting radionuclides are required, and only a few positron emitters of all radionuclides have been suitably utilized in clinical studies. These radionuclides include 11C, 13N, 18F, 15O, etc., and are produced in the cyclotron. The operation of a cyclotron and the production of useful positron emitters are described below.
Cyclotron Operation
In a cyclotron (Fig. 7.1), charged particles (S) are accelerated in circular paths within the two D-shaped hollow metallic electrodes called the dees (A and B) under vacuum by means of an electromagnetic field. Charged particles can be either positive ions (e.g., proton, deuteron, α particle) or negative ions (e.g., negatively charged hydrogen atom, H−). The following is a description of a cyclotron to accelerate H− particles (negative ion cyclotrons).
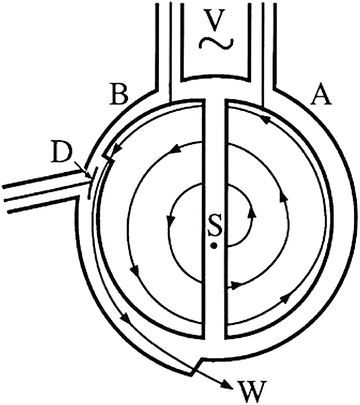
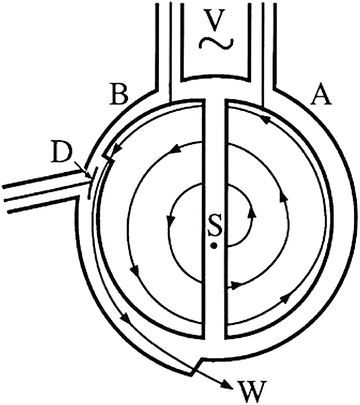
Fig. 7.1
A schematic illustration of a cyclotron: V alternating voltage, S ion source, A and B dees under vacuum, D carbon foil stripper, W window
The ions are obtained from an ion source (S) positioned at the center of the cyclotron. The ion source is a small chamber located between two negative high-voltage (V) (1–3 kV) tantalum cathodes. The hydrogen gas flows (4–10 mL/min) into the chamber. Electrons emitted from the cathodes and constrained by the magnetic field of the main magnet interact with the hydrogen atoms to form a plasma in the chamber that consists of three entities: protons, negatively charged hydrogen atoms H−, and neutral hydrogen atoms. In some cyclotrons, the gas is ionized by applying 20 kV DC, producing proton and H−. Protons or negative hydrogen ions (H−) are pulled out of the ion source chamber through a narrow slit by the electrostatic force depending on the polarity of the dees.
The two dees (A and B) are half-pie-shaped, troughlike, hollow copper structures connected to an alternating high-voltage (30 kV) oscillator operating at radiofrequencies of 20–30 MHz and are positioned between the two poles of an electromagnet. In some cyclotrons, there are four dees, which look like a quarter of a pie. The inside of the dees is kept at extremely high vacuum (10−6 mtorr for positive ions and 10−7 mtorr for negative ions). Since H− can lose its electrons on interaction with any molecule inside the dee, such high vacuum is essential in negative ion cyclotrons, which is tenfold higher than in positive ion cyclotrons. Charged ions are attracted toward a dee with an opposite charge during the cycle of oscillating radiofrequency voltage waveforms and pass into the hollow dee, while in the dee, ions do not experience any electrical field, but are subjected to a magnetic field provided by a large electromagnet (up to 2 T). Under the magnetic field, the path of the ions bends such that as the ions reach the edge of the dee, the polarity of the dee is changed. At this moment, the ions are repelled by the dee and also attracted toward the opposite dee, thus gaining more kinetic energy to accelerate. The radiofrequency is so synchronized that every time the ions cross the dee intersection, the polarity of the dee is changed and the ions gain energy and travel in a larger trajectory in the dee. For a particular cyclotron, the energy of the ions depends on the radius of the cyclotron, magnetic field, and charge and mass of the ion. Thus, the kinetic energy (KE) of the ion is given by
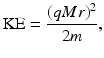
where q is the charge of the particle, M is the magnetic field in gauss, r is the radius of the cyclotron, and m is the mass of the particle.
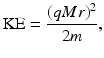
(7.1)
Since negative H− ions do not interact with the nuclei of the target and positive ions do, a 2- to 5-μm-thick and <4 cm in diameter carbon foil (D) is inserted vertically inside the cyclotron just ahead of the target position to strip two electrons to produce positive ions (i.e., protons), which then can cause nuclear reactions in target nuclei. The carbon foils are loaded in a carousel, and multiple such carousels (e.g., 2–6) are placed in appropriate locations near the exit inside the cyclotron. The carousels are spun under computer control providing multiple beam lines as needed. The negative ion cyclotron offers the scope of dual bombardments where two targets can be irradiated simultaneously. A carousel containing the carbon-stripping foil is inserted in the path of the beam in such a way that only a part of the beam passes through it producing a positive ion beam that is steered out to the beam line leading to a target for radionuclide production. The remainder of the beam is intercepted by a carbon foil in another carousel further down the orbit to produce a second positive ion beam aimed at another target. Two separate targets can be used for the production of two different radionuclides (e.g., 18F and 13N) or two same targets may be used to produce a larger quantity of the same radionuclide. The kinetic energy of the accelerated particles can vary from a few MeV to several hundred MeV depending on the size and design of the cyclotron. For a given cyclotron, the external beam energy remains constant, whereas the internal beam energy varies radially inside the cyclotron. Various particles, namely, α particle, proton, deuteron, 3He, and a few heavy ions can be accelerated depending on the design of the cyclotron. The operation of cyclotrons is mostly automatic with minimal manual input and is controlled by the computer.
There are several features that make the negative ion cyclotron operationally more advantageous than the positive ion cyclotron. Beam extraction in a positive ion cyclotron is accomplished by steering the beam along a relatively long channel under the application of an electrostatic force with an extraction efficiency of ~80%. The remaining 20% of the positron beam is lost inside the cyclotron inducing radioactivity in the housing. This obviously warrants more shielding around the cyclotron unit. In contrast, beam extraction in a negative ion cyclotron by carbon foil stripping is a simple method. Because of the short path of extraction and an extraction efficiency of almost 100%, cyclotron housing is not activated by negative ions and shielding requirement is less than in positive ion cyclotrons. In a negative ion cyclotron, the particle beam can be split into several beam lines providing the scope of irradiating several targets simultaneously. On the other hand, a positive ion cyclotron is limited to only a single beam line because of the large size of the electrostatic deflector. However, since H− can lose the electrons by an encounter with any molecule producing positive ions, the vacuum inside the negative ion cyclotron needs to be extremely high compared to the positive ion cyclotron.
A point of consideration for the positive ion cyclotron is that the internal beam of positrons of variable energy can be utilized for radionuclide production by placing a probe with the target at different radial positions. The negative ion cyclotron does not have this provision.
Medical Cyclotron
Medical cyclotrons are compact cyclotrons that are primarily used to produce short-lived, positron-emitting radionuclides used for PET imaging. Most of the clinically useful positron emitters are formed by nuclear reactions with low-energy particles and hence the compact cyclotrons. These are commercially available and can be installed in a relatively small space. Moreover, in medical cyclotrons, negative hydrogen ions (H−) are commonly accelerated, because unlike in positive ion cyclotrons, the housing of the cyclotron does not become radioactive in negative ion cyclotrons. Also, the beam can be split by two carbon-stripping foils so that two targets can be irradiated simultaneously. Whereas the particle energy may be in the range of a billion electron volts in high-energy cyclotrons, the typical energy of the protons or H− particles in medical cyclotrons ranges between 10 and 30 MeV. In some medical cyclotrons, both deuterons and H− particles can be accelerated interchangeably by switching the ion sources between deuterium and hydrogen. Shielding is a major concern for cyclotron installation. Whereas, in high-energy cyclotrons, shielding is achieved mostly by thick concrete walls and with large separation between the walls and the cyclotron, medical cyclotrons are mostly self-shielded with lead blocks because of their compact nature. The shielding commonly consists of four lead block quadrants supported on casters that wheel in and out for closing and opening of the quadrants for easy access to the cyclotron for maintenance. The operation of a medical cyclotron is a turnkey type and computer controlled, and a technologist with appropriate training can operate it without difficulty. A typical medical cyclotron is shown in Fig. 7.2. The cyclotrons from different manufacturers are listed in Table 7.1.
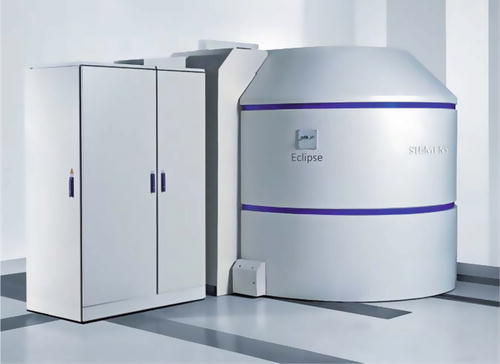
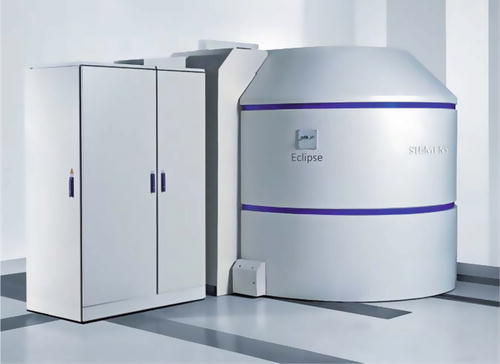
Fig. 7.2
A commercial cyclotron, ECLIPSE HD, manufactured by Siemens Medical Solutions USA (Courtesy of Siemens Medical Solutions USA, Inc.). The features of this cyclotron are given in Table 7.1
Table 7.1
Features of medical cyclotrons from different manufacturers
Company | Model | Beam type | Proton particle energy (MeV) | Proton beam current (μA) | No. of target positions | Simultaneous irradiation of targets |
---|---|---|---|---|---|---|
IBA | Cyclone 11 Cyclone 18/9 | H− H−/d− | 11 18/9 | 60 80/35 | 8 8 | 2 2 |
Siemens | Eclipse | H− | 11 | 60/40 | 4 | 2 |
GE | PETtrace 840 Minitrace | H−/d− H− | 16.5 9.6 | 75 60 | 8 8 | 2 2 |
Advanced Cyclotron Systems | TR 30 TR 14–19 | H−/d− H−/d− | 15–30 14–19 | 1000 300 | 8 16 | 2 2 |
Best Cyclotron Systems, Inc. | BCSI 15p | H− | 15 | 400 | 4 | 2 |
Sumitomo | Cypris HM-12 | H−/d− | 12 | 90 | 8 | 2 |
Nuclear Reaction
When targets of stable elements are irradiated by placing them in the external beam or in the internal beam of accelerated particles at a given radius inside a cyclotron, the particles interact with the target nuclei and nuclear reactions take place. Depending on the kinetic energy, the incident particle may be completely absorbed, depositing all its energy, or may leave the nucleus after interaction with one or more nucleons, leaving part of its energy. Nuclear reactions involving very high-energy particles are called spallations, in which many nucleons are ejected from the nucleus by the direct interaction of the incident particle. In either case, an excited nucleus is formed, and the excitation energy is disposed of by the emission of protons and neutrons, provided it is energetically allowed. Particle emission is followed by a cascade of γ-ray emissions when the former is no longer energetically possible. Depending on the energy deposited, several nucleons may be emitted resulting in the production of different radionuclides. The larger the energy deposited, the more particles are emitted, and a variety of radionuclides are produced. A simple nuclear reaction induced by a proton p on a target Z A X can be given by
where n is the neutron emitted and
is the radionuclide formed.


After irradiation, the target is transferred to a specially constructed containment unit called “hot cell” by a pneumatic tube system. The hot cell is built with thick lead wall encased in stainless steel sheets for radiation protection. It has a lead glass window to view inside and is equipped with two robotic arms, also termed manipulators, to maneuver the different operations such as transferring, pouring, lifting, heating, etc., of containers and materials inside the hot cell. It is exhausted through a HEPA filter for clean air environment to comply with good manufacturing practice (GMP) and kept under negative air pressure. The hot cell is equipped with water supply, purified air, lighting, electrical outlets, and other gases needed for various chemical procedures. A typical hot cell is shown in Fig. 7.3. Solid targets are dissolved in an appropriate solvent and radionuclides are separated from the target material by appropriate chemical methods such as solvent extraction, precipitation, chromatography, ion exchange, and distillation. Liquid and gas targets are specially designed, and appropriate methods are adopted to separate radionuclides. A dose calibrator is installed in the hot cell to measure the radioactivity produced.
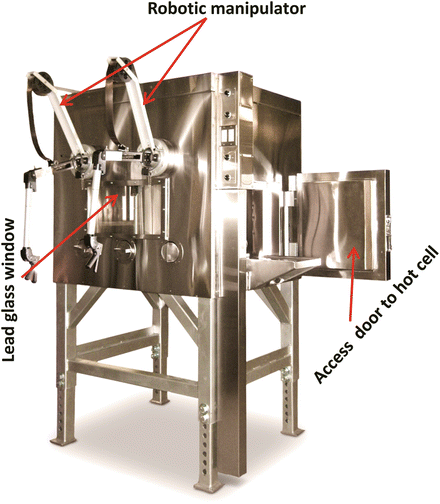
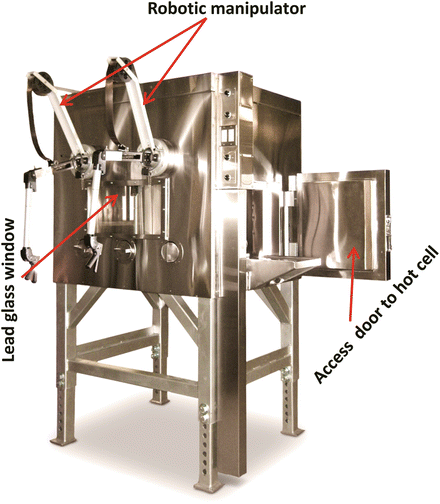
Fig. 7.3
A commercial hot cell whose features are described in the text. Red arrows indicate the robotic manipulators, the lead glass window, and the door to access the hot cell (picture provided by Radiation Shielding, Inc.)
Whereas the synthesis of some radiopharmaceuticals is carried out in the hot cell, many radiopharmaceuticals are synthesized in a step-down compact unit called minicell that is a smaller version of a hot cell and is commonly used for handling low level of activity (Fig. 7.4). A synthesis box of radiopharmaceuticals is placed inside the minicell, which is kept in GMP compliance and negative pressure. Water supply, air, exhaust, lighting, electrical outlets, and other essential accessories are provided in the mincell. In many cases, the minicell is seamlessly attached next to the hot cell and the separated radionuclide is transferred from the hot cell to the minicell by remote control via a side door. The features of a commercial synthesis box are described in Chap. 8.
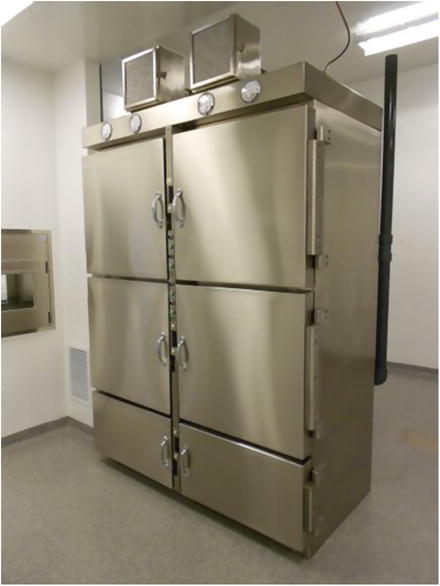
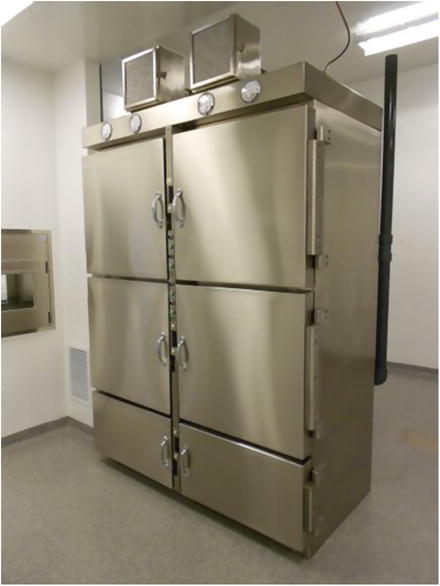
Fig. 7.4
Four commercial minicells, inside each of which a synthesis box for synthesis of PET radiopharmaceuticals is installed (picture provided by Radiation Shielding, Inc.)
Cyclotron-produced radionuclides are typically proton rich, i.e., neutron deficient and, therefore, decay by β + emission or electron capture. Also, the radionuclides, which are different from the target nuclides, do not contain any stable (or “cold”) atoms and are called carrier-free. Another term for these preparations is no-carrier-added (NCA), because no cold atoms have been intentionally added to the preparations.
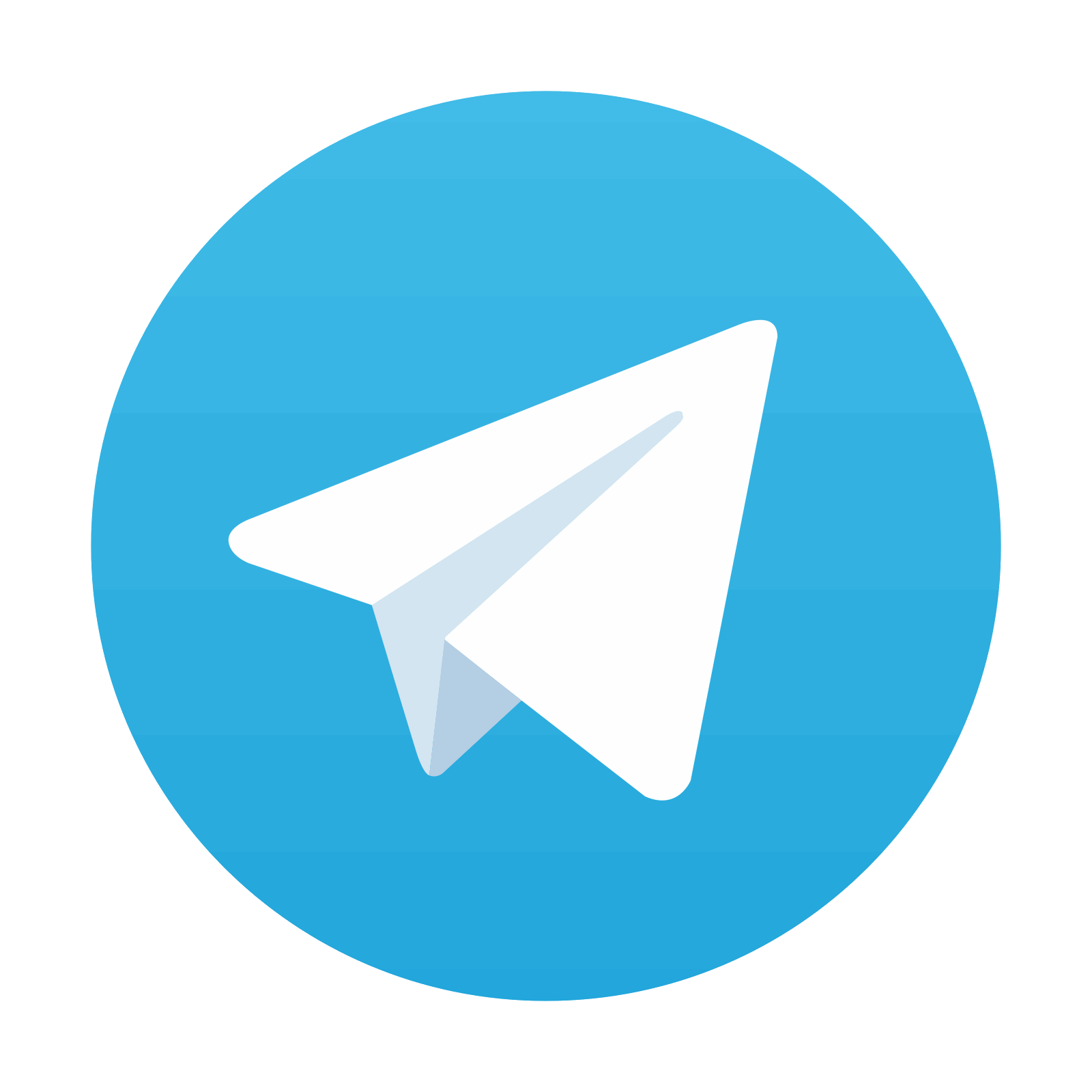
Stay updated, free articles. Join our Telegram channel

Full access? Get Clinical Tree
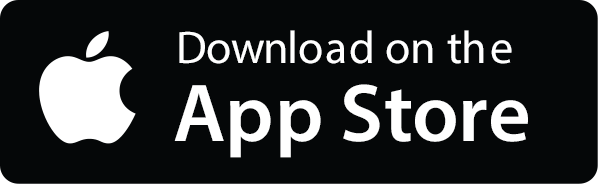
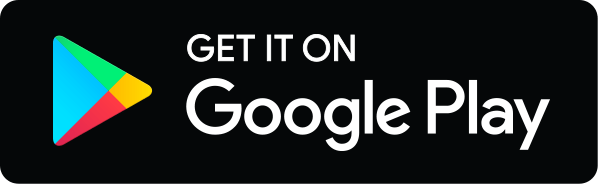