Introduction
Acute and chronic low back pain is a ubiquitous complaint encountered by primary care physicians throughout the United States, accounting for the second most common symptomatic reason for office visits. Low back pain is the leading cause of disability for patients younger than age 45, resulting in lost time from work and decreased work productivity, with increasing cost expenditures for evaluation and treatment of back pain estimated at $90 billion annually ($4695 per year in 1997 vs. $6096 in 2005); it is thus considered a major public health issue in the United States.
The most common reason why patients undergo imaging of the spine is pain. Degenerative/acquired and congenital spinal stenosis, neoplasm, infection, trauma, inflammatory or arthritic processes are all known causes of back pain. However, a majority of patients presenting with neurogenic intermittent claudication and radiculopathy is the result of acquired “degenerative” spondylotic changes as the cause of central canal, subarticular recess, and/or neuroforaminal compromise. Acquired degenerative disease is due to a combination of bone, ligament, joint, and disk disease, most commonly affecting the lumbar spine, followed by the cervical spine. Thoracic disk disease is now more commonly recognized with the increased use of magnetic resonance imaging (MRI).
Imaging Guidelines, Techniques, and Methods
Advanced imaging has led to a more accurate understanding of radiographic changes throughout the spine that are now known to occur as part of the normal aging process. However, imaging does allow for accurate assessment of mechanical causes of nerve root compression, offering objective evidence in support of a clinical diagnosis of back pain or radicular symptoms. Conversely there is a high prevalence of abnormal computed tomography (CT) and MRI findings in asymptomatic patients. Degenerative changes involving the intervertebral disks or facet joints have not been proven to correlate with back pain, because many patients without back pain demonstrate zygapophyseal joint osteoarthrosis, disk desiccation, reduced disk height, annular tears, and disk protrusions. Thus degenerative changes may be coincidental, given the poor association between low back pain and anatomic findings, and when found at imaging are not correlative of a patient’s pain or predictive for the development of back pain.
The use of advanced spine imaging has continued to rise rapidly, with MRI often being performed on patients not having received a trial of conservative therapy as currently recommended. The natural history of disk herniation is spontaneous regression, with extrusions and sequestrations demonstrating a higher trend toward resolution. Although multiple sources have confirmed that uncomplicated acute low back pain and/or radiculopathy is a benign self-limited condition that does not warrant imaging, many patients continue to undergo radiographic or advanced imaging evaluations without a clear indication. Improvement in pain and functional status usually occurs in most patients presenting with acute back pain (with or without radiculopathy) within 4 weeks regardless of treatment. It has been shown that routine imaging does not improve clinical outcomes, may expose patients to unnecessary radiation and invasive procedures, and contributes to rising healthcare costs. Owing to the ever-increasing trend in spine imaging and the associated expenditures, multiple medical professional organizations have issued recommendations and practice guidelines to address the overutilization of spine imaging.
Updated in 2015, the American College of Radiology (ACR) published appropriateness criteria for diagnostic imaging in patients with low back pain presenting with various scenarios, which were in parallel with previous guidelines from the American College of Physicians and the American Pain Society. In summary, the ACR appropriateness criteria recommend lumbar radiography as the initial evaluation for low back pain in patients with recent trauma, osteoporosis, on chronic steroids, patients suspected of vertebral compression fracture, and in the evaluation of young patients for ankylosing spondylitis. However, low back pain complicated by “red flags” may justify the use of CT or MRI. In the setting of major trauma, patients with neurologic compromise, history of malignancy, and patients at risk for vertebral infection for which clinical decision making may be directly influenced, advanced imaging should be performed. Furthermore, for low back pain complicated by radicular symptoms lasting longer than 6 weeks following a trial of conservative therapy, MRI should be performed, especially in patients who may be candidates for surgical intervention or percutaneous epidural steroid injection. As can be expected, the challenge for the referring physician is to distinguish this smaller group of patients who may require further workup and advanced imaging because of suspicion of a more serious underlying process.
Multiple imaging modalities have been used in the evaluation of degenerative disk disease, including radiography, diskography, CT, myelography with postmyelographic CT, MRI, and scintigraphy, which contributes to the dilemma of their application.
Radiography
As mentioned, radiographs may provide sufficient data in the initial evaluation of low back pain in patients with recent trauma, osteoporosis, or age older than 70. They provide an inexpensive screening tool of the spine, including osseous detail, alignment, stability, and postoperative evaluation of hardware and fusion. Most patients experience symptoms that occur in axially loaded positions such as standing or sitting; however, advanced imaging studies performed in the supine position do not reproduce the physiologic changes of weight bearing. Standing or upright frontal and lateral lumbar spine radiographs are an appropriate initial imaging study in patients with back pain, with dynamic imaging reserved for the presurgical setting.
CT/CT Myelography
The advancement of multidetector (MD)CT has allowed for rapid acquisition, with datasets reconstructed into multiple planes without the loss of spatial resolution or additional radiation exposure. CT imaging provides superior detail of cortical and trabecular bone compared with MRI. Similarly, MDCT can demonstrate ligamentous ossification, spondylosis, spondylolysis, facet joint arthropathy with encroaching osteophytes, and fracture. Although CT can accurately distinguish disk herniations, CT has been shown to be less reliable than MRI in detecting nerve compressive lesions. Although minimally invasive, CT myelography is recommended in patients with contraindications to MRI or in the postoperative spine with preexisting hardware. In patients with cervical radicular symptoms, CT myelography may be critical in determining osseous versus diskogenic impingement, as well as show lateral recess lesions that are less conspicuous on MRI. Furthermore an ACR expert panel on chronic neck pain recommends CT myelography as a viable alternative to MRI for patients with suspected cord involvement when MRI cannot be performed.
MRI
The superior soft tissue contrast resolution of MRI has allowed for noninvasive detailed anatomic evaluation of the spine without radiation exposure and is considered the imaging modality of choice for detection of an objective cause of radicular symptoms versus normal age-related changes. Application of different pulse sequences allows for image acquisition to exploit tissue composition. T1-weighted sequences provide optimal contrast between bright epidural fat and the internal components of the spinal canal. Osseous anatomy can also be evaluated using T1 sequences, owing to the abundance of fat within the vertebral body marrow. Accordingly an infiltrative or marrow-replacing process, as well as focal bone lesions, can be detected as hypointense signal on T1-weighted images. The intervertebral disks are slightly lower signal than the adjacent vertebral bodies. Cerebrospinal fluid (CSF) is lower signal on T1-weighted images than the relatively higher-signal spinal cord, nerve roots, and peripheral epidural fat. The exiting nerve roots and dorsal root ganglia are also surrounded by epidural fat.
In general, T2-weighted images have greater contrast differentiation compared to T1-weighted images. For example, the cortical bone of the vertebral bodies demonstrates hypointense T2 signal, whereas the vertebral bone marrow remains higher signal owing to its rich fat content. The nucleus pulposus is made of water-binding glycosaminoglycans, thus demonstrating intermediate T1 and hyperintense T2 signal. The anulus fibrosus, which is the less-hydrated fibrous capsule surrounding the nucleus pulposus, demonstrates hypointense signal on all pulse sequences. CSF demonstrates high T2 signal, allowing for more sensitive identification of the intermediate-signal spinal cord and nerve roots. Fat-suppression techniques or the application of short tau inversion recovery (STIR) sequences allows for more conspicuous detection of marrow edema. Fluid-weighted sequences are thus useful for visualizing disk desiccation, high-intensity zones, and Modic end-plate changes. In the cervical spine, conventional spin echo imaging poorly differentiates cortical osteophytes from disk material. Gradient echo sequences allow for delineation of bone and disk margins, providing excellent contrast between the spinal cord and surrounding subarachnoid space, as well as clear visualization of the exiting cervical nerve roots.
Lumbar Spine
Anatomy
The changes that compromise the central canal and neuroforamen are directly related to the three-joint functional unit of the spine allowing motion: the end plate–intervertebral disk–end plate complex and the paired zygapophyseal (facet) joints. A review of the lumbar diskovertebral complex as they relate to acquired degenerative disease is useful. The anterior column consists of the anterior portion of the vertebral body, intervertebral disk, and anterior longitudinal ligament. The middle column consists of the posterior half of the vertebral body, intervertebral disk, and posterior longitudinal ligament. The posterior column includes the pedicles, facet joints, laminae, spinous processes, ligamentum flavum, and interspinous ligaments ( Fig. 29-1 ). The spinal canal is formed by the posterior cortex of the vertebral body anteriorly, pedicles laterally, laminae posterolaterally, and the base of the spinous processes posteriorly, forming a protective ring surrounding the neural elements.
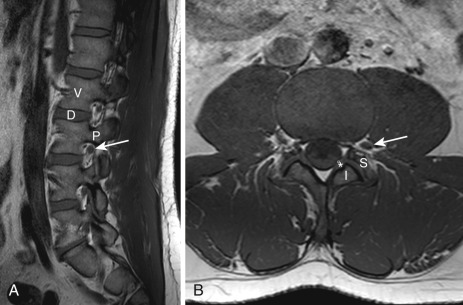
Each spinal nerve exists within intervertebral neural foramen, bordered superiorly and inferiorly by adjacent pedicles, anteriorly by the intervertebral disk and vertebral body, and posteriorly by the facet joints. When viewed sagittally, the foramina should have a vertical oval appearance, with the exiting nerve roots passing through the upper portions just posterior to the diskovertebral complex. All lumbosacral nerve roots originate at approximately the T12-L1 level where the spinal cord terminates as the conus medullaris. The nerve roots course inferiorly through the spinal canal, forming the cauda equina, with the most laterally positioned roots leaving the bundle until they exit through their respective neural foramen. The exiting nerve roots carry the same number designation as the adjacent pedicle they travel beneath (e.g., the L5 nerve root exits under the L5 pedicle). The lateral recesses, which are located on the anteromedial aspects of the pedicles, contain the traversing nerve roots. The nerve roots travel within the lateral recesses after leaving the thecal sac but before entering the next exiting neural foramina. Epidural fat concentrically surrounds the thecal sac, which encases the exiting spinal nerves and fills the neural foramina ( Figs. 29-2 and 29-3 ).
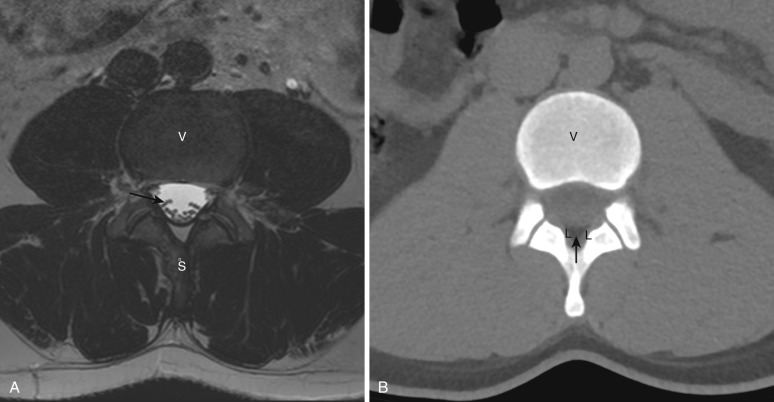
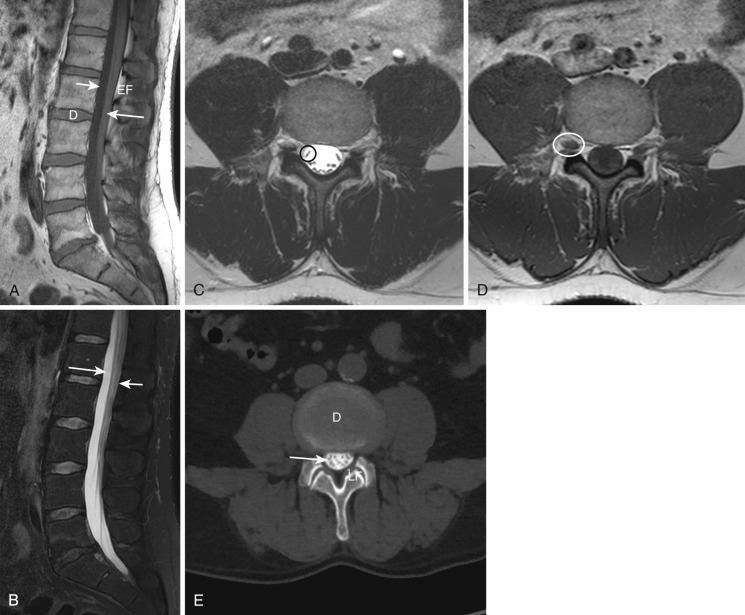
The superior articular process, which is directed posteromedially, and the inferior articular process, which is directed anterolaterally, extend cranially and caudally and are joined by the pars interarticularis. The lumbar facet articulations are true synovial joints lined by hyaline cartilage, innervated by medial branches of the primary dorsal rami that arise from the spinal nerve just peripheral to the dorsal root ganglion. The ligamentum flavum, the interspinous and supraspinous ligaments, and the facet joint capsules constitute the posterior ligamentous complex. The ligamentum flavum bridges the interlaminar interval, attached to the facet joint capsules laterally and to the midline interspinous ligament medially, forming the posterior wall of the vertebral canal.
Imaging of Lumbar Degenerative Disk Disease
Pathophysiology.
The diskovertebral complex is a cartilaginous joint formed by the intervertebral disk components and the hyaline cartilage–lined vertebral end plates. Surrounding the inner nucleus pulposus is a fibrous capsule, the anulus fibrosus, which is attached to the vertebral apophyseal ring by Sharpey fibers and continuous with the anterior and posterior longitudinal ligaments. Water bound to proteoglycans comprises a majority of the inner nucleus pulposus, acting to disperse axial loads in a radial distribution. With aging, disk desiccation ensues as the proteoglycan concentration and water-binding capacity decreases, causing the nucleus to become more fibrous and less elastic, thus transferring axial loads to the posterior aspect of the anulus. As the anulus continues to stretch, fissures can develop, resulting in internal disk disruption, axial pain, and disk herniation. Excessive physical loading and stretching of the posterior anulus allows for mechanical stimulation of the pain receptors within the annular fibers. However, radiculopathy and radicular pain are the products of both mechanical nerve compression and an inflammatory response, likely mediated by tumor necrosis factor (TNF)-α, although other substances may play a role in the pain cascade. Evidence to support an inflammatory response as a component of radicular pain includes: (1) pain persists despite adequate surgical decompression, (2) large disk herniations may be asymptomatic, (3) size or shape of herniation has no significant correlation with severity of symptoms, and (3) conservative therapy is usually effective. Furthermore, the nucleus pulposus has been shown to induce an inflammatory response and nerve dysfunction, which is relieved by anti-inflammatory agents, but concomitant compression is necessary to cause pain.
Degenerative changes or degenerative disk disease applies to the process of intervertebral disk desiccation, manifesting as loss of the normal T2 signal within the nucleus, which may be an early sign of degeneration or reflect normal age-related changes. Spondylosis is often synonymous with disk disease but should be reserved as a descriptor for marginal anterior and lateral osteophyte formation without substantial disk height loss. Osseous remodeling can occur in a relatively normal joint that is exposed to excessive external loads, or as a response to the nucleus pulposus matrix degradation. In a study by Nathan, anterior and lateral osteophytes were identified in all cadavers older than age 40, and posterior osteophytes were present in a majority of specimens older than age 80. However, failure of the nucleus sets in motion a cascade of events termed intervertebral osteochondrosis, manifesting as decreased disk space height, posterior osteophytes, radial annular fissures, end-plate erosion and marrow changes, and nitrogen gas within the nucleus, which are pathologic processes that are not features of normal aging. Osteophytes are formed as attempts to increase the surface area of the diskovertebral complex by creating additional articular surfaces to reduce or redistribute the axial loading compressive forces throughout the joint. With loss of disk height and compressive stress redistribution across the joint, load-bearing capacity is shifted toward the posterior column. The facet joints endure increased stresses, leading to thinning and erosion of the articular cartilage, joint space narrowing, subchondral sclerosis, osteophyte formation, synovitis, and synovial cyst formation. Facet hypertrophy and osteophyte formation primarily arising from the superior articular facet can result in central canal stenosis as well as lateral recess and neural foraminal narrowing with subsequent compromise of traversing or exiting nerve roots. Disk degeneration with height loss and facet joint osteoarthrosis causes increased stress on the posterior ligamentous structures, resulting in ligamentum flavum hypertrophy, laxity, and anterior buckling and thus spinal stenosis ( Fig. 29-4A1-A3 ). Increased stresses also result in degeneration of the interspinous process ligaments, leading to close apposition and hypertrophy of the spinous processes (“kissing”), causing flattening or a grooved appearance, subchondral sclerosis, and cystic degeneration and interspinous bursa formation (see Fig. 29-6B1-B4 ). Moreover, spondylolisthesis or subluxation of the facet joints allows for vertebral body motion or slippage, most commonly at L4-L5, causing progressive central canal or neural foraminal narrowing (see Fig. 29-4B1-B4 ). The dynamic changes that occur in the anterior and posterior columns of the spine in combination contribute to narrowing of the central canal, lateral recesses, and neural foramen.
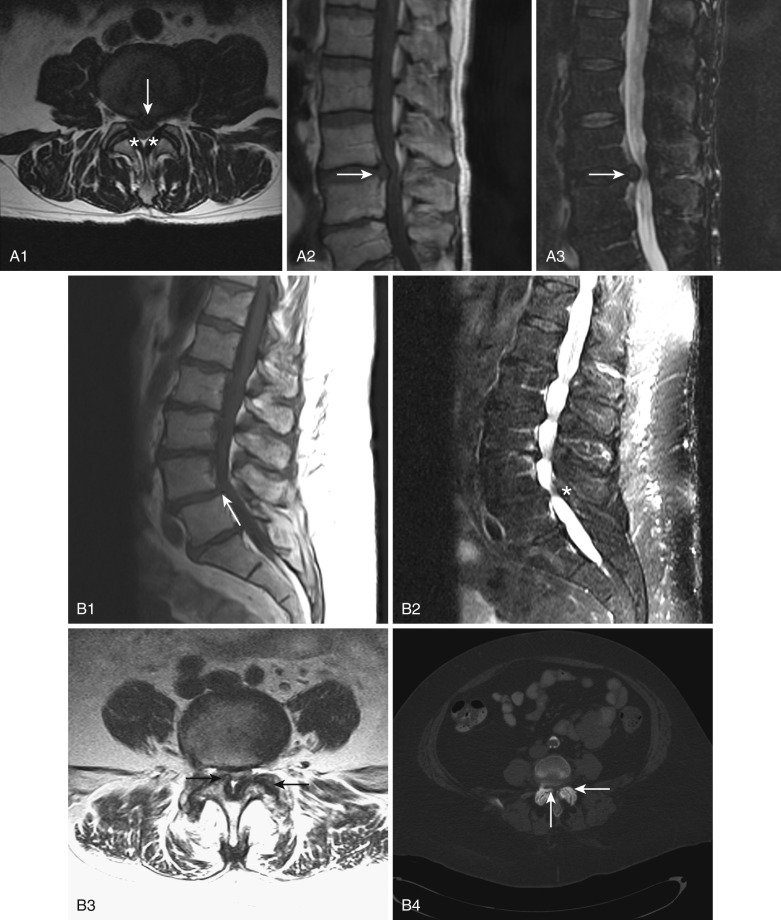
Modic Changes.
Signal changes that occur within the subchondral bone along the vertebral end plates in response to continued nuclear matrix degradation and end-plate articular cartilage disruption have correlated with histologic findings. Originally described by Modic and colleagues, the spectrum of subchondral vertebral marrow changes in association with degenerative disk disease has been classified into three types. Type I change is thought to represent an active inflammatory state, type II a more dormant state, and type III a postinflammatory state. However, it is not uncommon to visualize mixed I/II changes, suggesting that these changes can convert between types. Modic II changes are the most common, followed by type I and mixed type I/II.
Type I changes show subchondral bone marrow edema, as demonstrated by decreased signal intensity on T1-weighted images and increased signal intensity on T2-weighted images ( Fig. 29-5A1-A3 ). Histologically the end plates are disrupted and fissured, with the subchondral marrow infiltrated by fibrovascular granulation tissue, and thus exhibit enhancement on postcontrast images. Patients with Modic I changes have been documented to have high levels of C-reactive protein, which may support the presence of a local inflammatory response within the vertebral end plate. Furthermore, elevated levels of immunoreactive nerve fibers and TNF-immunoreactive cells were significantly higher in end plates demonstrating Modic I changes in comparison with patients with normal end plates on MRI or in end plates exhibiting Modic type II changes. Luoma et al. documented accelerated diskovertebral degenerative change in patients with Modic I changes, which supports a distinct pathologic process at the level of the diskovertebral unit. Overall, Modic I change has a stronger association with low back pain than type II changes.
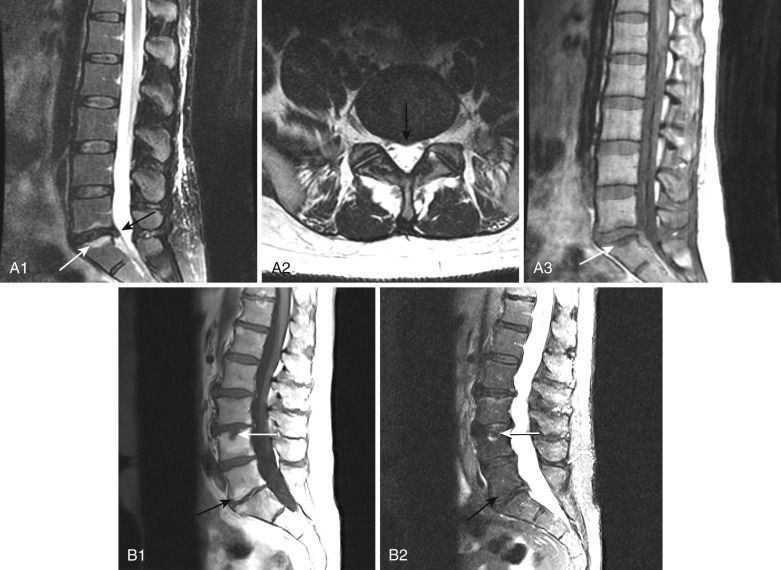
Type II changes are characterized by increased signal intensity on T1- and T2-weighted images, correlating to yellow or fatty marrow replacement within the subjacent vertebral body end plates (see Fig. 29-5B1-B2 ).
Type III changes are characterized by decreased signal intensity on both T1- and T2-weighted sequences, correlating with subchondral end-plate sclerosis.
High-Intensity Zones.
Initially described by April and Bogduk, a high-intensity zone (or annular fissure/tear) on MRI indicates internal disk disruption and the presence of trapped nuclear material within the periphery of the anulus fibrosus, correlating with grade IV annular fissuring at postdiskography CT and reproducible pain during provocative diskography. The trapped nuclear material demonstrates hyperintense T2 signal greater than that of the parent nucleus, as well as enhancement on postgadolinium images, secondary to the localized inflammatory process ( Fig. 29-6A1-A4 ). Although there is agreement that a high-intensity zone is a marker for a posterior annular fissure, there is variable disagreement as to the significance of high-intensity zones identified on MRI as an indicator of low back pain, because they are not always associated with reproducible pain response at diskography. However, it has been shown that annular tears exhibiting higher levels of T2 signal have greater specificity and stronger positive predictive value for pain production.
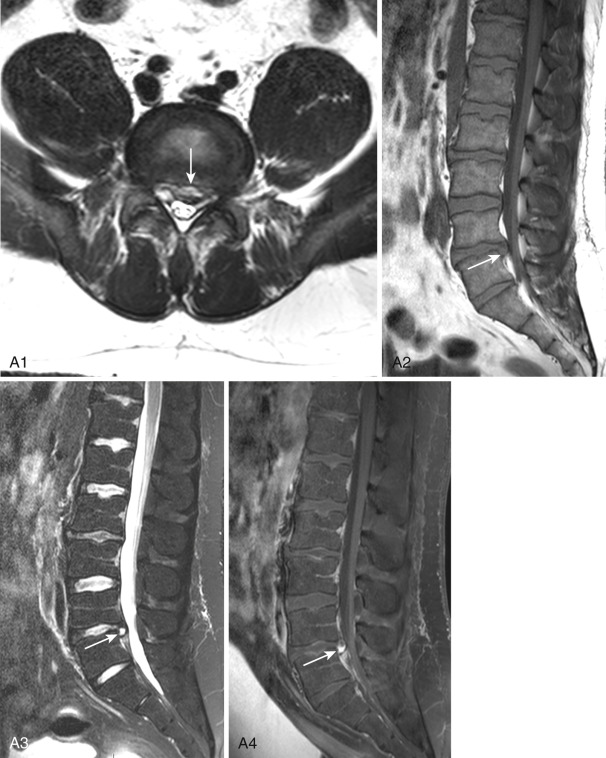
