- •
Be familiar with the anatomy of the normal aorta and branch vessels
- •
Appreciate that the comprehensive evaluation of the aorta includes examination of the heart for other comorbid conditions
- •
Identify and describe major pathologic findings of diseases of the aorta on CMR/MRA studies
- •
Understand the challenges of contrast-enhanced magnetic resonance angiography
Cardiovascular magnetic resonance (CMR) is recognized as a useful tool for the noninvasive evaluation of the aorta by providing clinicians with high quality diagnostic images, in any imaging plane, without interference from surrounding soft tissue or bone, using only a peripheral IV catheter and administration of CMR contrast agents characterized by a favorable safety profile. Recent technological advances in magnet design allow for short acquisition times, high signal-to-noise and contrast-to-noise ratios, and improved spatial and temporal resolution. With images resembling conventional x-ray angiography, contrast-enhanced MRA (CE-MRA) is the preferred currently available MRA technique used today. This technique does not rely on the flow characteristic of blood but rather on T1-shortening effects of a circulating CMR contrast agent, making it less prone to flow-related artifacts. Successful CE-MRA requires the coordination of an intravenously administered CMR contrast agent bolus with image acquisition during the arterial phase of the first-pass transit of the CMR contrast agent. With a short acquisition time, the scan can be completed before venous enhancement occurs. For vascular applications, CE-MRA can depict often complex and entwined vascular anatomy because of the free choice of imaging planes by the scan operator and the acquisition of 3D data sets that overcome many limitations of projection techniques. CE-MRA contains little information about the morphology of the aortic wall, and should be complemented by T1- or T2-weighted precontrast and, in some cases, post-contrast images.
One of the strengths of CMR is the ability to obtain dynamic information regarding blood flow and heart function. A cardiac cine CMR study (whether complete or abbreviated) should be performed before CE-MRA to examine left ventricular cavity size, mass, and global and segmental function as clues of other comorbid conditions. Cine CMR is also invaluable for determining aortic valve morphology (such as bicuspid or calcific valves) and, in cases of aortic dissection and aneurysm, the mechanism of aortic regurgitation (e.g., dilatation of the aortic root and annulus, tearing of the annulus or valve cusps, downward displacement of one cusp below the line of valve closure, loss of support of the cusp, and physical interference in the closure of the aortic valve by an intimal flap). Phase contrast methods through the aortic root can be used to calculate the regurgitant fraction or estimate aortic stenosis severity by measuring peak transaortic velocity.
Unlike CT angiography or conventional x-ray angiography, CMR is not associated with concerns related to ionizing radiation exposure (whether to the patient, operator, or staff), or to the unfavorable side effect profile of iodinated contrast (including nephrotoxicity, anaphylactoid allergic response, and adverse hemodynamic effects of lowering blood pressure, increasing left ventricular end diastolic pressure, and depressing myocardial contractility). X-ray angiography carries the risks associated with arterial puncture, vascular injury in patients with difficult vascular access, hemorrhage, and catheter-induced thromboembolic or atheroembolic ischemic complications. There is no aftercare of the patient following a CMR examination, and compared with conventional x-ray angiography, it is virtually painless.
NORMAL ANATOMY OF THE AORTA AND BRANCH VESSELS
In order to cover large vascular territories, CE-MRA can be performed using several overlapping contiguous CE-MRA acquisitions, so-called multistation MRA. In Figure 21-1 , the initial station is centered on the neck and the first scan is initiated with arrival of gadolinium in the aortic arch. Each subsequent MRA station is acquired in rapid succession moving caudally to capture the arterial transit of gadolinium down the aorta. A stepping table is essential to perform this “bolus chase” and consists of the progressive movement of the imaging field of view to coincide with gadolinium run-off. The main challenge of multistation MRA is to image quickly to avoid enhancement in the venous vessels, which overlap arterial vessels. The ascending aorta takes its origin from the left ventricle. At its beginning, the aortic root comprises the aortic annulus and the sinuses of Valsalva (three localized dilatations or sinuses), and from the right and left sinuses arise the right and left coronary arteries. The sinuses lie above and behind the aortic semilunar valve leaflets. The sinuses join the tubular ascending aorta at the sinotubular junction, and from there the aorta is directed upward and forward. The ascending aorta is mobile for the most part and is enclosed by the pericardium up to the level of the sternal angle (angle of Louis). The aortic arch is the continuation of the ascending aorta and emerges from the pericardium traveling first upward, then backward and to the left, before turning downward just to the left side of the fourth thoracic vertebra. Aortae that cross over the left pulmonary artery and pass left of the trachea are termed left-sided, and right-sided when crossing over the right pulmonary artery and to the right of the trachea. For the most part, adult patients will have normal ventriculoarterial concordance and left-sided aortae. In all forms of aortic disease, it is important to identify the origins of the branch vessels. Branch ostia may originate from the body of the aneurysm or from the false lumen in cases of dissection. It is important to identify the branch vessel origins in these cases because a complete surgical repair will be determined in part by this information. Branches of the aortic arch are the innominate artery (or brachiocephalic trunk), left common carotid artery, and the left subclavian artery. This pattern is found in about 65% to 70% of people. Attached to the underside of the arch opposite the origin of the left subclavian artery and directed toward the root of the left pulmonary artery is the ligamentum arteriosum , which is a band of connective tissue about 3 to 5 mm in diameter and represents the remains of the fetal ductus arteriosus . Here a focal narrowing of the lumen of the aorta may be found called the aortic isthmus. The aorta is fixed to the thorax at this point. The descending aorta then travels caudally to the left of the spine until it penetrates the diaphragm at the aortic hiatus near the level of the twelfth thoracic vertebra. Below the level of the diaphragm, the aorta becomes the abdominal aorta and ends by dividing into the two common iliac arteries at the bifurcation. Three ventral unpaired branches of larger size supply the gastrointestinal tract: the celiac trunk, the superior mesenteric artery, and the inferior mesenteric artery. The celiac trunk arises from the front of the aorta just below the aortic hiatus. The classic anatomic branches of the celiac trunk (occurring in about 50% to 60% of patients) are the splenic, left gastric, and common hepatic arteries. The superior mesenteric artery (SMA) is the second of the unpaired branches of the aorta, originating caudal to the celiac trunk, and supplies branches to the pancreas, all of the small bowel (except the proximal duodenum), and the right and mid colon. The renal arteries arise one on each side of the aorta at the upper level of the second lumbar vertebra about 1 cm below the SMA. They pass transversely to the hilum of each kidney where they divide typically into three branches that then break into numerous secondary branches. Multiple renal arteries are present in 30% of the population, usually arising as accessory renal arteries from the aorta. Left accessory renal arteries are encountered more often than right sided ones. As a rule, aortic origins of accessory renal arteries occur caudal to the main renal artery. The abdominal aorta bifurcates into the right and left common iliac arteries at the level of the umbilicus, typically near the level of the fourth lumbar vertebra. Atherosclerosis commonly first involves the arteries of the lower extremities at or near the aortic bifurcation. Normal aortic dimensions are listed in Table 21-1 .
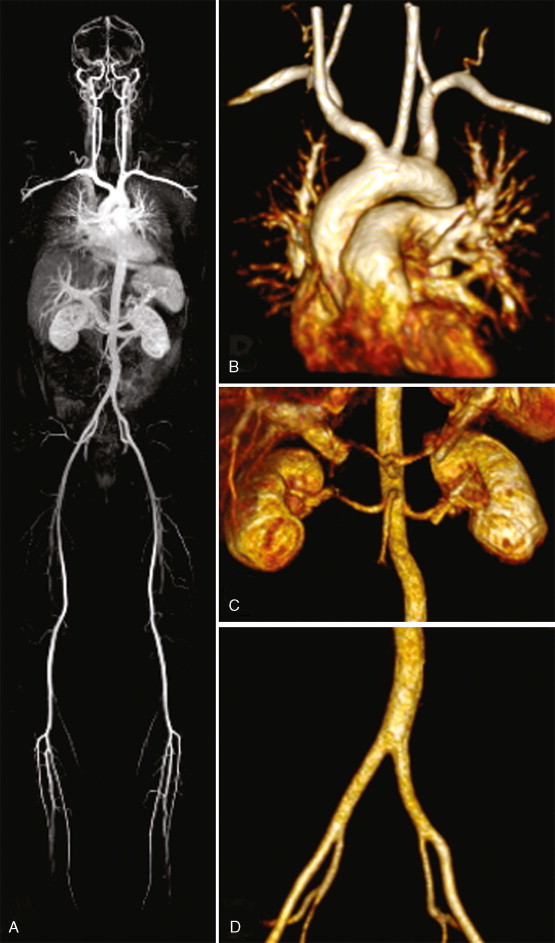
MEN | WOMEN | |
---|---|---|
Aortic annulus | 26 ± 3 mm | 23 ± 2 mm |
Aortic root | 31 ± 5 mm | 28 ± 3 mm |
Ascending aorta | 34 ± 6 mm | 28 ± 4 mm |
Descending aorta | 25 ± 4 mm | 20 ± 4 mm |
A common anatomic variation of the arch vessels is the so-called “bovine” arch where the innominate and left common carotid arteries share a common origin ( Figure 21-2 ). In this example the left common carotid artery originates off the innominate artery, the second arch vessel is the left vertebral artery, and the third arch vessel is the left subclavian artery. This branching pattern is found in about 25% to 27% of people, and interestingly, in most apes. Less common are four separate ostia for each of the arch vessels (2.5%) and the “avian” arch characterized by only two branches, or bi-innominate anatomy (1.2%).
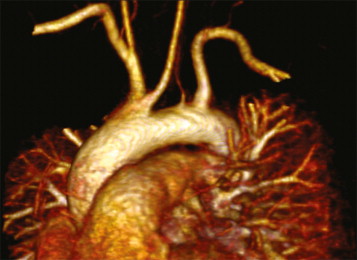
AORTIC ANEURYSM
A 50-year-old man with hypertension treated with an ACE inhibitor and diuretic presented with mild chest pain across the mid chest. ECG demonstrated sinus bradycardia with voltage criteria for left ventricular hypertrophy, inferior T-wave inversion, and J-point and ST-segment elevation in leads V3-V4. Bright blood CMR images were taken ( Figure 21-3 ).
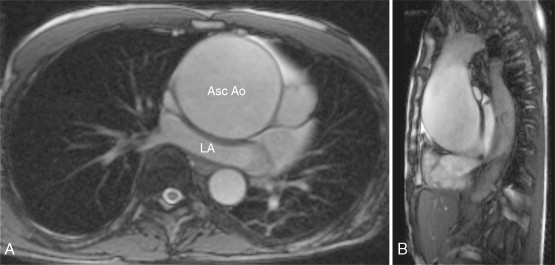
Aneurysms are areas of focal or diffuse dilatation of the aorta that develop at sites of medial weakness. The aneurysmal sack is composed of fibrous tissue. Aneurysms can be fusiform (uniform, symmetric, circumferential shape) or saccular (eccentric, focal, outpouching shape). The most common etiologies of aneurysmal development are atherosclerosis and hypertension. The atherosclerotic process is thought to erode the aortic wall and destroy the medial elastic elements that leads to weakening of the wall and dilatation; whereas hypertension imposes increase mechanical wall stress. Ascending thoracic aortic aneurysms can occur from Marfan’s syndrome (or other connective tissue diseases), longstanding aortic stenosis, and rarely, traumatic or mycotic etiologies.
For contrast-enhanced MRA (CE-MRA) to be successful, a high concentration of contrast agent (a chelate of gadoliunium) should be maintained in the aorta during the entire image acquisition time. Contrast volume, delivery rate, delay time from injection to image acquisition, and saline flush are important for good image quality. To make blood appear bright compared with the background tissues, it is necessary to inject a sufficient dosage (or volume) of gadolinium at a sufficient rate.
The arterial phase of the gadolinium bolus injection is the optimal time to acquire MRA data to take advantage of the higher arterial SNR and to eliminate overlapping venous enhancement. An extremely fast MRA acquisition is essential to capture the gadolinium bolus during the brief moment that the agent is present in the aorta but not yet in the venous system. Circulation times are variable from patient to patient because of differences in cardiac output, blood flow profiles, and plasma volume. Coordination of image acquisition and the arrival of the gadolinium bolus in the aorta are crucial to achieve good image quality. Imaging prematurely can result in incomplete vascular depiction. Imaging too late can result in insufficient signal within the aorta (caused by washout of the gadolinium and diffusion into the tissue) and enhancement of the veins and soft tissues, which can obscure the aorta. Different strategies have been developed to optimize the proper timing of synchronizing arterial enhancement with image acquisition in order to avoid enhancement of any unwanted structures. A real time method of timing the bolus arrival is MR fluoroscopy ( Figure 21-4 ).
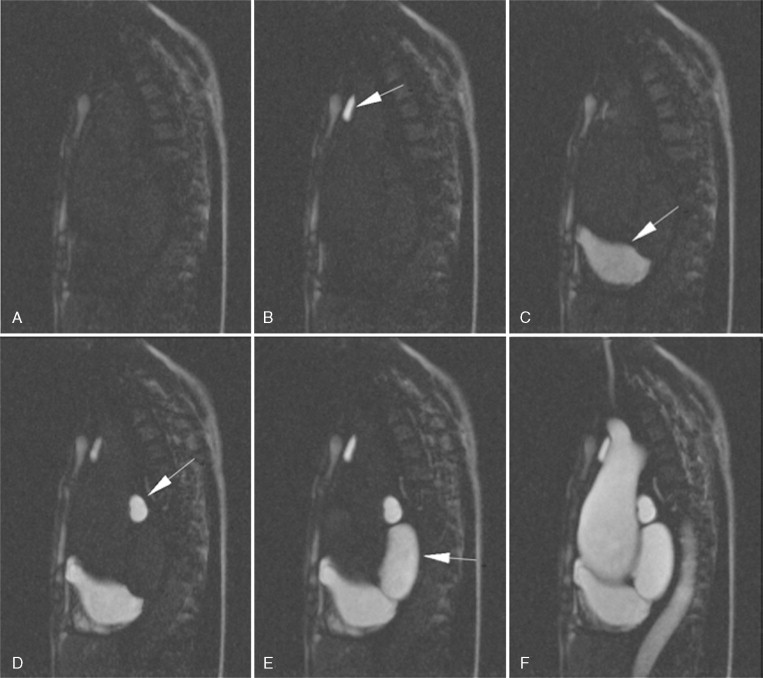
An aortic aneurysm can be described in regards to its site or location along with its morphology or shape (fusiform versus saccular). It may be helpful to reference the location to another anatomical landmark. Aortic dilatation may extend into branch vessels ( Figure 21-5 ).
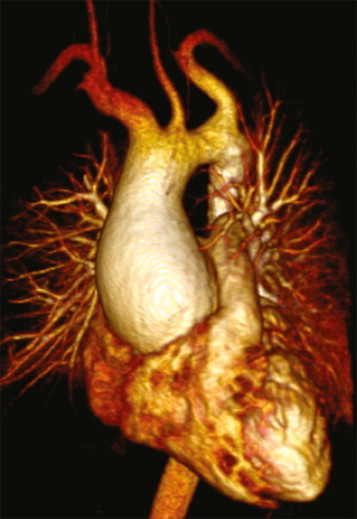
One of the strengths of CMR is the ability to obtain dynamic information regarding the blood flow and heart function. A complete CMR/MRA evaluation for aortic disease includes scans to evaluate not only the aorta and aortic lumen but also the heart. Aortic insufficiency is common with ascending aortic aneurysms ( Figures 21-6 and 21-7 ).
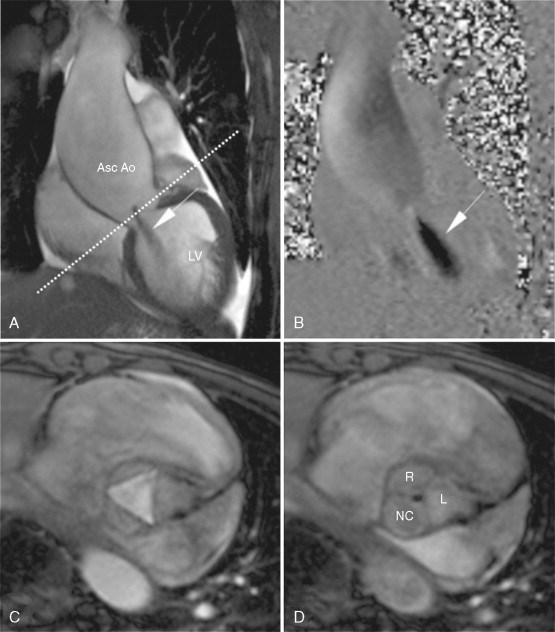
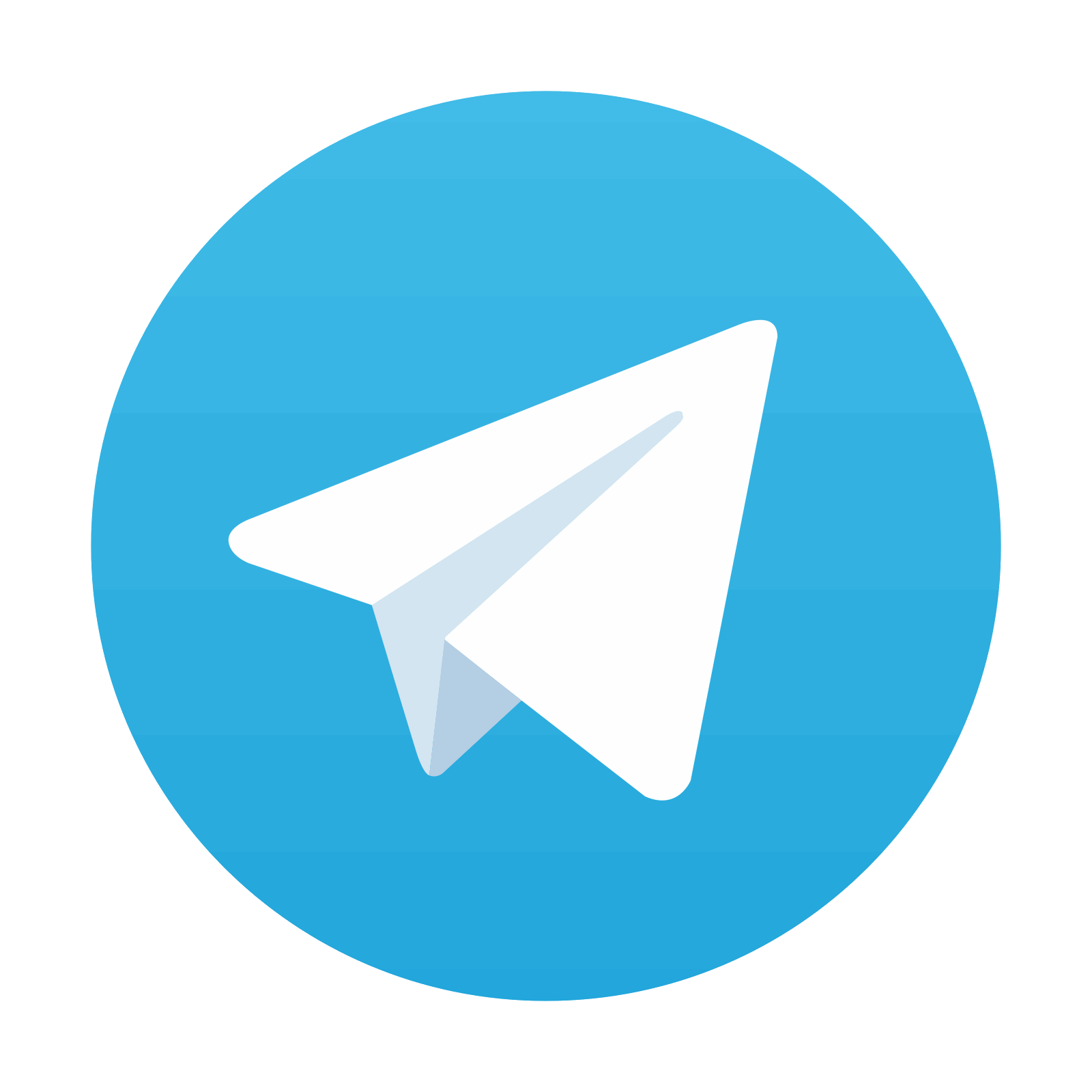
Stay updated, free articles. Join our Telegram channel

Full access? Get Clinical Tree
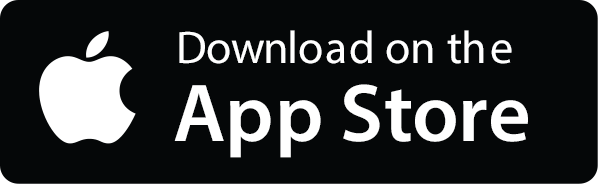
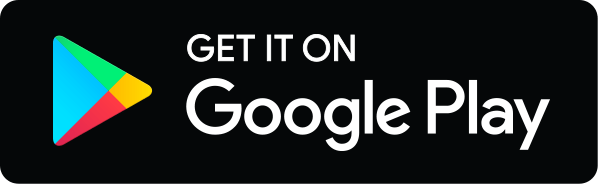
