Fig. 1
The chronology of pulmonary injury after HCT (high-dose chemotherapy)
Table 1
Pulmonary complications in bone marrow transplantation
Complications
|
Days after BMT
|
Incidence (%)
|
Pulmonary infiltrates
|
---|---|---|---|
Pulmonary edema
|
1–30
|
Unknown
|
Diffuse
|
DAH
|
1–30
|
5
|
Diffuse
|
Bacterial pneumonia
|
1–30
|
20–50
|
Focal
|
Aspergillus
|
1–30
|
20
|
Focal
|
HSV
|
1–30
|
5–7
|
Diffuse
|
CMV
|
31–100
|
10–40
|
Diffuse
|
IPS
|
31–100
|
10–17
|
Diffuse
|
PCP
|
31–100
|
<1
|
Diffuse
|
COP
|
≥31
|
1–2
|
Diffuse
|
Chronic GVHD
|
>100
|
20–45
|
No infiltrates
|
BO
|
>100
|
6–10
|
No infiltrates
|
DPTS
|
>100
|
Unknown
|
Diffuse
|
RLD
|
>100
|
<20
|
No infiltrates
|
PAP
|
>100
|
<5
|
Diffuse
|
Vasculopathy
|
>100
|
<5
|
No infiltrates
|
As mentioned previously, pulmonary complications are caused by a host of interrelated factors including cytotoxic agents. Numerous chemotherapy agents have direct pulmonary toxicity and inflict damage upon the lungs in the absence of any other contributing factors and are listed in Table 2. In addition, these agents frequently work synergistically with radiotherapy which causes even further pulmonary toxicity when used in combination with TBI. The pathological features of both drug- and radiation-induced pulmonary injury are alveolar septal thickening with interstitial fibrosis, the presence of atypical type 2 pulmonary cells, and endothelial damage (Gosselin and Adams 2002; Alam and Chan 1996). Drug-induced injury is manifest in a varied pattern including capillary leak pulmonary edema, a hypersensitivity reaction, diffuse alveolar damage, and a nonspecific interstitial pulmonary pneumonitis (Gosselin and Adams 2002; Wilczynski Stephen et al. 1998). Symptoms secondary to drug-induced injury are most likely to become apparent within the first 100 days from transplant. Bleomycin in particular, is a known pulmonary toxin which causes pulmonary fibrosis and its use either prior to or as part of the conditioning leads to a high rate of long-term lung damage (Hartsell et al. 1995).
Table 2
Parameters associated with pulmonary toxicity
Variable
|
Reduced incidence
|
Increased incidence
|
---|---|---|
TBI Total dose (higher)
|
X
|
|
Fractionation effect
|
X
|
X
|
STBI
|
||
FTBI
|
||
TBI dose rate
|
X
|
|
Chemotherapy
|
X
|
|
ACT-D
|
X
|
|
Dox
|
X
|
|
Bleo
|
X
|
|
BU
|
X
|
|
CY
|
X
|
|
BCNU
|
X
|
|
Interferons
|
X
|
|
MTX
|
X
|
|
ARA-C
|
X
|
There are several irradiation-related factors which have been shown to influence the incidence of late pulmonary toxicities in most clinical reports although controversies still exist as to their magnitude. These factors include total dose, fractionation schedule, dose rate, and use of lung shielding. One of the earliest clinical studies examining the relationship of total dose of irradiation and the incidence of pneumonitis was reported by Van Dyk and colleagues at the Princess Margaret Hospital. The incidence of IP was collected in 245 patients who had received various single whole lung doses for metastatic solid tumors. At doses below approximately 7.5 Gy, the incidence of pneumonitis was minimal. Above 7.5 Gy to approximately 11 Gy, the incidence rapidly increased to almost 100 % in a sigmoid fashion (Van Dyk et al. 1981). This corresponded well to studies subsequent to the Seattle report documenting that the incidence of IP for patients treated with a single fraction TBI preparative regimen ranged from approximately 20 to 50 % (Thomas et al. 1977; Hartsell et al. 1995; Van Dyk et al. 1981; Barrett et al. 1987; Cordonnier et al. 1986; Granena et al. 1993; Kader et al. 1994; Morgan et al. 1995; Weiner et al. 1986; Fryer et al. 1978). As animal models began to suggest that there was no deleterious effect of fractionation on marrow reconstitution (Deeg et al. 1988; Collis and Down 1984; Storb et al. 1999; Kolb et al. 1990), fractionated TBI began to be utilized in the clinical setting. There is now ample data that confirms the reduction in pulmonary and other late toxicities through the use of fractionated TBI (Leiper 2002a; Beddar et al. 1995; Forsl et al. 2006; Sampath et al. 2005; Savani et al. 2005; Shank et al. 1982, 1990; Hoffmeister et al. 2006; Gopal et al. 2001) to <15 % in most centers. Recent mathematical modeling derived from clinical data from human trials is consistent with this data. The model predicts that the incidence of radiation pneumonitis is <10 % at total lung doses of ≤15 Gy fractionated at 2 Gy per fraction in the absence of any other pulmonary insults (Semenenko and Li 2008). The dose rate at which TBI is administered also has an impact on the development of pulmonary toxicity with high dose (HD) rates leading to an increased incidence of pulmonary complications than lower dose rates (<approximately 15 cGy/min) in both animal models as well as clinical trials (Deeg et al. 1988; Yen et al. 2004; Gosselin and Adams 2002; Soubani et al. 1996; Afessa and Peters 2008; Alam and Chan 1996; Kader et al. 1994; Storb et al. 1999; Kolb et al. 1990). In an effort to further abrogate the toxicity of TBI, many centers employ lung shielding to reduce the total irradiation dose to the lungs (Sampath et al. 2005; Forslöw et al. 2006; Rutter and Chien 2007). The Seattle group showed that pulmonary function tests were better with the use of lung shielding (lung dose < approximately 9 Gy) versus no lung blocking at 100 days but not at 1 year (Rutter and Chien 2007). Sampath and colleagues demonstrated an incidence of interstitial pneumonitis of 11 % in unshielded patients treated to 12 Gy in 6 daily fractions versus 2.3 % when the lung dose was limited to approximately 6 Gy (Sampath et al. 2005). The rate of marrow engraftment was not different in lung blocked patients compared to patients whose lungs were not shielded in either of these studies (Sampath et al. 2005; Rutter and Chien 2007).
The incidence of pulmonary dysfunction after HCT appears to be similar in children to that in adults and the etiologies alike (Hoffmeister et al. 2006; Beinert et al. 1996; Cerveri et al. 2001; Nysom et al. 1996; Frisk et al. 2003). In the largest longitudinal study, Hoffmeister and colleagues demonstrated pulmonary function declines in 55 % of pediatric patients followed for a median time of 10 years (Hoffmeister et al. 2006). However, only 26 % of those with decreased pulmonary function tests were symptomatic. Risk factors associated with pulmonary toxicity included single fraction TBI, time from transplant, cGVHD, and prior exposure to CY or anthracyclines among the most important (Hoffmeister et al. 2006).
2.1 Summary
Pulmonary toxicity from HCT has been substantially reduced from the early transplant experience due to numerous factors including the use of fractionated TBI, dose rate, total dose, lung blocking, improved diagnosis and treatment of pulmonary infections, and improved treatment of GVHD, as the most important. Conditioning with BU-CY may cause the same pulmonary toxicity risk as TBI containing regimens. Patients who develop GVHD have a higher rate of lung dysfunction as are patients who have previously had chest irradiation. Finally, the rate of pulmonary toxicity is similar in both children and adults.
3 Optic Structures
Ocular complications after BMT are common and can be classified as to the region of the eye which is affected: the anterior segment (lens, lacrimal gland); and the posterior segment (retina, optic disc). Damage to the anterior segment most often manifests as late complications occurring months to years after treatment while complications to the posterior segment are more likely to arise within 6 months to the first year after transplant (Coskuncan et al. 1994) and include microvascular retinopathy, optic disc edema, and hemorrhagic and infectious complications. The most commonly observed late complications of the anterior segment of the eye are posterior subcapsular cataracts and keratoconjunctivitis (Leiper 2002b).
Cataract formation in the crystalline lens of the eye has long been recognized as a complication of ionizing radiation (Chalupecky 1897). The lens has a high intrinsic radiosensitivity with a calculated α/β of approximately 1.2 Gy which suggests a HD rate and fractionation effect (Ozsahin et al. 1993; Schenken LL Hagemann 1975; van Kempen-Harteveld et al. 2000, 2002a). The latency period from irradiation to cataract formation ranges from 6 months to 35 years with an average latency period of 4 years for doses in the range of 6.5–11.5 Gy (Hall 1994). The latency period decreases with increasing radiation dose, with single fraction TBI, and increasing dose rate of TBI (Belkacemi et al. 1998a; Deeg et al. 1984).
The early experience with single fraction TBI (10 Gy) demonstrated an 80 % actuarial risk of cataract formation at 5 years (Deeg et al. 1984) (Fig. 2). The hazard rate of cataract development for patients treated with this regimen increased for the first 3 years and began to decline thereafter (Deeg et al. 1984). The probability of requiring surgical repair because of significant visual impairment was approximately 50 % at 6 years as determined by Kaplan–Meier analysis (Deeg et al. 1984). These findings have been confirmed in numerous subsequent publications (Ozsahin et al. 1993; van Kempen-Harteveld et al. 2002a, b; Belkacemi et al. 1998b; Bray et al. 1991; Tichelli et al. 1993).
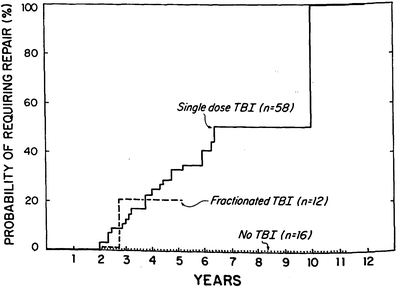
Fig. 2
Time course for RT-associated cateract formation requiring repair (with permission from Deeg et al.). Kaplan-Meier product limit estimates of the probability of requiring surgical repair of cataracts after marrow transplantation among patients given single-dose fractionated, or no TBI
As mentioned previously, the lens has a low α/β ratio which in radiation biological terms suggests that low dose rate schedules and fractionated schedules would reduce the cataractogenesis effect. Deeg and colleagues (1988) compared the incidence of cataracts in three groups of patients: single fraction TBI (10 Gy); fractionated (majority 12 Gy, 2 Gy bid × 3 days); and non-TBI preparative regimens (Deeg et al. 1988). As mentioned previously, the single fraction group had a 5-year actuarial rate of 80 versus 19 % for both the fractionated and the non-TBI prepared cohorts (p < 0.00005). These results have been confirmed by other investigators (Ozsahin et al. 1993; van Kempen-Harteveld et al. 2000, 2002a, b; Belkacemi et al. 1998b). However, with longer follow-up, the Seattle group found that even in patients receiving fractionated TBI, the probability of developing cataracts at 11 years was 34–50 % depending on the dose of TBI the patient received (Benyunes et al. 1995) which still is an improvement but not as favorable as initially reported. Fractionation appears to have its primary affect on the latency period to lens opacification (Leiper 2002b).
Other factors have been found to contribute to cataractogenesis in transplant patients in addition to irradiation. The use of steroids (prednisone) for the prevention or treatment of GVHD has been shown to increase cataract formation as has cranial irradiation (CI) preceding TBI (Deeg et al. 1984; Dunn JP et al. 1993; Frisk et al. 2000). On the other hand, the use of heparin post-transplant for the treatment of sinusoidal obstructive disease (SOS) appears to provide some measure of protection (Leiper 2002b; van Kempen-Harteveld et al. 2000, 2002a, b; Belkacemi et al. 1998a; Bray et al. 1991).
Pediatric transplant patients do not appear to be more susceptible to cataractogenesis than adult patients (Leiper 2002b) Most studies have not found age to be correlated with lens opacification (Deeg et al. 1984; Tichelli et al. 1993; Belkacémi et al. 1996) although Fife and colleagues suggested that patients <25 years of age had a lower corrective surgical rate than adults (Fife et al. 1994). As with adults single fractionated TBI is more cataractogenic than fractionated TBI (Deeg et al. 1984; Frisk et al. 2000). Frisk et al. (2000) suggested that two patterns for the progression were observed in children post-HCT. They reported either a rapid progression with loss of visual acuity within 2–4 years or little progression over time. Factors influencing rapid progression could not be determined due to the small numbers of patients.
Keratoconjunctivitis sicca (KCS) or dry-eye syndrome is a commonly encountered complication of BMT and it has similarities to Sjögrens syndrome in its clinical and pathological manifestatiöns (Tichelli et al. 1996). Diminished tear production resulting in superficial epithelial thinning and keratinization of the cornea and conjunctiva may progress to corneal ulceration, scarring, or in the most cases perforation. It has been reported to occur in up to 21–45 % of patients at 15 years (Tichelli et al. 1996; Calissendorff et al. 1989) although the incidence in children is not as well established and ranges from 6 to 47 % (Leiper 2002b; Calissendorff et al. 1989; Fahnehjelm et al. 2008). There is an association between KCS and the development of GVHD although KCS may develop in patients in whom no GVHD occurs (Leiper 2002b; Calissendorff et al. 1989; Fahnehjelm et al. 2008). KCS may also be related to the conditioning anti-neoplastic drugs, TBI, and/or the treatment for GVHD.
3.1 Summary
Cataract formation is common after TBI containing preparative regimens. Fractionation regimens appear to have a lower incidence of cataractogenesis although the major effect may be to prolong the latency period to cataract formation. Children do not appear to be more susceptible than adults to this complication. The use of steroids to treat GVHD increases the likelihood of cataract formation.
4 Renal Complications
Renal complications following irradiation are well documented in animal studies as well as human clinical trials. After single fraction whole-kidney irradiation, a dose associated with a 5 % renal complication rate (renal failure) at 5 years (TD 5/5) has been estimated to be 23 Gy to a single kidney, while the dose for a 50 % complication rate at 5 years (TD 50/5) has been estimated to be 28 Gy. If both kidneys are irradiated the TD 5/5 drops to 20 Gy (Emami et al. 1991; Kal and van Kempen-Harteveld 2006). The renal tolerance dose for TBI has been much more difficult to ascertain because of the myriad of factors involved in the transplant process including differing chemotherapy regimens, confounding infections or anti-infective therapies and the interaction of GVHD as a few of the variables involved. The tolerance dose does appear to be lower after TBI and is estimated to be approximately 14 Gy in adults and <12 Gy in children (Kal and van Kempen-Harteveld 2006).
Acute renal insufficiency or failure (ARF) is common in the post-transplant setting with an onset within 3 months of transplantation. The clinical presentation is that of severe anemia, hematuria, proteinuria, elevation of creatinine and blood urea nitrogen, hypertension, and evidence of microangiopathic intravascular hemolysis (Cohen et al. 1995; Tarbell et al. 1988). The pathophysiologically demonstrated injury is to the glomeruli which show severe changes, the hallmark of which is mesangiolysis (Leiper 2002b). The incidence of ARF in adults is estimated to be 26–53 % (Zager 1994; Zager et al. 1989; Gruss et al. 1995; Hingorani et al. 2005), while the incidence in children is estimated to be 5–50 % (Leiper 2002b; Kist-van Holthe et al. 1998; Van Why et al. 1991; Detaille et al. 2007). The cause of acute renal failure has many contributing nephrotoxic factors including sepsis, shock, SOS, allogeneic transplantation, conditioning chemotherapy agents (teniposide, fludarabine), and other nephrotoxic drugs including the use of cyclosporine for GVHD, aminoglycosides, amphotericin, foscarnet, busulfan, and vancomycin (Leiper 2002b; Kist-van Holthe et al. 1998; Cheng et al. 2008; Cohen 2001) among others. TBI is generally not implicated in the development of ARF (Hingorani et al. 2005).
Chronic renal dysfunction/failure (CRF), on the other hand, which commonly occurs 3–12 months after transplantation is a recognized but frequently underreported complication with only scattered case reports through the mid to late 1980s (Spruce et al. 1982; Steele and Lirenman 1979). Since that time, CRF has become a more recognized complication of BMT/HSCT in both adults and children. The clinicopathological findings and timing is suggestive of a radiation nephritis attributed to damage to the vascular epithelium (Leiper 2002b) with typical histopathological findings of mesangiolysis, tubulointerstitial scarring, and in late stages thrombotic microangiography (Cohen and Robbins 2003). Cohen and colleagues reported that approximately 5–20 % of adult patients developed late renal dysfunction after undergoing BMT(Cohen et al. 1995, 2007; Cohen 2001). Hingorani and colleagues (2007) from Seattle reported an incidence of chronic renal disease in 23 % in 1635 adult patients treated from 1991 to 2002. The determination of causative factors remains inconsistent from study to study. TBI has been invoked to be an important nephrotoxin (Kal and van Kempen-Harteveld 2006; Cohen et al. 1995, 2007; Cheng et al. 2008; Cohen and Robbins 2003; Lawton et al. 1991; Miralbell et al. 1996; Otani et al. 2005) Cheng et al. (2008) examined the impact of various drug therapies and radiation dose on the development of CRF by performing metanalysis on 12 published series. Their analysis suggested that total radiation dose, radiation dose rate (>10 cGy/min), and the use of fludarabine increased the likelihood of developing CRF in a mixed population of patients (adults and children). However, when the pediatric patients were analyzed separately, no TBI parameters were correlative but the use of cyclosporine or teniposide was associated with CRF (Cheng et al. 2008). In contrast Hingorani did not find any association between the development of CRF and conditioning with TBI (Hingorani et al. 2007) in a very large cohort of patients from a single institution. The development of ARF has been shown to be an important predictor of CRF in a number of studies (Kist-van Holthe et al. 1998; Detaille et al. 2007; Hingorani et al. 2007) but not in others (Van Why et al. 1991). The presence and/or the treatment of GVHD have also been implicated in the development of CRF particularly with the use of cyclosporine (Zager 1994; Cohen 2001; Cohen et al. 2007, 1993; Lawton et al. 1991).
The incidence of CRF in the pediatric transplant population has been variously reported to occur in 5–45 % of children undergoing transplantation (Tarbell et al. 1988; Kist-van Holthe et al. 1998, 2005) and may be higher than the incidence in adults. The factors discussed above associated with CRF in adults have been variously implicated in the pediatric population as well (Tarbell et al. 1988; Kist-van Holthe et al. 1998, 2005; Patzer et al. 2003). Cheng et al. (2008), however, suggest in their metanalysis that children are not more susceptible to the nephrotoxic affects of TBI, but rather the different conditioning chemotherapy regimens used in children are responsible for the apparent susceptibility and other series would support that finding (Van Why et al. 1991; Patzer et al. 2003; Chou et al. 1996).
4.1 Summary
Chronic renal insufficiency occurs in approximately 20 % of patients post-HCT and has many etiologies post-HCT including the use of nephrotoxic drugs, acute renal insufficiency during the transplant and shortly thereafter often due to hemodynamic changes, and TBI among the most notable. TBI appears to be a major risk factor for the development of CRF and seems to have a similar effect in adults and children.
5 Cardiac
Late cardiotoxicity may present in a variety of ways depending on the type and combinations of cardiotoxic drugs which are utilized in preparative regimens for HCT or prior exposure to agents before HCT (Leiper 2002a). These signs and symptoms include clinically significant congestive heart failure (CHF), arrhythmias, fatal cardiomyopathy, pericardial and valvular disease, electrocardiogram changes, or asymptomatic reduction in left ventricular ejection fraction as determined by echocardiography. Clinical Oncology Trials are not designed in most cases to closely monitor cardiac function over long follow-up periods so it is likely that clinically asymptomatic patients are underdiagnosed. The incidence of clinically significant symptoms has varied from 1 to 66 % as ascertained from the literature (Fujimaki et al. 2001; Lehmann et al. 2000; Morandi et al. 2005; Sakata-Yanagimoto et al. 2004; Pai and Nahata 2000) in adults.
The potential cardiotoxicity of numerous chemotherapeutic agents used either as single agents or in combination for the treatment of oncological diseases has been well established (Morandi et al. 2005; Pai and Nahata 2000). Table 3 lists the most commonly encountered agents in the transplant setting with estimated incidences and mortality rates associated with the use of those drugs which were generated from a literature review of publications over a 30-year period (Morandi et al. 2005; Pai and Nahata 2000). The most consistent associations with cardiac dysfunction reported are with anthracyclines and cyclophosphamide (Morandi et al. 2005), although other drugs have also been implicated as listed in Table 3. Other cardiotoxic drugs which are not used in HCT but may be used for treatment of malignancies prior to the transplant procedure include: alkylating agents, ifosfamide, Cisplatin, mitomycin, carmustine, and chlormethine; antimetabolites: fluorouracil; antimicrotubule agents: paclitaxel, vinca alkaloids, etoposide, and teniposide; and other miscellaneous drugs: amascarine, cladribine, asparaginase, pentostatin, and tretinoin (Pai and Nahata 2000; Carver et al. 2007). More recently, cardiac toxicity has been associated with several new tyrosine kinase inhibitors (sunitinib and sorafenib) with mild to life-threatening events in almost 20 % of patients (Carver et al. 2007; Schmidinger et al. 2008) and asymptomatic cardiac findings in an additional 16 %. Prior use of these agents with resultant cardiotoxicity may lead to worsening of cardiac function after HD chemotherapy used in HCT preparative regimens (Morandi et al. 2005).
Table 3
Clinical cardiac toxicity of single-agent high dose chemotherapeutic agents and their combinations
Single-agent/combination
|
Risk factors
|
Type/incidence
|
Increased mortality
|
|
---|---|---|---|---|
Cardiac dysfunctiona
|
Doxo ≥400 mg/m2b
|
|||
Cyclophosphamide
|
No
|
No
|
CHF, acute restrictive cardiomyopathy, pericardial effusion (1.7–28 %)
|
Yes (2–14 %)
|
Cyclophosphamide and mitoxantrone
|
No
|
Yes
|
CHF (25–66 %)
|
Yes (4–33 %)
|
Cyclophosphamide and cytarabine
|
No
|
Yes
|
CHF, acute restrictive cardiomyopathy, pericardial effusion (1.7–33 %)
|
Yes (9–26 %)
|
Cytarabine
|
No
|
Yes
|
Arrhythmias, pericarditis
|
No
|
Melphalan
|
No
|
No
|
Arrhythmias/(5 %)
|
No
|
Melphalan and TBI
|
No
|
No
|
Constrictive pericarditis
|
No
|
Melphalan and fludarabine
|
No
|
No
|
CHF (2–14 %)
|
Yes (1–3 %)
|
Fludarabine
|
Yes
|
CHF (8 %)
|
No
|
|
Thiotepa and melphalan
|
No
|
Yes
|
CHF (4.1 %)
|
No
|
Thiotepa and carboplatin or TBI
|
Yes
|
Yes
|
CHF (5.3 %)
|
No
|
Ifosfamide
|
No
|
Yes
|
CHF, arrhythmias (15.2 %)
|
Yes (2 %)
|
Carmustine
|
No
|
No
|
Myocardial ischemia
|
No
|
Cyclophosphamide (CY) containing HD chemotherapy regimens (HD chemotherapy) used for HCT conditioning are the most commonly associated regimens with cardiotoxicity (Morandi et al. 2005) (Table 3). In studies from the 1970s and 1980s, the incidence was reported to be up to 43 %. It has been found, however, that cardiotoxicity is dose- and schedule-dependent and with modern multifractionated administration, this incidence has been substantially reduced (Morandi et al. 2005; Braverman et al. 1991). The injury appears to be initially to the endothelial cells with subsequent extravasation of toxic CY metabolites which leads to myocyte injury, interstitial hemorrhage, and edema (Morandi et al. 2005). The injury occurs during or soon after (within 3 weeks) administration of the drug although the deleterious effects may manifest weeks to months afterward (Morandi et al. 2005; Baello et al. 1986).
The cardiotoxicity of anthracyclines is well established in adults as well as in children (Morandi et al. 2005; Pai and Nahata 2000; Carver et al. 2007; Alvarez et al. 2007; Yahalom and Portlock 2008; Uderzo et al. 2007; van Dalen et al. 2006; Lipshultz 2007; Lipshultz et al. 2005). Although this agent is not commonly used in HCT preparative regimens, its ubiquitous use for the treatment of numerous cancer types exposes many patients to the harmful effects prior to HCT. Approximately 60 % of children treated for malignancies receive anthracyclines (van Dalen et al. 2006). Risk factors associated with anthracycline toxicity include younger age at treatment, longer follow-up, female sex, cumulative doses, and higher dose rates (Fujimaki et al. 2001; Morandi et al. 2005; Carver et al. 2007; Alvarez et al. 2007; van Dalen et al. 2006; Lipshultz 2007; Simbre et al. 2005). The injury is to the myocytes and is progressive in nature. The left ventricular (LV) dysfunction develops through two separate pathways. The first is characterized by depressed LV function due to decreased LV contractility. The second pathway is characterized by an elevated afterload (stress on the heart wall) (Alvarez et al. 2007; Lipshultz 2007). Patients are then at risk for developing CHF and associated mortality from pump failure (Alvarez et al. 2007; Lipshultz 2007).
The role of TBI as a causative factor of late cardiac toxicity is less certain. The cardiotoxicity of irradiation to the heart has long been recognized (Carver et al. 2007; Yahalom and Portlock 2008; Adams et al. 2003a, b). Much of the data collected is from long-term survivors of treated childhood Hodgkin’s disease. Mediastinal irradiation can cause damage to the pericardium, myocardium, endocardium, vascular system, conduction system, and cause autonomic dysfunction (Carver et al. 2007; Adams et al. 2003a). Factors which increase the risk of cardiac sequelae after mediastinal irradiation include the volume of the cardiac silhouette irradiated, total dose >30 Gy, and a dose fraction >2 Gy per day (Carver et al. 2007; Adams et al. 2003a). The effect of irradiation on the cardiac structures is felt to be “deterministic” that is there is a threshold below which no damaging effect is noted (Hendry et al. 2008; Schultz-Hector and Trott 2007) and that threshold from clinical studies appeared to be in the 30 Gy range. More recently, some investigators have suggested that there is no accurate threshold dose for cardiovascular risk and that a radiobiologically equivalent mean dose to the heart of 1–2 Gy may be the threshold dose above which late cardiovascular toxicity may occur (Hendry et al. 2008; Schultz-Hector and Trott 2007). If this threshold is correct, TBI doses currently used would be expected to have some cardiac toxicity but it is unclear from published data whether there is or is not cardiotoxicity from TBI (Fujimaki et al. 2001; Lehmann et al. 2000; Sakata-Yanagimoto et al. 2004; Baello et al. 1986; Carlson et al. 1994). However, previous radiotherapy to the mediastinum or left chest wall increases the likelihood of cardiotoxicity especially if the patient has also been exposed to anthracyclines prior to HD—chemotherapy used for conditioning (Morandi et al. 2005; Sakata-Yanagimoto et al. 2004; Adams et al. 2003a, b; Cazin et al. 1986) or have preexisting cardiac dysfunction (Fujimaki et al. 2001).
The incidence of cardiotoxicity in children after HCT is difficult to ascertain as the data is sparse and results often conflicting (Leiper 2002a). Late cardiac toxicity manifests similarly in children and adults with the signs and symptoms mentioned above. The incidence of symptomatic cardiac abnormalities appears to be <15 % in most studies (Leiper 2002a; Uderzo et al. 2007; Eames et al. 1997; Leung et al. 2000a; Nicolini et al. 2000). The reported incidence of abnormalities found in asymptomatic patients is far higher and approximates 40 % (Leiper 2002a; Eames et al. 1997; Pihkala et al. 1994), while the incidence of abnormalities on treadmill exercise testing may be as high as 75 % (Eames et al. 1997). Children who have received previous mediastinal irradiation and/or anthracyclines are at an increased risk of developing cardiotoxicities just as their adult cohorts (Leiper 2002a; Eames et al. 1997; Pihkala et al. 1994; Shankar et al. 2008; Armenian et al. 2008). The effects of cardiotoxic agents used during HCT preparation seem similar in adults and children. The role of TBI in inducing cardiotoxicity remains as obfuscated in children as in adults with conflicting reports (Leiper 2002a; Eames et al. 1997; Nicolini et al. 2000; Pihkala et al. 1994).
5.1 Summary
Late cardiac toxicity does occur in both children and adults after HCT and is likely underreported because some abnormalities may only be discovered by cardiac stress testing. Exposure to cardiotoxic drugs either prior to HCT or during HCT is an important factor related to late cardiac effects as is prior mediastinal irradiation. TBI may be responsible for some added cardiotoxicity but is unlikely a major cause.
6 Gastrointestinal
Toxicity of the gastrointestinal organ system is common after HCT affecting primarily the liver although acute intestinal injury is a very early consequence of the preparative regimens. Liver injury is particularly common after HCT and has several etiologies including hepatic veno-occlusive disease (VOD) or more recently named sinusoidal obstruction syndrome (SOS), GVHD, viral and fungal infections, tumor invasion, and cholestatic disorders (Barker et al. 2005; Cheuk et al. 2007; Junghanss et al. 2002; Strasser et al. 1999). Although largely an early complication of transplantation, liver damage is a significant toxicity and so will be discussed. VOD/SOS does not appear to be related to cirrhosis in long-term survivors of HCT (Strasser et al. 1999).
One of the most serious complications of HD chemotherapy and HCT is VOD/SOS of the liver. As a transplant-related conditioning regimen toxicity, VOD/SOS is one of the most common causes of early morbidity and mortality after transplantation in both adults and children (Leiper 2002a; Barker et al. 2005; Cheuk et al. 2007; Cesaro et al. 2005; Kumar et al. 2003; Litzow et al. 2002; Wadleigh et al. 2003; Bresters et al. 2007; Carreras 2000; Palladino et al. 2008). The onset of VOD/SOS most commonly occurs within the first 20–35 days after transplantation although some cases are seen later and are associated with preparative regimens containing multiple akylating agents (Wadleigh et al. 2003; Carreras 2000). The clinical manifestations of VOD/SOS include: weight gain not attributable to fluid overload but due to renal sodium retention; development of edema (60 %) and ascites (25 %); hepatomegaly; and liver tenderness (Litzow et al. 2002; Wadleigh et al. 2003; Carreras 2000; Coppell et al. 2003; Ganem et al. 1988; Ho et al. 2007). Hyperbilirubinemia occurs in most patients and elevation of other liver function tests (transaminases, alkaline phosphatase) may occur (Carreras 2000; Coppell et al. 2003). The incidence of VOD/SOS ranges from 10 to 60 % in reported series with most suggesting an approximately 20 % rate (Kumar et al. 2003; Carreras 2000; Palladino et al. 2008; Coppell et al. 2003; Ganem et al. 1988; Ho et al. 2007). The classification of VOD/SOS into three groupings mild, moderate, and severe, is based on the ultimate outcome of the clinical syndrome rather than any clinical or laboratory findings at the time of diagnosis. In both the mild and moderate disease, most patients fully recover with or without treatment (Coppell et al. 2003). The mortality rate for severe VOD/SOS is extraordinarily high and approaches 100 % in some series (Ho et al. 2007), but appears to be 20–50 % in most studies (Kumar et al. 2003; Litzow et al. 2002; Wadleigh et al. 2003; Carreras 2000; Coppell et al. 2003; Ganem et al. 1988; Lazarus and McCrae 2008).
The earliest pathophysiological injury which leads to the development of VOD/SOS is injury to the hepatic venules and sinusoidal endothelium in the centrilobular zone (Zone 3) of the liver acinus (Kumar et al. 2003; Wadleigh et al. 2003; Coppell et al. 2003; Ho et al. 2007). Histological early features include subendothelial edema, erythrocyte extravasation, fibrin deposition, and expression of factor VIII (von Willebrand factor) within the venule walls leading to concentric narrowing of these vessels (Kumar et al. 2003; Wadleigh et al. 2003; Coppell et al. 2003; Ho et al. 2007). As the process continues with release of other markers of inflammation, the hepatic sinusoids become dilated and hepatocyte necrosis occurs which eventually leads to intense collagen deposition in the sinusoids and venules and fibrosis of the lumens. Occlusion of the vessels causes hepatic outflow obstruction which leads to a post-sinusoidal intrahepatic portal hypertension (Carreras 2000). Additional ischemia leads to further hepatocellular necrosis with hepatorenal pathophysiology a clinical feature of this process. Most patients succumb to multiorgan failure as a byproduct of hepatic injury (Kumar et al. 2003; Wadleigh et al. 2003; Coppell et al. 2003; Ho et al. 2007) in the severe form of this complication. The treatment of VOD/SOS is largely supportive with maintenance of fluid and electrolyte balance among the most important measures. The use of defibrotide, a novel drug which has local antithrombotic, anti-ischemic, and anti-inflammatory activities in the past decade has shown promise in reversing the process is a substantial portion of patients as well as reducing the mortality without apparent long-term toxicities (Ho et al. 2007; Lazarus and McCrae 2008; Benimetskaya et al. 2008).
Risk factors that may be associated with the development of VOD/SOS have been evaluated by numerous investigators (Cesaro et al. 2005; Kumar et al. 2003; Litzow et al. 2002; Wadleigh et al. 2003; Carreras 2000; Coppell et al. 2003; Ganem et al. 1988; Ho et al. 2007; McDonald et al. 1993; Carreras et al. 1998) and often seem to have contradictory results largely reflecting differences in study design as well as HCT treatment dissimilarities. Table 4 lists the risk factors associated with VOD/SOS from several studies (Carreras 2000). The single most important factor from these and other studies appears to be the presence of hepatic injury prior to HCT (Coppell et al. 2003; McDonald et al. 1993; Carreras et al. 1998). The injury may be caused by a variety of factors including: active hepatitis, previous abdominal irradiation, and excessive alcohol intake. Other risk factors which are related to the transplant or the treatment of complications (infections, GVHD, etc.) include high-intensity conditioning regimens frequently seen in unrelated donor HCT; known hepatotoxic drugs such as cyclophosphamide, busulfan, dacarbazine, gemtuzumab, methotrexate, vancomycin, acyclovir, sirolimus, ketoconazole, cyclosporine, and amphotericin B; single dose TBI and total dose >12 Gy. In addition, there may be genetic factors such as mutations of genes encoding enzymes in the critical liver enzyme glutathione which are involved in the catabolism of many of the drugs mentioned above and when depleted leads to hepatic injury (Coppell et al. 2003). The presence of more than one risk factor increases the likelihood of developing VOD/SOS (Cesaro et al. 2005).
Table 4
Risk factors for veno-occlusive disease of the liver after hemopoietic cell transplantation
Risk factor
|
Lower risk
|
Higher risk
|
---|---|---|
Type of transplantation
|
Syngeneic/autologous
|
Allogeneic
|
Type of donor
|
HLA identical sibling: other related
|
HLA identical sibling: unrelated
|
Grade of compatibility
|
Match: minor-mismatch
|
Match: major mismatch
|
Type of progenitors
|
Peripheral blood
|
Bone marrow
|
T cell depleted
|
Non T cell depleted
|
|
Diagnosis
|
Nonmalignancy (remission)
|
Malignancy (relapse)
|
Conditioning regimen
|
Fractionated
|
Single dose
|
TBI
|
Less than 12 Gy
|
More than 12 Gy
|
Total dose
|
Low dose rate
|
High dose rate
|
Busulfan
|
Cy alone: Cy-TBI
|
Cy alone: BVC
|
Administration
|
Non-containing: dose-adjusted
|
Non-containing: non-dose-adjusted
|
Timing
|
Others combinations
|
Busulfan first
|
Cy to TBI 36 h
|
Cy to TBI 12 h
|
|
Age
|
Younger
|
Older
|
Sex
|
Male
|
Female
|
Karnofsky index
|
100 ± 90
|
Lower than 90
|
Transaminase level before conditioning
|
Normal
|
High
|
HCT number
|
First
|
Second
|
Previous liver radiation
|
No
|
Yes
|
Liver status
|
Normal
|
Fibrosis, cirrhosis, tumour
|
Concomitant drugs
|
No administration of progestogens, ketoconazole, cyclosporine, methotrexate, amphotericin B, vancomycin, acyclovir
|
The incidence of VOD/SOS in children is approximately the same as in adults at 20 % (Barker et al. 2005; Cheuk et al. 2007; Cesaro et al. 2005; Reiss et al. 2002) and the mortality is also similar. The risk factors are also similar to those found in adults (Cheuk et al. 2007). The data reported by Cesaro et al. (2005) also suggests that younger age within the pediatric population is related to a higher incidence of VOD/SOS. The treatment of this complication is also the same as in adults, although the experience with defibrotide in the pediatric population is not as mature as in adults.
6.1 Summary
VOD/SOS remains a significant complication of HCT with a high mortality rate in both children and adults. Hepatic injury from drugs, irradiation, infections, and genetic factors prior to and related to HCT and its complications are associated with the development of this entity. Irradiation, either previously to the liver or single fraction TBI, or doses over 12 Gy likely add to the risk of hepatic toxicity.
7 Endocrine/Growth and Development
Endocrine complications are among the most prevalent late effects after HCT particularly in children and may have significant impact on quality of life (Brennan and Shalet 2002; Cohen et al. 2008; Ghavamzadeh et al. 2003; Kauppila et al. 1998a, b; Littley et al. 1991; Rutter and Rose 2007; Sanders 2004, 2007; Sanders et al. 2009; Tauchmanovà et al. 2002). The toxicities of various agents used in HCT (cytotoxins, irradiation, etc.) may occur at the central level (hypothalamus-pituitary axis) or at the target organ site (gonads, thyroid, parathyroid, and adrenal glands). The extent of endocrinological abnormalities depends on numerous factors of which the following appear to be most important: age; types and dose schedules of cytotoxic agents used; the inclusion of TBI and the dose regimen utilized; and previous irradiation. The deleterious effects can be particularly striking in children with growth and developmental inhibition, with younger aged children having more pronounced impairments (Chemaitilly and Sklar 2007).
7.1 Thyroid
Thyroid dysfunction is one most common endocrinopathies following HCT in both children and adults (Brennan and Shalet 2002; Cohen et al. 2008; Littley et al. 1991; Sanders 2004, 2007; Sanders et al. 2009, 1989; Chemaitilly and Sklar 2007; Al-Fiar et al. 1997; Katsanis et al. 1990). The most common biochemical finding is that of a mildly elevated basal thyroid stimulating hormone (TSH) level with normal serum thyroxine levels or an exaggerated response to TSH-releasing hormone (Brennan and Shalet 2002; Sanders 2004; Katsanis et al. 1990; Legault and Bonny 1999) indicating that the injury is to the target organ rather than the hypothalamic-pituitary axis. This compensated hypothyroidism may be transient or progress to overt (uncompensated) hypothyroidism over the ensuing decades. The incidence of compensated hypothyroidism ranges from approximately 10 to 50 % in most adult and pediatric series with uncompensated hypothyroidism occurring in approximately 0–15 % (Cohen et al. 2008; Kauppila et al. 1998a; Sanders 2004, 2008; Ishiguro et al. 2004; Berger et al. 2005) although Al-Friar and colleagues reported a lower incidence in adults (8.9 %) (Al-Fiar et al. 1997). The variability in incidence may be related to length of follow-up with Sanders et al. showing the risk for developing thyroid abnormalities extends at least up to 28 years in children (Sanders 2008) (Fig. 3). One consequence of hypothyroidism in children is its role among other factors in growth inhibition (Sanders 2008; Chemaitilly et al. 2007).
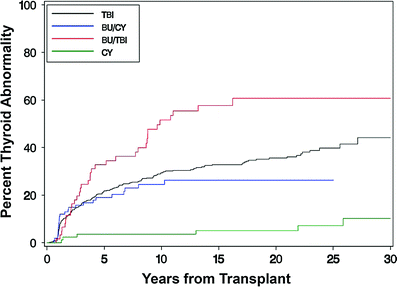
Fig. 3
Development of thyroid dysfunction by preparative regimen for patients undergoing transplant (with permission from Sanders 2008). Cumulative incidence of developing thyroid dysfunction after hematopoietic cell transplantation divided by type of preparative regimen received: TBI, busulfan, busulfan TBI, or cyclophosphamide only
TBI as has been implicated as the major factor in post-HCT thyroid dysfunction in most reported series. Fractionated TBI has been reported in most studies to cause less thyroid suppression than single fraction TBI with an incidence of thyroid abnormalities of approximately 15 versus 40 % (Brennan and Shalet 2002; Sanders et al. 2009; Sanders 2008; Berger et al. 2005; Thomas et al. 1993; Ranke et al. 2005). Other radiotherapy factors which have been associated with an increased incidence of thyroid dysfunction include age <10 (Sanders et al. 2009; Ishiguro et al. 2004) and previous local irradiation involving the neck (Sanders et al. 2009). A higher incidence was also reported when Bu was used in conjunction with TBI compared with CY + TBI (Sanders et al. 2009) (Fig. 3). Interestingly, thyroid dysfunction may occur after chemical conditioning only regimens that contain BU/CY with an incidence of approximately 15 % (Sanders 2004; Sanders et al. 2009; Tauchmanovà et al. 2002; Al-Fiar et al. 1997; Berger et al. 2005; Bakker et al. 2004). Conditioning with HD-Cy alone has a very low incidence of thyroid dysfunction (Sanders et al. 2009, 1989).
7.2 Growth and Development in Children
Abnormalities of growth and development in children and young adults are among the most common and vexing late complications after HCT (Brennan and Shalet 2002; Sanders 2007, 2008; Chemaitilly and Sklar 2007; Sanders et al. 2005a). The causes of linear growth impairment are numerous and are both endocrine and nonendocrine in nature. Patient characteristics (age), treatment variables (preparative regimen, prior irradiation), and post-HCT complications such as GVHD, and nutritional status are the primary determinants of growth impairment in this population (Brennan and Shalet 2002; Chemaitilly and Sklar 2007; Sanders 2008; Bakker et al. 2006; Couto-Silva et al. 2006).
The age at the time of HCT is a significant factor in predicting final adult height. Children who are transplanted before the age of 6–8 years have the greatest risk of growth inhibition and significantly reduced adult height due both to GH deficiency and the direct effect of irradiation on the epiphyseal growth plates (Sanders 2008; Ranke et al. 2005; Bakker et al. 2006; Couto-Silva et al. 2006).
Growth hormone (GH) deficiency has been a long recognized complication of HCT in children with a reported incidence of 20–85 %. Variables which contribute to such inconsistent rates include differences in the age at HCT, preparative regimen, dose and fractionation scheme of TBI, previous CI, time to testing after HCT, and method of GH testing (Sanders 2008). GH stimulates growth of the epiphyseal cartilage and ensuing bone growth directly through the actions of insulin growth factor 1. When insufficient GH is present, growth velocity and bone maturation are impaired. Irradiation to the hypothalamic-pituitary axis has been shown to be a primary cause of GH deficiency and is related to total dose, fractionated scheme utilized, and time elapsed to treatment (Rutter and Rose 2007; Chemaitilly and Sklar 2007; Sanders 2008). Children receiving CI for acute lymphoblastic leukemia (ALL) at doses of 18 Gy have a significant incidence of GH deficiency (Rutter and Rose 2007). A threshold dose of 18 Gy has been suggested (Ranke et al. 2005), but GH declines have been reported after TBI fractionated doses of 12 Gy (Sanders 2008; Ogilvy-Stuart et al. 1992; Brauner et al. 1997). Fractionated TBI appears to interfere with GH secretion less than single fractionated TBI in most studies (Thomas et al. 1993; Sanders et al. 2005a; Bakker et al. 2006; Brauner et al. 1997; Cohen et al. 1999). Children who have received CI prior to HCT are more likely to have GH deficiency and lessened adult height compared to those not having CI (Cohen et al. 2008; Chemaitilly and Sklar 2007; Sanders 2008; Bakker et al. 2006; Cohen et al. 1999). HD-CT only preparative regimens have for the most part been shown to cause much less growth inhibition compared to TBI containing regimens (Chemaitilly and Sklar 2007; Sanders 2008; Cohen et al. 1999) with Bu containing regimens possibly having an affect although not likely mediated through GH deficiency mechanisms. The data pertaining to GH deficiency in adults after HCT is sparse, but there appears to be a negligible incidence of GH deficiency with little clinical relevance (Kauppila et al. 1998a; Chemaitilly and Sklar 2007).
In addition to the affect on GH secretion, TBI may cause direct damage to the epiphyseal growth plates of bone and cartilage with ensuing skeletal dysplasia (Brennan and Shalet 2002; Ranke et al. 2005). Disproportionate growth has been noted in several studies (Chemaitilly et al. 2007; Bakker et al. 2006), with spinal growth being more impaired than growth of the legs after TBI which is consistent with skeletal injury rather than GH deficiency. The influence on growth centers is age dependent with fractionated doses as low as 10 Gy causing growth inhibition (Hogeboom et al. 2001; Paulino et al. 2000). The growth of craniofacial bones may also be affected, particularly in very young children (Sanders 2004; Dahllöf et al. 1989) with a reduction in lower face height (Dahllöf et al. 1989). Prior irradiation to the spine such as for Hodgkin’s disease or neuroblastoma amplifies the growth inhibition.
Other endocrinopathies which potentially can influence growth and development in children are hypothyroidism, deficiencies in Gonadotropin releasing hormone which stimulates the release of LH (luteinizing hormone) and FSH (follicle stimulating hormone), adrenal corticotrophin hormone, and prolactin. Deficiencies in these hormones are uncommon after TBI containing regimens unless prior CI was used and even in those patients the incidence of deficits is low (Rutter and Rose 2007; Ranke et al. 2005). Figure 4 from Rutter shows the doses of irradiation to the hypothalamus-pituitary axis which are felt to cause hormone deficiencies in children.
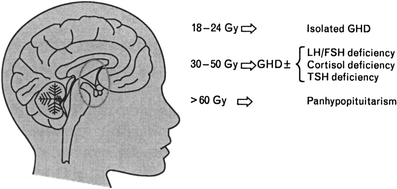
Fig. 4
Illustration of the doses of RT to the hypothalamus–pituitary axis felt to cause hormone deficiencies in children (with permission from Rutter and Rose 2007). GHD Growth hormone deficiency, LH luteinizing hormone, FSH follicle stimulating hormone, TSH thyroid stimulating hormone. Association between the dose of fractionated cranial irradiation, including the hypothalamic-pituitary axis within the field, and anterior pituitary hormone deficits
7.3 Gonadal and Reproductive Function
In contradistinction to late growth-related abnormalities associated with HCT, gonadal dysfunction is frequent in both children and adults. The prevalence is high and is upward of 90 % in men, women, and children (Brennan and Shalet 2002; Tauchmanovà et al. 2002; Mertens et al. 1998). Gonadal toxicity may result in failure to initiate or progress through puberty, infertility, sexual dysfunction, and require hormone replacement and is influenced by age, conditioning regimen, sex, and previous therapy (Kauppila et al. 1998a; Littley et al. 1991; Sanders 2004, 2007; Chemaitilly and Sklar 2007; Sanders et al. 1989; Mertens et al. 1998). A loss of gonadal function has a major negative impact on quality of life of HCT survivors (Claessens et al. 2006). The affects of HCT on gonadal and reproductive functioning will be examined in the following paragraphs.
7.4 Puberty
The transition from childhood to adulthood during puberty is a period of complex physiological transformations with substantial changes in gonadal and GH activity, development of secondary sex characteristic, and heightened growth velocity. The timing and sequence of these changes are variable between individuals but there is a delay in pubertal development by about 2 years in boys compared to girls. The initiation and progression through puberty requires an intact hypothalamic–gonadal axis and disruption in that system, which was addressed previously, may lead to growth delays, delayed or absent pubertal development, and infertility. The FSH/LH surge which initiates puberty stimulates the gonads to produce germ cells and secrete sex hormones which produce the physiological changes. Injuries to the target organs (testes, ovaries) may also result in a delayed or absent pubertal growth and development, and in fact gonadal injury is the principal cause of pubertal delay or arrest and infertility in both children and adults and is characterized by hypersecretion of FSH and LH (Brennan and Shalet 2002; Cohen et al. 2008; Kauppila et al. 1998a; Sanders 2004, 2008; Chemaitilly and Sklar 2007; Mertens et al. 1998).
7.5 Testes
The major causes of testicular dysfunction in children and young adults after HCT consists of the use of TBI and HD alkylating agents in the preparative regimens (Sanders 2004; Chemaitilly and Sklar 2007). The germ cell epithelium in the testis which is the target organ for FSH action is particularly sensitive to the affects of irradiation and HD-alkylating agents as compared to the Leydig cells (testosterone production). Transient azoospermia may occur after exposure to doses as low as 15 cGy. Complete recovery occurs after 50–60 days after 15 cGy; 9–18 months after doses <1 Gy; may occur 30 months for 2–3 Gy; and may occur 5 or more years for doses of 4–6 Gy (Greven and Paunesku 2006) although permanent sterility may result after doses as low as 2 Gy (Kauppila et al. 1998b; Shalet 1993). Cumulative doses above 6 Gy are likely to lead to permanent germ cell dysfunction and sterility in practically all males, and thus most male patients treated with TBI will be infertile (Littley et al. 1991; Sanders 2004, 2008; Chemaitilly and Sklar 2007; Mertens et al. 1998; Greven and Paunesku 2006; Sanders et al. 1986; Sanders et al. 1983). Pubertal status (prepubertal, pubertal, and postpubertal) at the time of TBI does not appear to affect the incidence of sterility. Leydig cells, in contrast, are much more resistant to the affects of irradiation with cumulative doses of >20 Gy necessary to interfere with testosterone secretion. Previous testicular irradiation as prescribed for testicular involvement (doses 24 Gy) may lead to Leydig cell insufficiency and require androgen replacement therapy (Sklar et al. 1990; Brauner et al. 1988). Fractionated TBI does not decrease the incidence of infertility but may actually be more deleterious than single fraction (Mertens et al. 1998; Greven and Paunesku 2006; Ash 1980
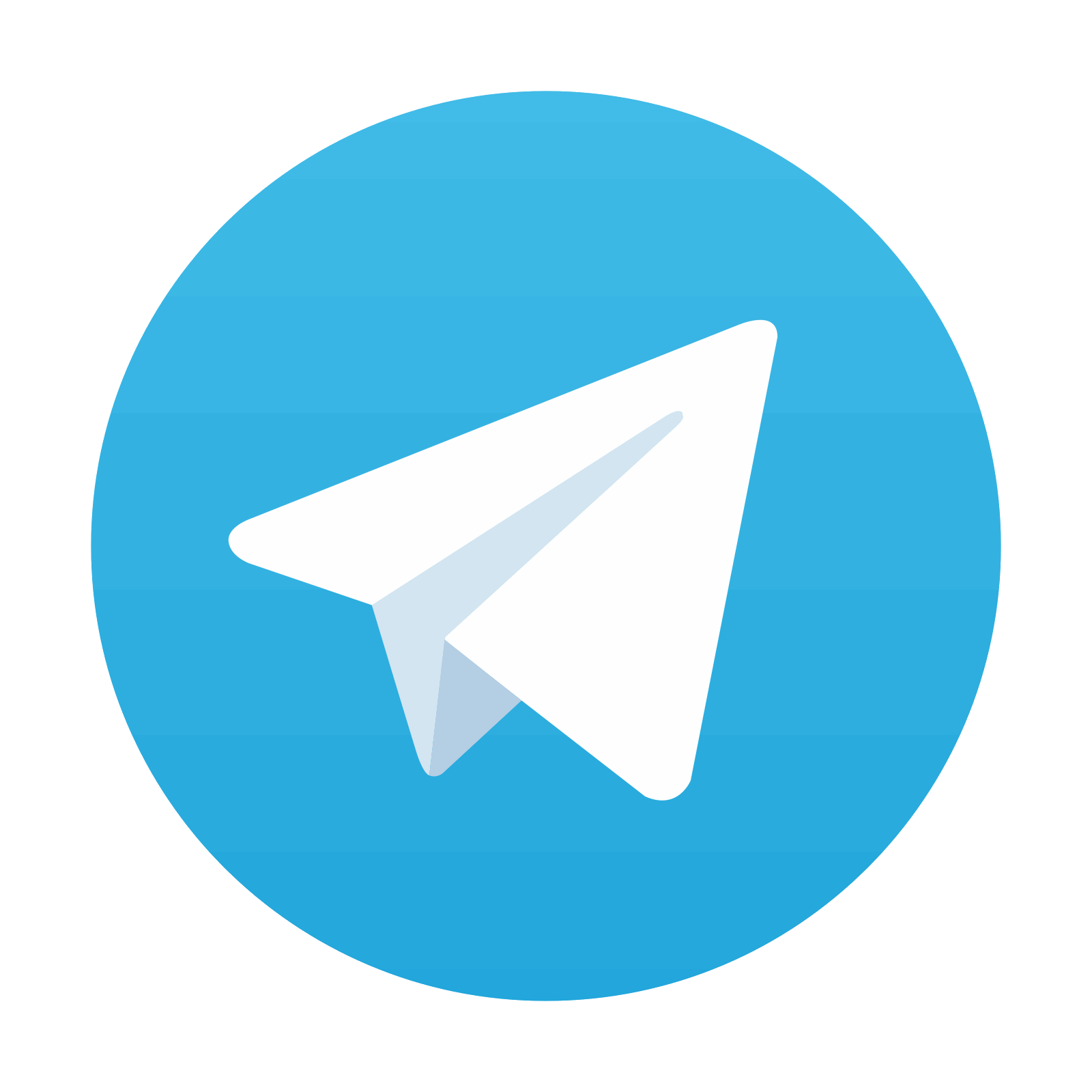
Stay updated, free articles. Join our Telegram channel

Full access? Get Clinical Tree
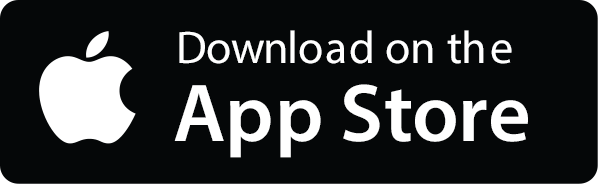
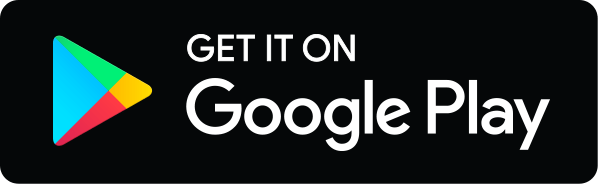