Fig. 34.1
Zenith endovascular stent system (Permission for use granted by William Cook Europe, Bjaeverskov, Denmark)
Talent Abdominal Stent Graft (MedtronicVascular, Santa Rosa, CA)
The device is a self-expanding modular system composed of serpentine-shaped nitinol stents integrated into a woven polyester matrix (Fig. 34.2). The stents are discontinuous and are spaced along a full-length nitinol spine. The latter wire provides columnar strength to a graft that is otherwise flexible enough to accommodate aortoiliac angulations. The spine also prevents twisting and longitudinal infolding of the endograft during deployment. The proximal aortic fixation end has a 1.5-cm uncovered nitinol frame that allows for transrenal fixation of the device. These characteristics may allow increased potential for treating patients with challenging aortic neck configurations [4, 5]. Since its original introduction, the endograft has been modified to include the use of a thinner, low-profile graft fabric to create the Talent Low-Porosity System (LPS). Later, the Talent endograft nitinol springs were chemically treated to enhance durability, and the position of the iliac longitudinal connecting bar was moved from lateral to medial to improve conformability and decrease risk of kinking and thrombosis, resulting in the latest-generation Talent eLPS device. The Talent Abdominal Stent endograft has a 22F delivery system with a hydrophilic coating which does not require a delivery sheath. A 24F delivery system is required for 30 to 36-mm devices and allows treatment of up to 32-mm neck diameters with a neck length of 10 mm and <60° of angulation.
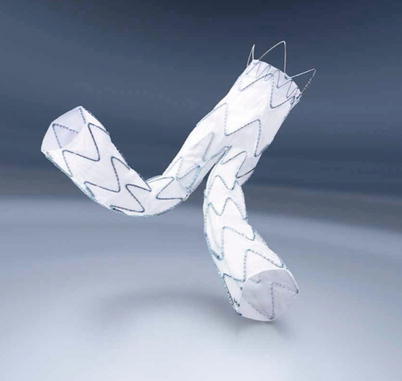
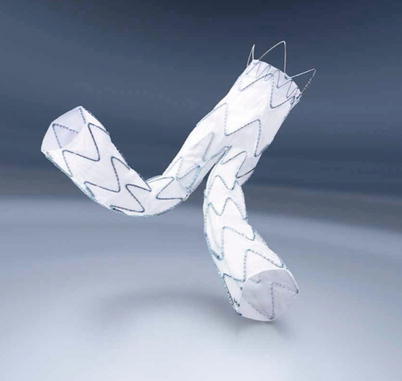
Fig. 34.2
Talent abdominal stent graft (Courtesy of Medtronic Italy Spa, Brescia, Italy)
Endurant Stent Graft (MedtronicVascular, Santa Rosa, CA)
This device features a polyester graft material externally supported by an electropolished nitinol stent structure attached to the graft by sutures (Fig. 34.3). An M-shaped proximal stent with anchoring hooks is designed to fix the endograft above the renal arteries. The hydrophilic, low-profile delivery system for the main body measures between 18F and 20F, while for the iliac extensions, the sizes are 14F and 16F. A tip-capture mechanism allows separate control-release deployment of the suprarenal stent. The Endurant endograft is loaded into a low-profile delivery system, with an outer diameter ranging from 18F to 20F for the main body and from 14F to 16F for the extenders. The delivery system features a tip-capture release mechanism to ensure accurate control of suprarenal stent deployment.
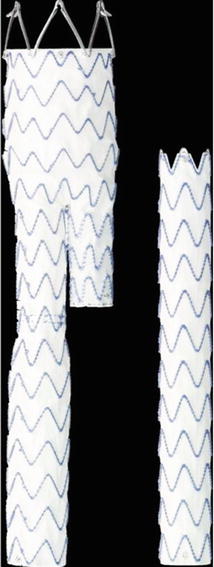
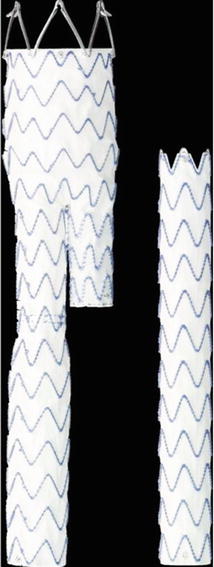
Fig. 34.3
Endurant stent graft (Courtesy of Medtronic Italy Spa, Brescia, Italy)
AneuRx Abdominal Stent Graft (MedtronicVascular, Santa Rosa, CA)
This is a modular endograft system that utilizes an exoskeleton of 1-cm self-expanding elements (Fig. 34.4). The self-expanding, thin-wall polyester graft material, supported by diamond-shaped elements, supplies high radial force for reliable and secure sealing without barbs, hooks, or balloons. To date, the AneuRx Abdominal Stent Graft has undergone eight modifications since the original clinical trial. The first-generation device consisted of a stiff-body design with a pre-reduced porosity graft material and a bullet delivery system. In 1998, the endograft flexibility was increased by changing the body of the endograft from a single 5-cm nitinol stent to a series of 1-cm diamond-shaped rings. Furthermore, the graft material was changed to a reduced porosity material. In 2002, the delivery system was changed to the Xpedient Delivery System with a tapered nose cone, allowing the device to be placed without a sheath. In 2004, the graft material was again changed to the Resilient graft material, which was associated with the greatest amount of sac shrinkage as compared to other contemporary graft materials [6]. In 2005, the Xcelerant Delivery System was added, allowing for easier deployment of the endograft. In 2006, the AneuRx AAAdvantage Stent Graft was offered, which added an extended aortic body of 4 cm, contoured stent rings, longer, larger, and flared iliac limbs to decrease the number of components required for repair and enhanced radiopaque markers. Finally, in 2008 a hydrophilic coating was added to the delivery system. Currently, the AneuRx AAAdvantage Stent Graft has a 21F delivery system and may be used to treat up to 26-mm aortic neck diameters that are 15 mm in length with <45° of angulation.
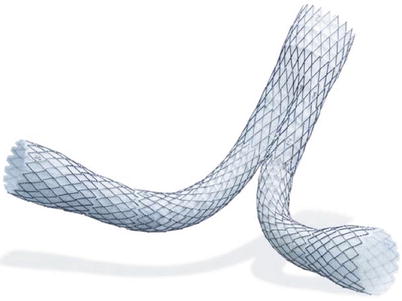
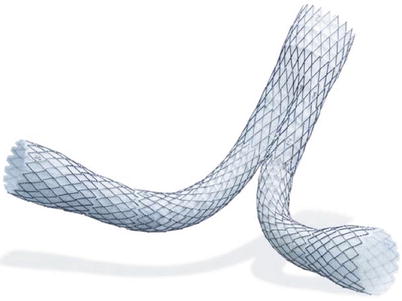
Fig. 34.4
AneuRx abdominal stent graft (Courtesy of Medtronic Italy Spa, Brescia, Italy)
Excluder Aortic Endograft (W.L. Gore & Associates, Flagstaff, AZ)
This device is a modular system covered with expanded polytetrafluoroethylene (ePTFE) and supported by a nitinol stent frame (Fig. 34.5). The endograft material is bonded to the nitinol frame and thus has no suture holes in the material. The microstructure in the original device permitted selective permeability of serous fluid in a subset of patients that could be associated with endotension [7, 8], but with the addition of a non-permeable, very thin, and highly durable fluoropolymer layer, this drawback was overcome [9, 10]. The endograft consists of a bifurcated main body with a single docking limb and assorted iliac limbs. Iliac and proximal extenders are also available. The main proximal portion of the main body has a scalloped end that is entirely covered with paired nitinol anchors for fixation set at 45°. There is no mechanism for suprarenal fixation. The proximal edge is identifiable by three gold markers designed to be placed immediately below the most inferior renal artery. The main body of the device is contained in an ePTFE jacket that is stitched with a single strand of ePTFE string. This is the mechanism for deployment: the string is pulled and the stitches are released, causing endograft deployment (proximal to distal). Rotational orientation is facilitated by different length markers on the ipsilateral and contralateral endograft sides. In the new C3 Delivery System, deployment is now a three-step maneuver, including the option of re-constraining and repositioning the device. If the position is believed to be too high or too low with regard to the renal arteries, the device can be easily adjusted to reach the ideal final location. Similarly, reorientation of the contralateral gate is possible, which makes cannulation easier and less time consuming. Currently, introducer sheaths are available in 30-cm length as 12F, 18F, and 20F sheaths. Main trunks with ipsilateral leg are available in 23-mm, 26-mm, and 28.5-mm diameters with 12-mm and 14.5-mm iliac diameters. The endoprosthetic length ranges from 12 to 18 cm in 2-cm increments. The recommended aortic neck diameter ranges from 19 to 28 mm. The ipsilateral iliac treatment diameter ranges from 10 to 13.5 mm. All these devices can be delivered via the 18F sheath except for the 31-mm endograft, which requires the 20F sheath. The contralateral endograft ranges from 12 to 20 mm in 2-mm increments and is available in 9.5-cm, 10-cm, 11.5-cm, 12-cm, 13.5-cm, and 14-cm length increments. The larger diameter contralateral leg endograft (16, 18, and 20 mm) can also be used as iliac extenders. The Excluder device contains flared iliac extenders to attach to aneurysmal iliac vessels. Dedicated iliac extenders range from 10 to 14.5 mm and are available in 7-cm length increments. The recommended iliac treatment diameters range from 8 to 18.5 mm. All may be delivered via the 12F sheath except for the 16-mm to 20-mm endograft, which requires an 18F sheath. Aortic extenders range 23, 26, and 28.5 mm and are available in 3.3-cm length increments. Their recommended aortic neck diameter is 19 to 26 mm in the United States, and a range of 19 to 29 mm has been used outside the United States.
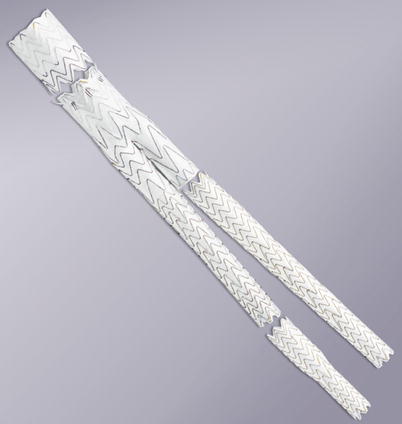
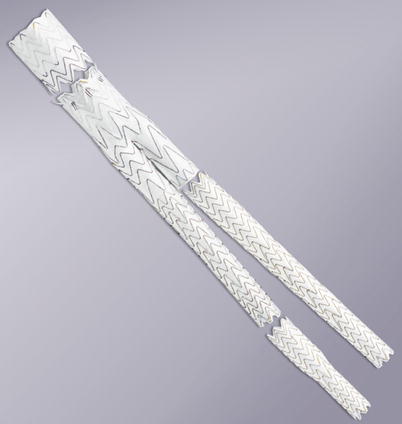
Fig. 34.5
Excluder aortic endograft (Courtesy of W.L. Gore & Associates, Inc., Flagstaff, AZ)
Anaconda AAA Stent Graft System (Vascutek, Scotland, UK)
The system is tri-modulare and is composed of a bifurcated body to which the ipsilateral and contralateral branch may engage (Fig. 34.6). A wide range of bodies and bifurcated iliac branches allows to treat patients with an aortic neck from 17.5 to 31 mm and iliac arteries from 8.5 to 21 mm. The tri-modulare system allows adapting the stent to the size and characteristic anatomy of each patient. The flexibility of the body is allowed by the fact that distally to the two rings of nitinol for the proximal anchorage, the prosthesis is supported by two other very flexible rings, which, while supporting the bifurcated body, will not impair flexibility. The end of the contralateral side has a thin support of nitinol and radiopaque markers to ensure an open and visible lumen during the procedure of cannulation. The iliac branches, straight and/or flared, are equipped with support rings and radiopaque markers for the correct positioning inside the main body. The rings of support are varied but independent of one another so as to ensure an excellent flexibility to the iliac branches and prevent kinking. The iliac branches can be used to treat isolated aneurysmal iliac arteries. The flexibility characterizes also the introduction and release system that allows placing the stent in vessels anatomically very tortuous. The proximal part of the aortic bifurcated body has two rings of nitinol supported by four pairs of hooks that ensure safe anchorage of the device.
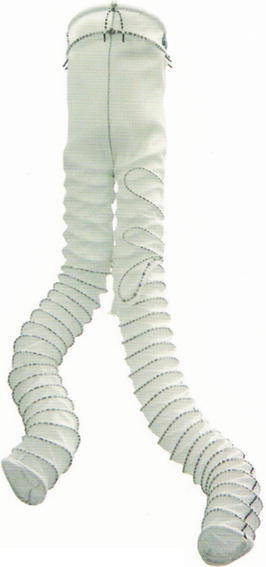
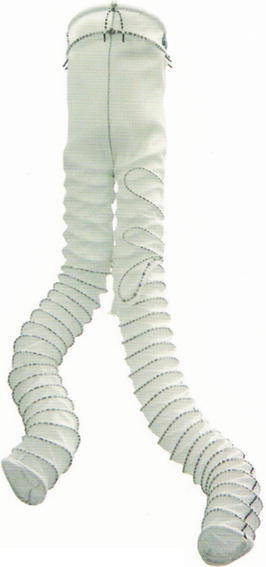
Fig. 34.6
Anaconda AAA stent graft system (Courtesy of Vascutek Ltd., Renfrewshire, Scotland)
AFX Endovascular AAA System (Endologix, Irvine, CA)
This Endovascular AAA System integrates anatomical fixation with an advanced delivery system and graft material technology (Fig. 34.7).
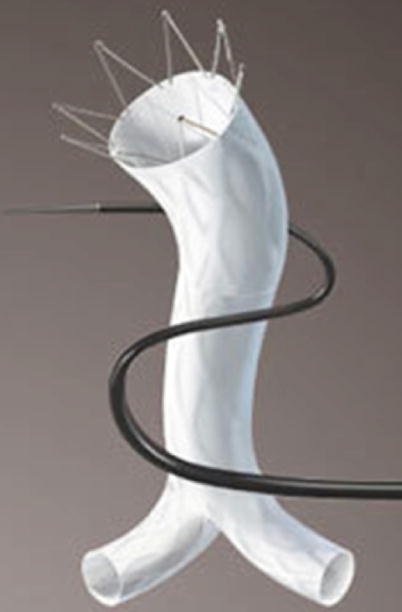
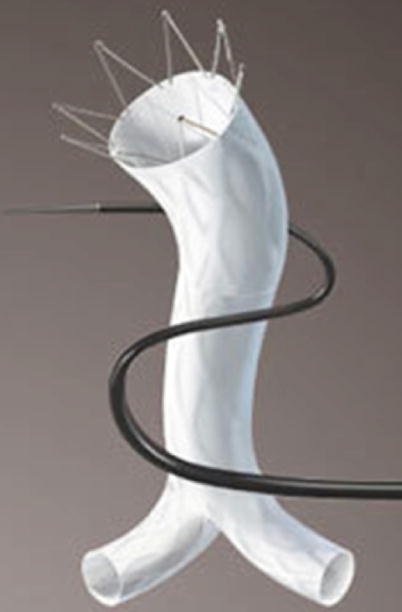
Fig. 34.7
AFX endovascular AAA system (Courtesy of Endologix, Inc., Irvine, CA)
Incraft Stent Graft System (Cordis Corporation, Florida, USA)
This new system was designed so that a few sizes will fit most vessels with respect to both diameters and length (Fig. 34.8). The modular components can be tailored during the implant procedure, with “in situ length adjustment” of the limb prostheses. This permits up to 2–3 cm of variability that can be achieved by varying the modular overlap length on each side reducing the risk of inadvertent coverage of the hypogastric orifice and, at the same time, allowing the maximum length of iliac fixation for migration prevention. The endograft design provides appropriate radial force over a relatively large range of diameters, so that four sizes can be used for aortic diameters ranging from 17 to 31 mm. Similarly, five different iliac limb diameters will accommodate iliac arteries ranging from 7 to 22 mm in size. Each iliac limb is supplied in four distinct lengths ranging from 8 to 14 cm, allowing a total aortoiliac coverage length between 13 and 21 cm. Therefore, a combination of 23 different modular components allows the operator to customize the device to the wide variety of AAA anatomy, with a significant reduction in the complexity of device selection, which is so critical in emergency settings. The Incraft device has a flared bare transrenal stent with 8–10 laser-cut barbs (size depending) located on the most cranial part of the stent for suprarenal fixation. The transrenal stent, the short segmented sealing infrarenal endoskeleton stent and the non-tilting deployment mechanism maximize the proximal seal, especially in the presence of a challenging aortic neck. The endograft is constructed of a seamless, woven polyester material supported by a series of laser-cut nitinol stents throughout its entire length. Such a design was chosen for kinking prevention, which has been associated with limb thrombosis. The Incraft stents are attached to the graft with a unique suture scheme to minimize micromotion between the stent and graft component, a mechanism believed to have a negative impact on endograft integrity with prior devices. Finally, one of the most innovative features of the device is the ultra-low profile: the integrated delivery system is 13F with a 14F (4.7 mm) outer diameter. The “catheter-like” flexibility and the elimination of sheath exchange minimize delivery problems, particularly in patients with small, diseased, and otherwise challenging access anatomy.
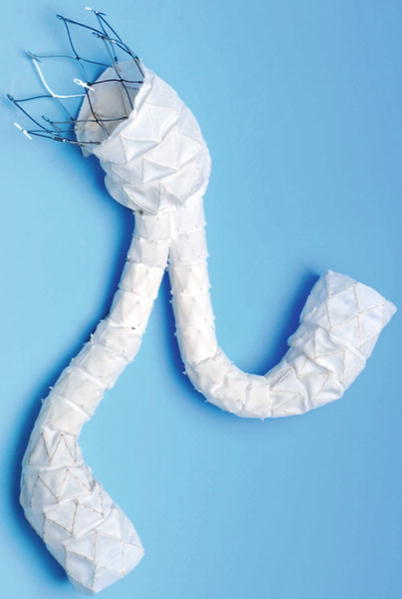
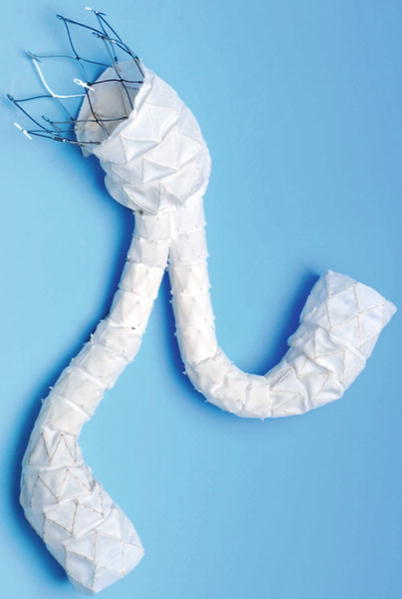
Fig. 34.8
Incraft stent graft system (Courtesy of Cordis Corp., Hialeah, FL)
Selection Criteria for EVAR
General accepted guidelines for EVAR include at least 10–15 mm of non-aneurysmal proximal neck, proximal neck diameter less than 30 mm, adequate access vessels (femoral/iliac arteries) at least 7 mm in diameter, minimal vessel calcification and thrombus, and a neck–body aneurysm angle ≤60°. The 2005 Excluder instructions for use recommend the following selection criteria: adequate iliac/femoral access, infrarenal aortic neck diameter ranging from 19 to 26 mm, minimum aortic neck length of 15 mm, proximal aortic neck angulation ≤60°, iliac artery treatment diameter ranging from 8 to 18.5 mm, and iliac distal vessel seal zone of at least 10 mm. Device delivery may be hindered by excessive tortuosity, calcification, or occlusive disease of femoral or iliac arteries. Additional considerations relate to the adequacy of collateral flow for occluded branches, such as an indispensable inferior mesenteric artery or accessory renal artery. The most common obstacle for EVAR is inadequate proximal anatomy. Self-expanding stents may be used more frequently to treat AAA with neck dilatation compared with balloon-expandable stents. An analysis comparing Excluder and the balloon-expandable Lifepath found this factor to be correlated with a higher rate of device migration seen in the Excluder device in this series [11]. A review from the EUROSTAR database analyzed 1,152 patients treated with EVAR having severe neck angulation (>60°) with Excluder, Talent, or Zenith endografts [12]. All three had acceptable outcomes in patients with severe neck angulation, with the Excluder and Zenith having increased risk for short-term type I endoleak and device migration and the Talent device having increased long-term risk of type I endoleak and secondary intervention. Favorable anatomic criteria allow use of any of the current commercially available endografts for EVAR. However, it has become apparent that certain features of proximal neck diameter, length, and angulation, or iliac anatomy characteristics favor some devices over another. The low-profile delivery is one such example. Also, the scalloped proximal edge makes precision delivery possible for tight proximal anatomy. A unique characteristic of the Excluder is its rapid deployment mechanism. This rapid deployment of a self-expanding device with proximal fixation in the setting of tortuosity and path rotation may lead to unexpected shortening and a more distal position than expected in inexperienced hands. Potential consequences of unexpected distal migration could include inadvertent hypogastric artery occlusion. Whittaker et al. found that this event occurred in 11 % of limbs studied with a shortening of graft path 1 cm or more [13]. Moreover, they noted that graft path shortening was affected more by anatomic factors than by Excluder device factors. Graft rotation was the most difficult pattern to predict preoperatively. Most patients will be assessed with three-dimensional reconstruction of multi-detector CT angiography (MDCTA). This has replaced previous modalities such as “road-map” angiogram or marker catheter as the best method for pre-intervention planning. Features such as tortuosity and rotation require special consideration in preoperative planning. Whittaker et al. reported that three-dimensional computer-aided measurement, planning, and simulation software was very accurate for preoperative planning with the Excluder device and more accurate than using marker catheter angiography [14]. Availability of several commercial devices with different properties with regard to proximal fixation, deployment accuracy, endograft flexibility, and size of introduction system enables to tailor the device selection according to each patient’s AAA anatomy. Zenith endografts were mainly used for short proximal necks in view of the suprarenal fixation of the bare stent with hooks and barbs. In addition, in view of the versatility of the Zenith Tri-Fab system in which the length of both limbs can be chosen after insertion of the main body, the endograft may be appropriate also in aneurysms where accurate length measurement proves difficult (e.g., angulation or short common iliac arteries). This feature is shared also by the Incraft Stent Graft System. The Talent endografts were initially used for proximal necks with a large diameter, but this advantage was lost when the Zenith equally featured larger proximal diameter sizes. Excluder endografts were preferred in patients with narrow and angulated iliac arteries because of flexible and thinner wall limbs. In July 2004, the Excluder underwent an important modification with the introduction of a lower porosity graft material. The Talent prosthesis was recently replaced by Medtronic with the Endurant. This new prosthesis, always with suprarenal attachment, has greater flexibility and conformability in the aortic vessel and requires thinner, dedicated introducers.
EVAR Procedure
The deployment technique for a two-piece modular device involves passage through one femoral artery of a sheath containing a body and the ipsilateral iliac limb. The superior end of the body is positioned just below the lowermost renal artery. The contralateral iliac limb is inserted through the opposite femoral artery and is overlapped with a short stump in the body of the device. The end result is a percutaneous Y-graft, with some important differences as compared to surgical grafts: the attachment sites in the infrarenal aortic neck, iliac arteries, and within the endograft are not sutured, and the aortic side branches are not ligated. Since the attachment sites are not hand sutured, they rely on the radial force of the stents to provide a hemostatic seal. Fixation at the attachment sites is also important to prevent endograft migration. Some manufacturers achieve this by adding hooks or barbs on the infrarenal stent. Some devices incorporate a bare suprarenal stent with or without hooks. This suprarenal stent may extend as high as the superior mesenteric artery.
Access-Related Issues
EVAR requires adequate vessel size in order to place the device and to exclude the aneurysm. Indeed, small external iliac arteries, vessel tortuosity, and heavily calcified vessels with aortoiliac occlusive disease make EVAR more difficult. For each of the currently available devices, the external iliac and common iliac arteries need a minimum diameter of 7 mm for the main device. Certain anatomical conditions will make access more difficult, but certain technical maneuvers can overcome these conditions.
Femoral Artery Access
Standard femoral artery access is achieved via a transverse or longitudinal incision. Use of small transverse incisions just below the inguinal ligament may be beneficial during EVAR. With this exposure, dissection just below the inguinal ligament gains access to the common femoral artery, typically in a relatively soft area of the artery. Furthermore, the femoral bifurcation is avoided, making control of the femoral artery easier and avoiding dissection of the profunda and superficial femoral arteries. When using this approach even in patients with heavily calcified vessels, it is usually possible to find a soft spot on the anterior wall of the femoral artery just distal to the inguinal ligament that can be used to puncture the artery. If the vessel is still heavily calcified, the inguinal ligament can be divided to allow access to the very distal external iliac artery. Once the femoral artery is dissected out, proximal and distal control of the vessel is obtained with vessel loops. Wire access is then obtained using the standard Seldinger technique. There is growing experience with a truly percutaneous approach using a technique, often referred to as the “pre-close technique.” With this technique, sutures are percutaneously placed before the insertion of the sheath and are used to close the femoral entry site at the end of the procedure. Using ultrasound guidance to confirm access to the common femoral artery, the artery is accessed and a 7F sheath is placed. A femoral arteriography is performed to confirm the sheath is within the common femoral artery. The 7F sheath is removed, and either a single 10F Prostar (Abbott Vascular, Santa Clara, CA) or two 6F ProGlides (Abbott Vascular, Santa Clara, CA), the second 45°–90° to the first, can be advanced over the guidewire with the sutures deployed within the vessel wall. Once the sutures are deployed, guidewire access is regained through the rapid-exchange port of the device. The suture device is then removed, and either an 11F or 16F sheath can be placed for hemostasis. This technique can be used with any of the currently available devices. When first learning this technique, it is best to avoid small, calcified vessels, as well as extremely tortuous vessels. A fully percutaneous procedure presents some advantages: less bleeding, reduced risk of femoral nerve injury, faster recovery, and, in most cases, earlier ambulation.
Aortic Angiogram
After femoral access is achieved, a marker pigtail angiography catheter is inserted into the sheath and advanced over a J-tipped guidewire up to the suprarenal aorta under fluoroscopic control. The guidewire is removed, and an aortogram is obtained with a pressure injector and recorded on the fluoroscopy screen with road-mapping technology. Aortic angiography with the marker catheter, which has radiopaque markers at 1-cm intervals, allows accurate measurement of the distance between the point of proposed placement of the proximal attachment system (below the lowest renal artery) and that of the distal attachment system (above the ipsilateral hypogastric artery). This serves to confirm preoperative MDCTA measurements and to assure that the appropriate endograft length had been selected.
Endograft Positioning and Deployment
Once guidewire access is obtained, before placing any large sheath or device, it is best to have a stiffer guidewire in place for the sheath and device to track. Stiff guidewire that are available to straighten out the vessels include the Amplatz Super Stiff (Cook Medical, Bloomington, IN), the Meier Wire (Boston Scientific Corporation, Natick, MA), and the Lunderquist (Cook Medical, Bloomington, IN). In vessels with extreme tortuosity and calcification, even advancing a stiff guidewire through a catheter can be difficult, with the stiff guidewire not advancing and the catheter coming back. This can sometimes be overcome with the use of a “buddy guidewire.” Initially, if a stiff guidewire does not pass through a catheter, a stiff Glidewire (Terumo Interventional Systems, Somerset, NJ) can be passed though the catheter, which will usually track easily. Leaving the stiff Glidewire within the catheter, a second guidewire – typically a Glidewire – can be placed within the sheath and into the descending thoracic aorta. A catheter is then advanced over the Glidewire. With the stiff Glidewire and catheter already in place, a stiffer guidewire, such as an Amplatz Super Stiff or a Lunderquist guidewire, can be advanced through the second catheter to straighten out the external iliac artery for placement of the device. Gradually increasing the size of small vessels bypassing dilators can be an adjunctive maneuver besides using a stiff guidewire for device placement. In some patients with severely diseased external iliac arteries or iliofemoral vessels of inadequate size, a conduit end-to-end sutured to the common iliac artery can be used to obtain adequate access for placement of the endograft. A 10-mm Dacron graft allows for passage of all devices. This can be extremely beneficial in preventing damage and/or rupture of the external iliac artery. Once the endograft sheath is introduced over the stiff guidewire and advanced under fluoroscopic control up the iliac segment and well into the aneurysm, the patients receive 5,000 IU intravenous heparin. With the guidewire left in place, the angiography catheter is removed, and the endovascular deployment assembly (trunk-ipsilateral component) is prepared for insertion into the sheath. The assembly is loaded over the guidewire and advanced under fluoroscopic control up the introducer sheath to the aortic position. The introducer sheath, initially advanced into the aorta, is backed into the external iliac artery, which permits the endovascular prosthesis portion of the assembly to be positioned within the aneurysm. The proximal attachment system is positioned just below the renal arteries, the distal attachment system is positioned in the common iliac artery, and the trunk-ipsilateral component of the endograft is deployed. In order to maximize and improve proximal fixation, there are some maneuvers that can be used. The first of these is magnification views and appropriate angulation of the fluoroscopy unit. Typically, adjustment of the image intensifier in the caudal direction will open up the infrarenal neck and show its true length. Typically, the infrarenal aortic neck begins to angle anteriorly following the course of the lumbar spine. Adjusting the image intensifier 10º to 20º is usually sufficient to open up the proximal neck. After guidewire cannulation of the contralateral “gate,” the contralateral leg of the endograft is then inserted in the opposite common femoral artery and advanced until overlap is obtained on the short contralateral leg of the aortic endograft placed in the abdominal aorta. An elastomeric balloon is then used to secure the endograft to the proximal aortic neck. This step is usually performed during cardiac pacing at high frequency to reduce blood pressure and to avoid that the displacing forces of the high aortic flow may push the inflated balloon causing downward migration of the endograft from its position. New balloons, such as the Tri-Lobe (Fig. 34.9), allow blood flow through the device itself and do not require temporary pacing. A pigtail angiography catheter is then reinserted over the guidewire into the suprarenal aorta, and a completion angiogram is obtained. Technical success is achieved when there has been successful access to the arterial system using a remote site, successful deployment of the endograft with secure proximal and distal fixation, absence of either a type I or type III endoleak (see complications), and a patent endograft without significant twist, kinks, or obstruction by intraoperative measurements [13–15]. The sheath and guidewire are removed, and the access site is sutured or hemostasis is obtained with the pre-close technique.
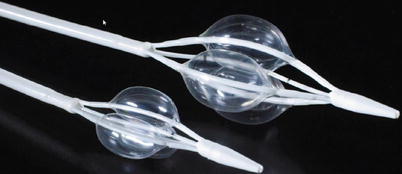
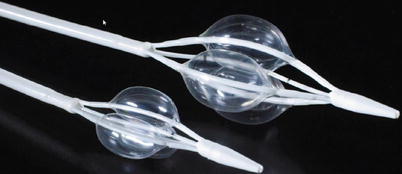
Fig. 34.9
GORE tri-lobe balloon catheter (Courtesy of W.L. Gore & Associates, Inc., Flagstaff, AZ)
Vascular Anatomy Issues
Proximal Aortic Neck
It has been demonstrated that placing the endograft as close to the renal arteries as possible at the original implantation significantly decreases the risk of migration. There are certainly some conditions that are going to increase the risk of migration or proximal type I endoleaks. These risks include a short angled neck as well as a reverse funnel neck. Currently, all devices are approved for use with a proximal neck length of 15 mm except the Talent abdominal endograft, which is approved for use with a 10-mm neck. The technique and precision of device implantation, along with patient selection are significant factors in predisposing patients to subsequent adverse events in the future, especially migration. Migration has been reported with all devices with an incidence between 2.3 and 9.5 % in clinical trials with a follow-up of 1–4 years [16–19]. When evaluating device-specific outcomes, Ouriel et al. found no significant difference among various devices regarding the risk of migration, which ranged from 0 % with the Talent and Ancure to 8.2 % with the Zenith [18]. In a more recent study, Abbruzzese et al. evaluated 177 Zenith, 111 Excluder, and 277 AneuRx endografts [19]. In the study, 39.3 % of devices were placed outside of at least one of the instruction for use parameters. Mean follow-up was 30 ± 21 months and was significantly shorter for the Zenith (20 months) compared to the Excluder (35 months) and AneuRx (31 months), respectively. Overall actuarial 5-year freedom from aneurysm-related death, reintervention, and graft-related event rates were similar among the three devices.
Iliac Artery Aneurysms
In patients with aortoiliac aneurysms, there are three options available to the implanting physician. For ectatic iliac vessels, use of flared limbs has simplified the repair of aneurysms with large common iliac arteries. Flared limbs as large as 24 mm allow vessels up to 20–22 mm to be safely treated without the need of coil embolization of the internal iliac artery. This is possible with both the AneuRx AAAdvantage and Talent abdominal endografts. However, when using these flared limbs, it is still important to try to achieve between 20 and 25 mm of seal to prevent a retrograde type I endoleak. With aneurysmal common iliac arteries, it is usually safer to occlude the hypogastric artery by coil embolization or vascular plug deployment and bring the endograft into the external iliac artery (Fig. 34.10).
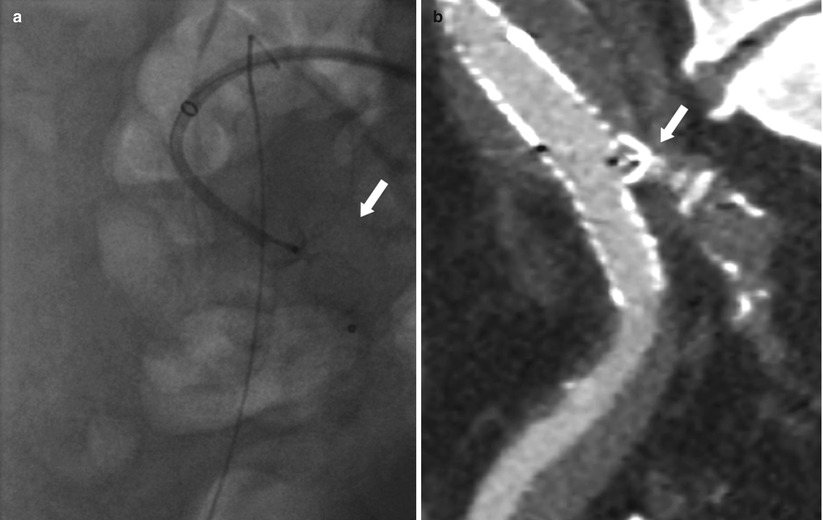
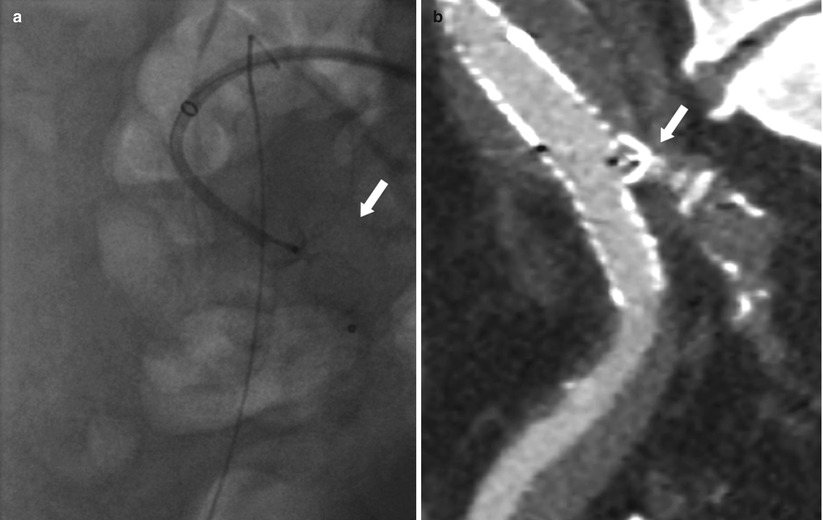
Fig. 34.10
Embolization of the hypogastric artery before EVAR. (a) Deployment of an Amplatzer vascular plug (arrow) in the right hypogastric artery. (b) MDCTA (multiplanar reconstruction) showing final result after EVAR. Note the deployed Amplatzer vascular plug (arrow) with complete occlusion of the right hypogastric artery and the endograft leg that was brought into the external iliac artery
Complications Following EVAR
Migration
As the attachment sites are not sutured, there may be movements at the aortic or iliac attachments or at points of connection within modular endografts. Device migration is defined as any report of postprocedure device movement. These migrations include reports of migrations of the trunk-ipsilateral, contralateral leg, and extender components. It is well known that preprocedure planning, which includes appropriate patient anatomical measurements and device selection, will minimize migration events. Even when placing the device as close to the renal arteries as possible during initial deployment, there is continued risk of migration, especially if there is a short aortic neck, significant neck disease, including thrombus and calcification, neck angulation, and adverse neck contour, such as a reverse funnel neck [18–21]. However, it is important to remember that endograft relies on three points of fixation, including the proximal aortic neck and the right and left common iliac arteries. In vivo animal studies have shown that by maximizing the distal iliac fixation, the amount of force required to displace the endograft is increased by 67 %. This has been demonstrated with both infrarenal and suprarenal devices with no significant improvement when hooks were present [22–25].
Endoleaks
The term endoleak was first used in 1996 and defines the persistent perfusion of the aneurysm sac after EVAR due to incomplete sealing. The presence of an endoleak implies failure to exclude the aneurysm or a vessel segment. A leak can appear during the first 30 days after implantation. This type of leak is called “primary endoleak.” “Secondary endoleak” is one that occurs after 30 days. Persistent blood flow within the aneurysm sac eventually may result in aneurysm rupture despite endovascular repair. Review of EUROSTAR data suggested that the presence of an endoleak can predict rupture, since 69 % of patients whose aneurysm ruptured following EVAR had a preexisting endoleak [26]. The incidence of endoleak varies from 10 to 50 %, and its treatment depends on the type, site, and size of the endoleak.
Endoleak Classification
The anatomic classification of endoleaks is based upon the source of the inflow into the aneurysm sac, regardless of the number and type of other vessels involved in the outflow. The initial definition differentiated between four types of endoleak, but it was expanded in 1999 to include endotension (type V) (Table 34.1).
Table 34.1
Endoleak classification
Types | Mechanism |
---|---|
I | Flow originates from ineffective endograft seal at fixation zones |
A | Proximal |
B | Distal |
C | Iliac occluded |
II | Branch vessel retrograde flow |
A | Single vessel (simple) |
B | Two or more vessels creating a circuit (complex) |
III | Flow results from structural endograft failure |
A | Junctional separation (modular devices) |
B | Endograft fracture or holes |
– Minor (<2 mm) | |
– Major (≥2 mm) | |
IV | Endograft fabric porosity (<30 days after endograft implantation) |
V | Endotension |
Type I
These endoleaks are caused by failure of the circumferential seal at the fixation points of either the proximal (type IA) or distal (type IB) end of the endograft. Type IC endoleak is due to non-occluded iliac artery in patients with aorto-mono-iliac grafts and fem–fem bypass. With type I endoleaks, the aneurysm is perfused directly from the aorta or iliac arteries (inflows). These leaks usually communicate through a channel (sometimes multiple channels) with the aneurysm sac. There are several outflow vessels, mainly lumbar arteries and inferior mesenteric artery that communicate with the channel and or the sac. The pressure within a type I leak is systemic. The tension on the aortic wall remains high. Causes of primary type I endoleaks include inappropriate anatomy, such as a significantly angulated neck, severe calcification/plaque at the proximal or distal landing zones, a noncircular landing zone, endograft malpositioning and under-dilation, and endograft type. Secondary type I endoleaks can be due to aneurysm remodeling, resulting in endograft migration, progressive dilatation of the proximal neck, design and dimensions of endograft, or unfavorable infrarenal necks, including the conically shaped neck and neck shorter than 15 mm. Treatment is mandatory because the aneurysm sac is at systemic pressure. Untreated type I endoleaks are at high risk of aneurysm rupture. Indeed, a rupture rate of 3.37 % has been reported [27]. Treatment requires satisfactory circumferential apposition between the endoluminal surface of the vessel and the external aspect of the endograft. Gentle expansion of the device with a compliant “molding” balloon will seal the majority of primary type I leaks. Occasionally, despite prolonged molding, the leak will persist. A giant Palmaz stent may then be used to prevent recoil and improve stent apposition to the proximal neck. If this procedure fails and the leak is significant, a limited laparotomy will enable an external band to be placed around the proximal neck, without opening the aneurysm sac. Open conversion is occasionally required and was more frequent in the past for very large endoleaks due to the migration of home-made devices. If the endoleak is due to endograft malposition or moderate distal migration, the endograft is extended to increase the length of neck contact or the distal seal in the case of a leaking limb. Hence, a proximal aortic cuff or a graft extension limb may be required, and these are generally oversized by 10–20 % to prevent further endoleaks.
Type II
This type of endoleaks corresponds to the retrograde filling of the aneurysm, mainly from lumbar arteries and/or inferior mesenteric artery but also, in rare situations, from sacral, gonadal, accessory renal artery arteries, or iliac artery branches (Fig. 34.11). The leak always communicates with another “outflow” vessel. These are the commonest endoleaks, affecting up to 43 % of cases, and may be associated with aneurysm expansion and rupture. However, this risk is much less than with the type I and III endoleaks (0.5 % vs. 3.4 %) [26, 28]. Of note, they are associated with a significant rate (up to 40 %) of spontaneous closure. The current consensus is that a type II endoleak in the setting of a shrinking aneurysm can generally be followed without the need of immediate intervention. Treatment is required only for endoleaks that persist for more than a year in an aneurysm of increasing size. Intervention is usually by percutaneous embolization, either by a transarterial or a translumbar route. Percutaneous CT-guided or ultrasound-guided thrombin injection in appropriate patients can be a useful treatment option and should be considered when the more conventional methods are difficult [29, 30]. In some instances, coil or plug embolization of feeding vessels before endograft deployment is a viable option for type II endoleak prevention [31] (Fig. 34.12).
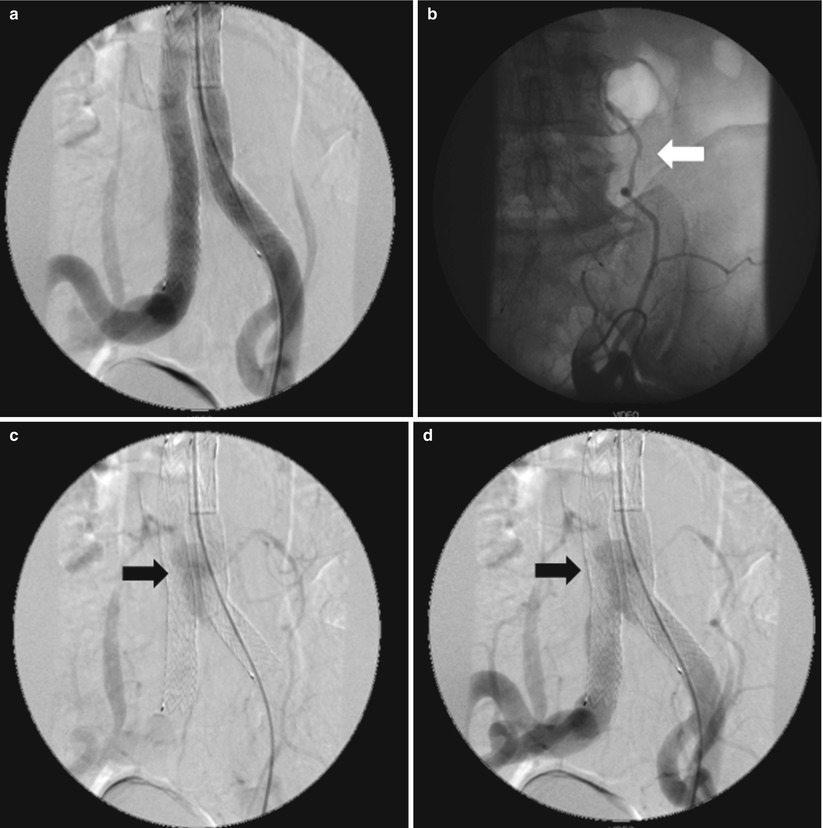
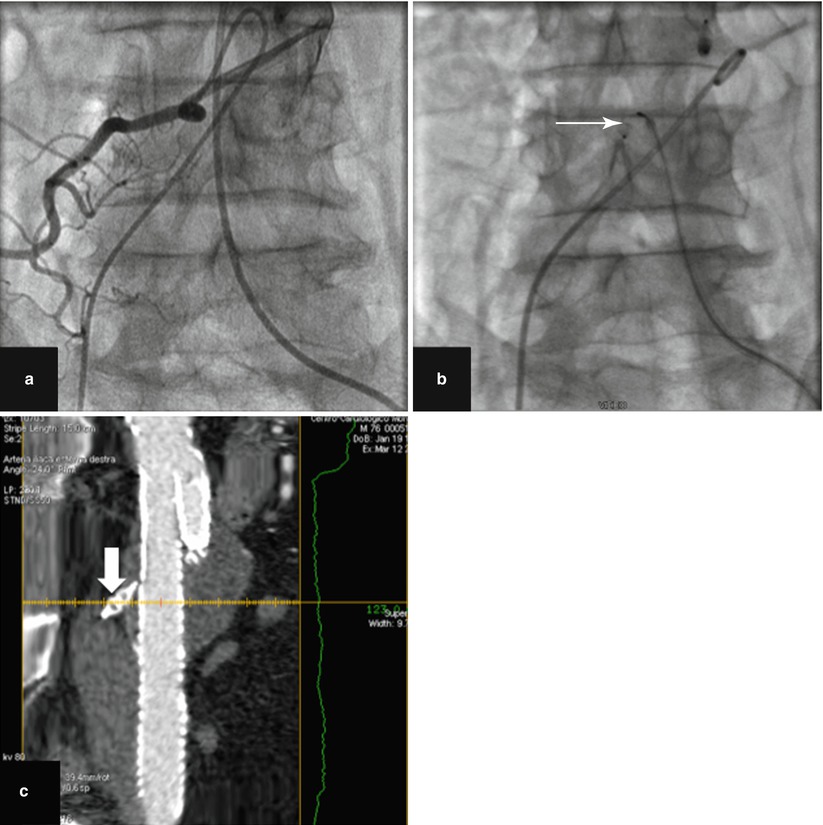
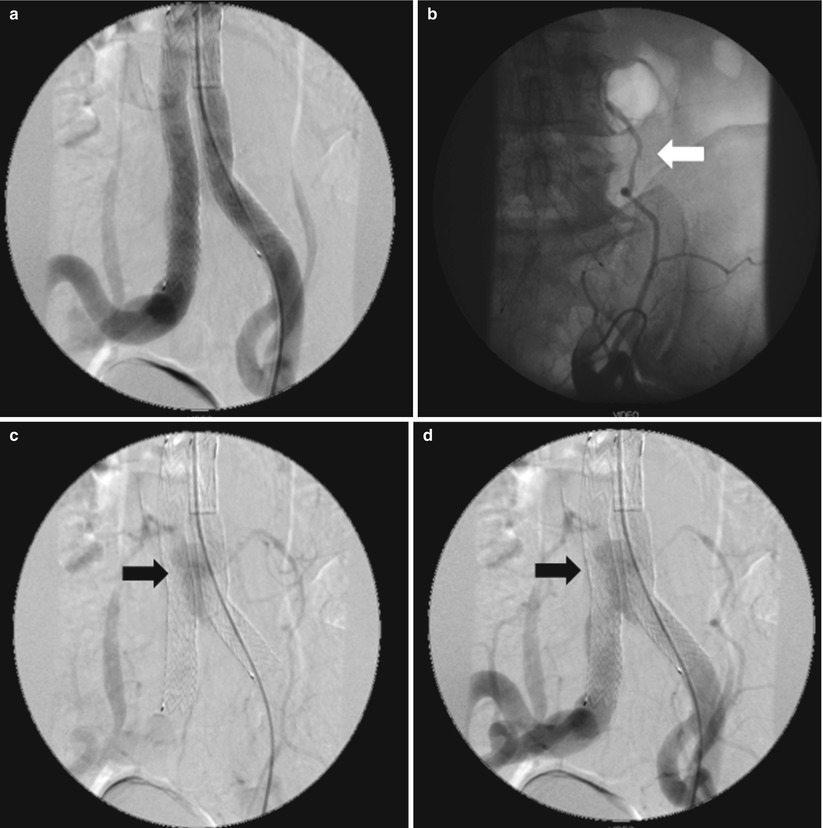
Fig. 34.11
Follow-up angiography after EVAR in a patient with type II endoleak. (a) Early-phase subtraction angiography showing patency and correct position of the endograft. (b) Selective angiography of the feeding collateral vessel (arrow) originating from the external left iliac artery. (c–d) Late-phase subtraction angiography showing the endoleak (arrows)
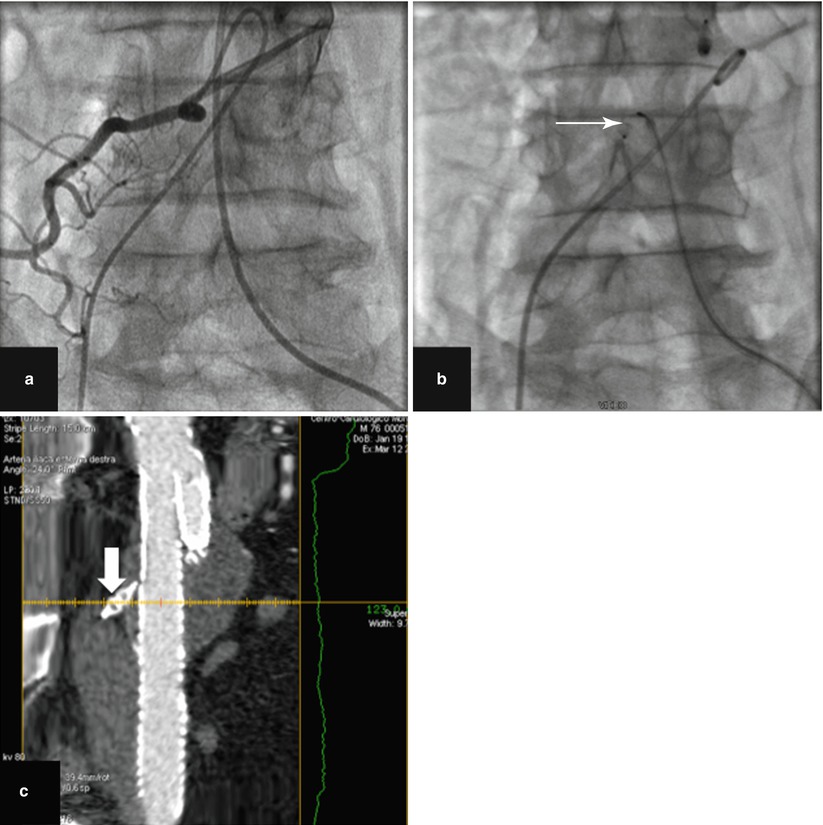
Fig. 34.12
Embolization of a large lumbar artery (a) selective angiography before EVAR. (b) Amplatzer vascular plug deployment (arrow). (c) MDCTA (multiplanar reconstruction) showing final result after EVAR. Note the deployed Amplatzer vascular plug (arrow) with complete occlusion of the lumbar artery
Type III
These endoleaks are caused by structural failure of the endograft due to separation of its modular components (type IIIA) or a tear in the graft material (type IIIB) (Fig. 34.13). As in the case of type I endoleaks, type III endoleaks represent direct perfusion of the aneurysm sac at systemic pressure and have a relatively high rupture rate. Primary type IIIA endoleaks are readily apparent during the initial procedure, but it can be difficult to differentiate them from type I endoleaks. They are usually caused by inadequate overlapping of the junctional sections of a modular endograft. Rarely, the cause may be defects in the graft material. Primary type IIIA leaks almost always resolve after balloon molding of the junction(s). If there is insufficient overlap of the components, an additional component may be required. If the endoleak is from the main body of the endograft or at the flow divider, a second endograft should be deployed within the first. Use of an aorto-mono-iliac device and a surgical fem–fem crossover helps to reduce the total amount of graft material. If no suitable graft is available, open surgical conversion must be considered. Delayed type IIIA endoleaks were seen commonly in the first-generation devices due to a short overlap between the main body and the limb [32]. They are generally due to sac remodeling, causing the graft components to separate, or to mechanical stress causing stent and fabric failure. They are very dangerous, since there is acute repressurization of the sac, and should be treated aggressively as primary type I leaks, accepting that there is a significant open conversion rate [26].
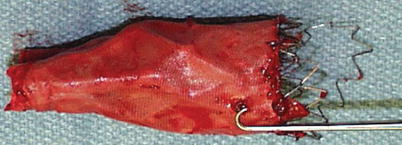
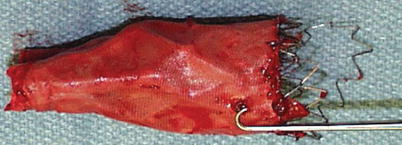
Fig. 34.13
Explanted endograft from a patient with type IIIB endoleak. Note the structural defect and a hole in the graft fabric
Type IV
Type IV endoleaks are caused by porosity of the endograft fabric during the primary procedure and are seen at the time of device implantation, as a faint blush on the postimplantation angiogram, when patients are fully anticoagulated. They were reported almost exclusively with the first-generation devices and seal spontaneously. Rupture has not been reported.
Type V
This type of endoleak is caused by continued expansion of the aneurysm sac in the absence of an endoleak visible on conventional imaging. The term “endotension” has also been coined for this phenomenon, which is thought to reflect the continued pressurization of the aneurysm sac. It is important to exclude the presence of a subtle endoleak by further investigation such as contrast-enhanced ultrasound. If found, this needs to be treated as described previously. In some cases, the expansion may be due to ultrafiltration through first-generation ePTFE endografts, resulting in the formation of a hygroma (Fig. 34.14), or to tiny endoleaks through suture holes in the endograft material. Sac expansion due to ultrafiltration of serous material can be left untreated even if rupture occurs. However, the inside of the endograft can be relined with a second device [33], and open surgical conversion remains a viable option (Fig. 34.15).
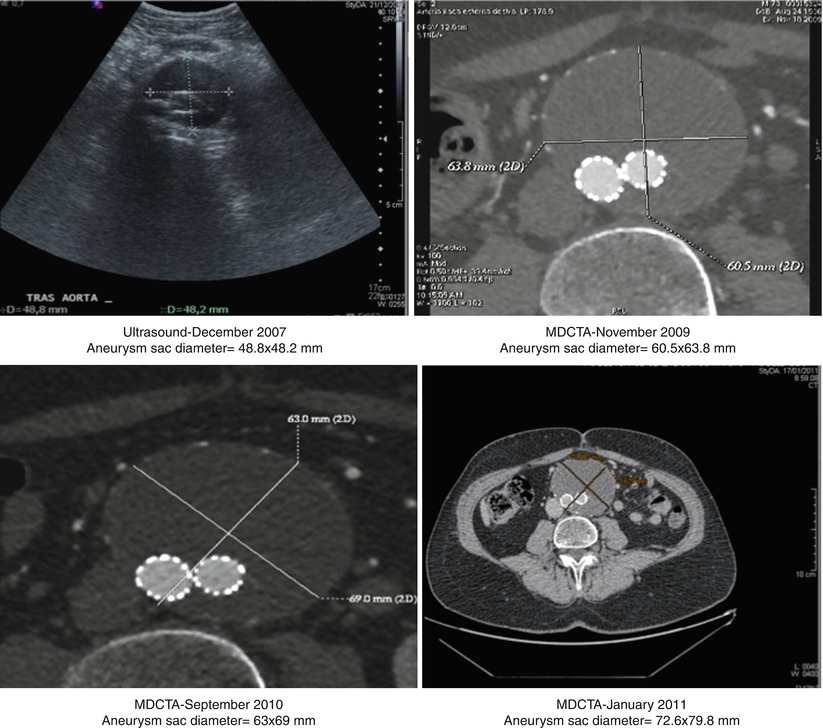
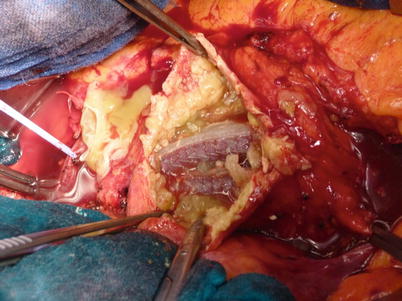
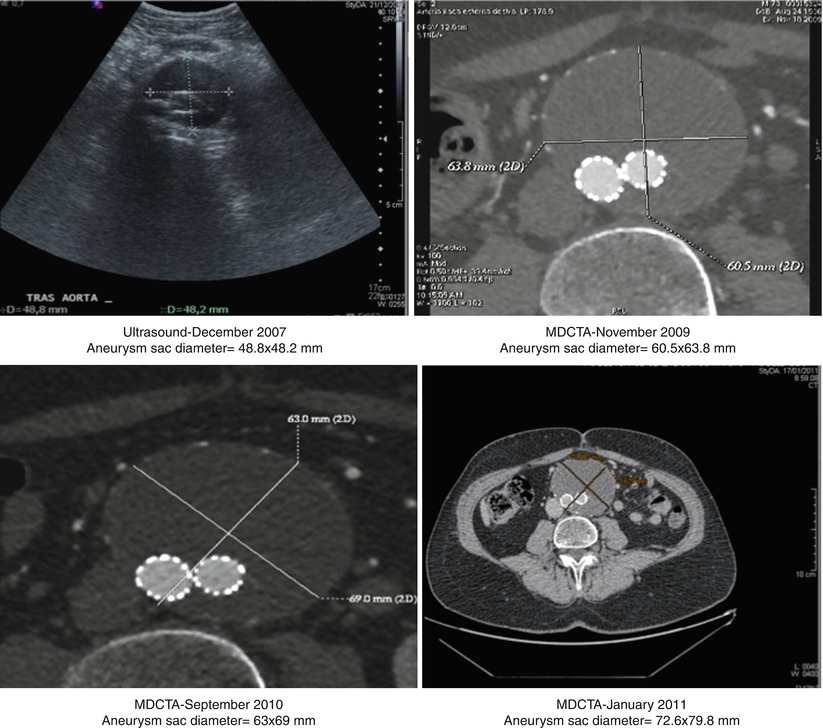
Fig. 34.14
Progressive expansion over time of the aneurysm sac in a patient treated with a first-generation Excluder endograft
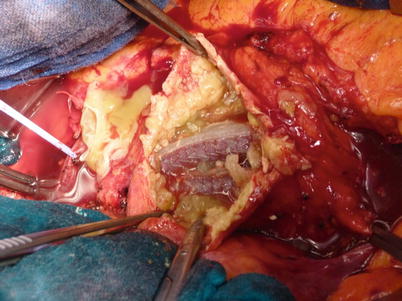
Fig. 34.15
Operating room picture during open surgical conversion (Same patient of Fig. 34.14). Note the proteinaceous material deposited inside the aneurysm sac indicative of hygroma formation
Endoleak Diagnosis
Contrast MDCTA is considered the imaging technique of choice for endoleak detection. Indeed, MDCTA is reported to be superior to aortography for the demonstration of small leaks. However, selective angiography is superior to MDCTA for the detection of inflow and outflow vessels (Fig. 34.11b). Deceitful MDCTA images, including calcifications, contrast within the folds of unsupported portions of the endograft, and residual contrast inside the aneurysm sac from the initial procedure may erroneously suggest the presence of endoleaks when noninvasive angiographic follow-up is obtained within 1–3 days after implantation. Of note, “pseudo-endoleaks” are seen in up to 57 % of patients.
Color Doppler ultrasound (CDUS) is a noninvasive and cost-effective imaging modality that can be used for endoleak assessment. It is highly dependent on the operator and has limitations in obese patients and in those with excessive bowel gas. Patients should be evaluated after 5–6 h fasting, in the supine and lateral position. The aorta is evaluated both transversally and longitudinally. A leak is suspected when a reproducible color and Doppler signal inside the aneurysm is visualized. Variable success is reported for the detection and localization of the source of endoleaks with ultrasound, depending on technical factors, imaging protocol, and image quality. Overall, the reported sensitivity for endoleak detection ranges between 12 and 100 %, with specificities ranging between 74 and 99 % [34]. Although these noninvasive techniques are reliable in detecting an endoleak, the characterization and type of endoleak, as well as endovascular treatment planning can still be difficult.
Structural Failure and Graft Distortion
Metals subjected to aortic pulsation pressures may fracture. The iliac limbs of the device may also become distorted and angulated within the residual space of the aneurysm.
Non-endoleak-Related Complications
These adverse events during or after EVAR can be categorized as complications owing to surgical exposure of the access arteries or the percutaneous approach, ischemic complications owing to intentional or inadvertent clot embolization or covering of an aortic side branch, stenosis or occlusion of an endograft limb, infection of the endograft or aneurysm sac, and contrast-induced nephropathy.
Local Wound Complications in the Groin
Local wound complications following surgical exposure of the access arteries include groin hematoma, infection, or lymphocele. Their reported incidence is 1–10 % [35]. In some cases, CDUS or MDCTA evaluation may be needed to evaluate the extent of the lesion. Clinical surveillance with or without medical treatment or surgical repair are mostly enough for definitive treatment.
Access Artery Injury
Arterial thrombosis, dissection, or pseudoaneurysm formation can occur in up to 3 % of EVAR procedures. Besides correct surgical and interventional skill, a thorough preoperative CDUS or MDCTA evaluation of the common femoral and iliac arteries with special attention to access vessel diameter, tortuosity, and degree of calcification is mandatory. The introduction of a stiff and large catheter system in the presence of small and heavily calcified iliofemoral arteries can induce vessel wall dissection or even perforation. In case of a postprocedural groin pseudoaneurysm, CDUS-guided thrombin injection is not always successful as the pseudoaneurysm neck can be too large, making surgical repair the only definitive treatment option.
Ischemic Complications
Ischemic complications may occur immediately after EVAR. They can be due to thrombus formation and embolization into aortic side branches and include colonic, renal, and pelvic ischemia. Another cause of ischemia is endograft misplacement and partial or complete covering of an aortic or iliac side branch, resulting in renal or pelvic ischemia.
Colonic Ischemia
Bowel ischemia occurs in 1–3 % of cases after open aortic aneurysm repair, and the incidence seems to be in the same range for EVAR [36, 37]. Postoperative bowel ischemia after aortic aneurysm repair still remains a serious complication with a mortality rate of 50 % within 1 month. However, the pathophysiological mechanisms of colonic ischemia most probably differ in open versus endovascular repair. Whereas interruption of the inferior mesenteric or iliac arteries has been suggested to be the cause of bowel ischemia in open procedures, the same mechanism does not seem to be important for EVAR. Zhang et al. suggested that thrombotic deposits and atheroma in the suprarenal aorta may play a role in major bowel ischemia in EVAR [38]. Thrombus and atheromatous material may be dislodged while the proximal part of the endograft is being positioned, deployed, and balloon dilated just below the renal arteries. These maneuvers can induce upstream flushing of mural clot or atherosclerotic debris into the superior mesenteric artery. They may also migrate into the renal, inferior mesenteric, and internal iliac and lower-limb circulation resulting in segmental, skipped, or patchy ischemia of the embolized areas. This embolization mechanism may also explain why bowel ischemia after EVAR mostly presents as multifocal patchy ischemia. This type of bowel ischemia is not seen after open repair, most probably because suprarenal aortic and ostial inferior mesenteric arterial clamping during surgery makes distal embolization unlikely. These observations underline the importance of a careful preprocedural evaluation of the proximal aneurysmal neck and the need to identify thrombus and atheroma that may make patients poor candidates for EVAR.
Spinal Cord Ischemia
Spinal cord ischemia after EVAR is very rare. Indeed, in the EUROSTAR database, an incidence of 0.21 % in 2,862 patients has been reported [39]. The mechanism is not completely understood, but atheromatous embolization and interruption of collateral circulation from lumbar and internal iliac arteries together with a variable anatomy of the artery of Adamkiewicz seem to be the most important contributing factors. The treatment is the same as for paraplegia after thoracoabdominal EVAR or open repair and consists in cerebrospinal fluid drainage and, if indicated, recanalization of occluded collateral arteries like the internal iliac artery [40–44].
Renal Artery Occlusion
Inaccurate endograft placement with partial or total coverage of one or both renal arteries occurs in less than 5 % of cases [45] and may be due to lack of high-quality imaging technology for guiding the EVAR procedure and limited experience of the endovascular team. In their early learning curve with EVAR, Kalliafas et al. described renal artery occlusion in 5 of 204 patients, resulting in renal failure with chronic hemodialysis need in two of them [46]. If misplacement is detected during EVAR, a bailout attempt can be made, using a pull-down maneuver with an inflated angioplasty balloon or by tugging caudally on a guidewire placed across the endograft bifurcation and exteriorized from both femoral arteries. Using this technique, Görich et al. could move the stent graft from 5 to 27 mm more distally [47]. It is noteworthy that these authors did not use this bailout technique on endografts with barbs at the proximal part of the suprarenal stent. Finally, in case of partial coverage of one renal artery, stent placement in the renal artery with slight protrusion of the stent over the proximal part of the endograft into the aorta can also be used to manage this complication [48]. Rarely, renal ischemia may also occur when the top of the endograft main body needs to be placed close to the main renal artery in the presence of an accessory renal artery originating from the infrarenal neck (Fig. 34.16).
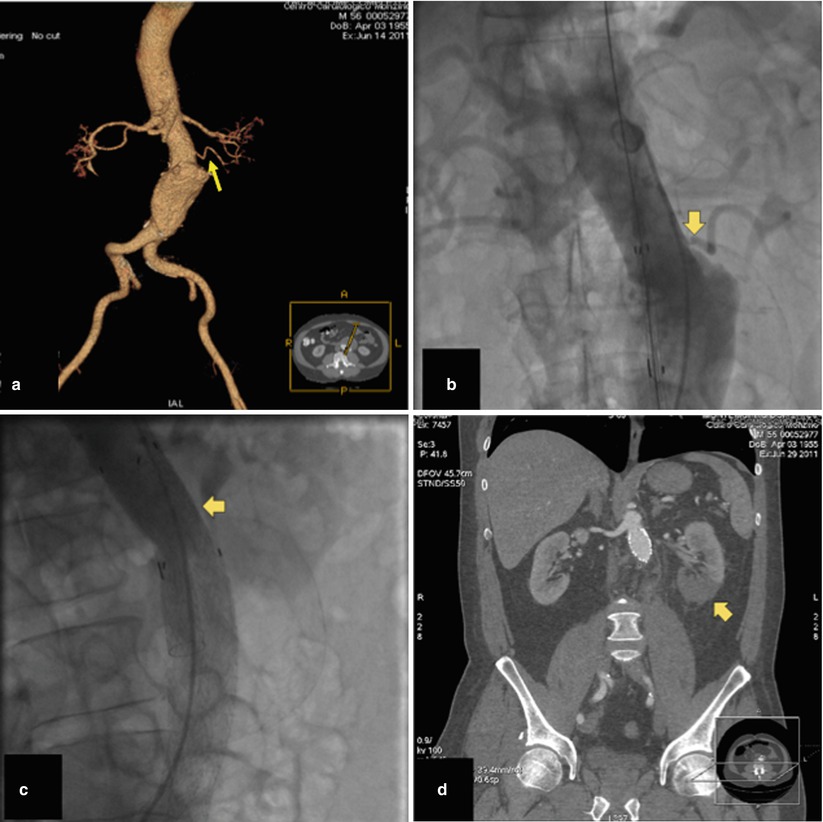
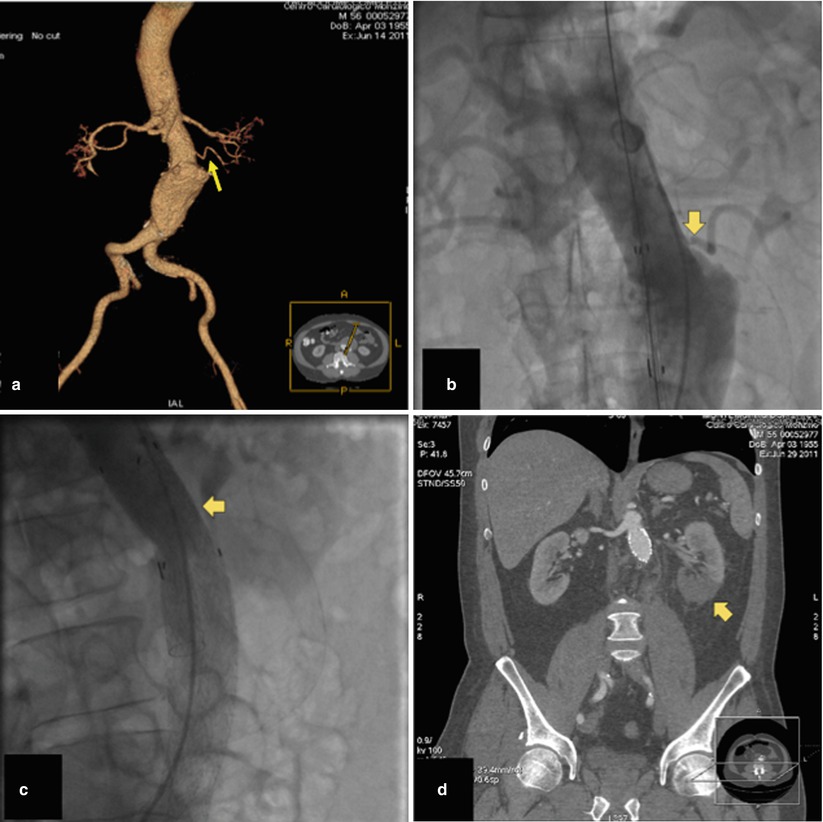
Fig. 34.16
Volume-rendering MDCTA (a) and invasive angiography (b) showing a left accessory renal artery (arrows). (c) The endograft main body was deployed close to the main renal artery occluding the accessory renal artery (arrow), and (d) multiplanar reconstruction MDCTA performed 3 days after EVAR showed infarct of the lower renal pole (arrow)
Early and Late Limb Occlusion After EVAR
Endograft limb thrombosis is a known complication of EVAR, especially in unsupported endografts. Indeed, it can occur in as many as 40 % of cases treated with this type of devices [49, 50]. The underlying mechanism is most frequently kinking of the unsupported endograft limb. Second-generation supported endografts perform significantly better with regard to limb thrombosis and have shown a significantly lower rate of limb occlusion, ranging between 0 and 5 % [51]. Most of the limb thrombotic events occur within the first 2 months after EVAR, and the underlying causes are endograft kinking and extension of the small-diameter endograft into the external iliac artery [52, 53]. Recently, it has been demonstrated that limb occlusion may also occur 4–5 years after EVAR [54]. The mechanism of late limb occlusion can be migration and dislocation of an endograft component causing major hemodynamic turbulence and, eventually, limb or entire endograft thrombosis. Treatment of limb occlusion includes various surgical and endovascular revascularization techniques. The best treatment option depends on the patient’s general status as well as on specific endograft and excluded aneurysm changes.
In contrast to limb thrombosis, incidentally found mural thrombotic deposits are much more frequent in both first-generation endografts (20 %) and second-generation supported endografts (17–33 %) [55]. A circumferential layer of thrombotic material has been observed more in the Zenith endografts than in the Excluder endografts, but they are clinically silent and are not associated with potential endograft thrombosis or distal embolization (Fig. 34.17). Additionally, there is no difference in survival among patients presenting with mural deposits in their endograft compared with patients without this thrombotic layer. Based on these observations, additional treatment in the form of relining the endograft with another endograft or administering any type of anticoagulant therapy cannot be recommended.
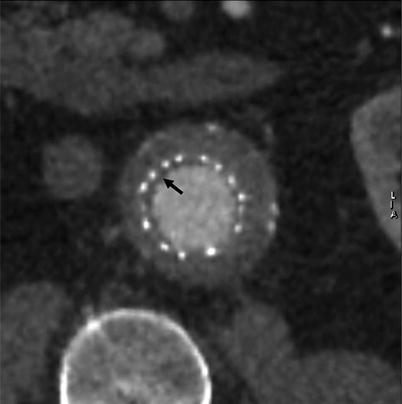
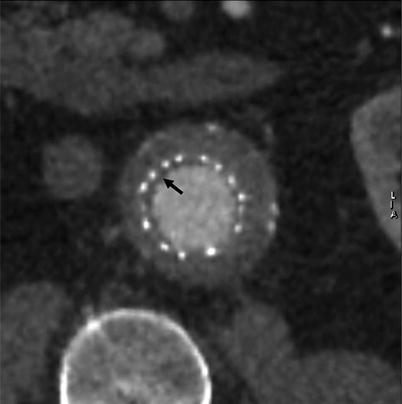
Fig. 34.17
MDCTA (short-axis multiplanar reconstruction) at 6-month follow-up showing a thin circumferential layer of thrombotic material (arrow) at the proximal end of an Excluder endograft main body
Infection
The incidence of endograft infection is 0.5–1 %. If untreated, infection can result in generalized sepsis and death [56]. There are multiple causes of this complication. Endograft contamination during EVAR seems to be the source of early infection. Secondary infection from a remote source is another pathophysiological mechanism. van den Berg et al. reported an endograft infection following septic complication of a kidney stone 1 year after EVAR [57]. Another case of endograft infection after EVAR has been reported in a patient who underwent an appendectomy for appendicitis and peri-appendicular abscess formation 1 month prior to EVAR. Another cause of infection is an aortoenteric fistula. Multiple mechanisms of aortoenteric fistula creation have been described and include endograft migration, erosion of the aorta and duodenum by embolization coils, fabric rupture, inflammatory nature of the aneurysm, and bacterial aortitis with chronic duodenal erosion [58–61]. Diagnosis of endograft infection is based on clinical and radiological findings. Leukocytosis, fever, and back pain are typical clinical signs, while MDCTA may show fluid collection around the rim of the aneurysmal sac. Air bubbles may also be seen within the aneurysm sac [62, 63]. Puncture of the collection or sac for microbiological analysis often gives the definitive diagnosis. After intravenous administration of antibiotics, treatment is always resection of the endograft and aneurysm sac, followed by extra-anatomic bypass or in situ venous bypass.
Contrast-Induced Nephropathy and Acute Kidney Injury
Although patients receiving EVAR are spared the ischemic insult of aortic cross-clamping and have less perioperative hemorrhage [64, 65], the potential nephrotoxicity of large contrast volume must be considered. Correct positioning and deployment of the endograft strictly needs high-quality fluoroscopy and digital subtraction angiography imaging using injection of a contrast agent. In addition, repeated administration of contrast during periprocedural evaluation and follow-up surveillance with MDCTA represents an additional risk for progressive renal dysfunction [66, 67]. Although an average contrast volume of 50–100 mL is commonly needed for an EVAR procedure, higher amounts are not infrequently used as a result of multiple angiographies that may be needed, particularly in complex anatomy for correct endograft positioning and for assessment and treatment of type I endoleak at the proximal or distal fixation sites after endograft implantation. Contrast-induced nephropathy, resulting in acute renal failure, occurs in 6.7 % of cases according to a nationwide survey by Wald et al. [68]. To avoid contrast-induced renal complications, carbon dioxide (CO2) can be used as an alternative contrast agent [69, 70].
In addition to contrast agents, other factors may be responsible for acute kidney injury after EVAR. Manipulation and positioning of the endograft within the aneurysm together with balloon inflation at the proximal fixation site may result in thromboembolism of the renal arteries and renal infarction. Moreover, the type of endograft used for proximal fixation in AAA may have an influence on renal function. Indeed, suprarenal fixation of the endograft is still creating concerns in regards to long-term effects. Interference with renal artery flow, narrowing of the renal ostium, renal infarction, and biological response of the aorta may be the result of continued injury from the suprarenal fixation stent and may play a role in renal function deterioration over time [71, 72].
Post-EVAR Follow-Up
The most common method of evaluating changes in aneurysm sac size is serial MDCTA and measuring the widest diameter for comparison (Fig. 34.14). Ultrasound screening is useful to evaluate sac diameter and to look for evidence of endoleaks, but it may not be sensitive enough to adequately assess early changes. Also, lateral plain X-ray has been used to evaluate graft migration by correlating graft markers with bony landmarks. The most sensitive method for detecting sac changes appears to be three-dimensional volumetric analysis by MDCTA [8]. Serial MDCTA has the benefit of enhanced information compared with ultrasound or plain radiograph. However, the financial burden, cumulative radiation exposure, and potential renal impairment from repeated contrast agent loads are a concern. It has been suggested that if an aneurysm is stable or reduced in size at 12-month MDCTA, it could safely be followed with clinical and ultrasound evaluation. This recommendation is based, in part, on the improved performance of the low-permeability endograft that markedly reduced the rates of endotension and sac expansion at 1-year follow-up. Although this improved short-term performance is encouraging, late expansion is still a concern and the importance of long-term serial evaluation should be emphasized. Another screening approach is to obtain a non-contrast CT image at follow-up. This would provide good information about device migration or deformation, aneurysm sac size, and stent fracture without exposing the patients to contrast agent administration. Screening with magnetic resonance angiography (MRA) is another option and is suitable for the Talent, Excluder, and Quantum low-permeability endografts but not for Zenith or Lifepath endografts due to artifact susceptibility [73].
Thoracic Aortic Aneurysm
Although, in general, cardiac surgeons manage thoracic segments of the aorta and vascular surgeons take care of abdominal aneurysms, the diagnosis and medical care of most aortic pathologies is frequently the responsibility of cardiologists. In light of this fact and considering the emergence of endovascular interventions for this disorder, it is important for cardiologists to gain confidence in managing at least the most frequent presentations of thoracic aortic aneurysms (TAA). Accordingly, it is also important for cardiologists to know the clinical and endovascular management of patients with TAA.
Indications for Intervention
Asymptomatic TAA are initially managed medically. Symptomatic and expanding aneurysms, or those more than 55 mm in diameter in the ascending aorta or more than 60 mm regardless of site or symptoms, are managed surgically. A novel predictor of TAA rupture, the aortic size index, could help predict rupture, dissection, or death. This index uses body surface area information (i.e., mm aortic diameter per m2 body surface area), enabling improved selection for surgical repair on a case-by-case basis and stratification of patients according to risk. A value of 2.75 cm/m2 or less represents low risk of rupture (approximately 4 % per year), 2.75–4.24 cm/m2 represents moderate risk (approximately 8 % per year), and more than 4.25 cm/m2 represents high risk (approximately 20 % per year), further underlining the importance of relative aortic size for predicting complications [74].
Endovascular Repair of Thoracic Aortic Aneurysm
For aneurysmal disease that encompasses the descending thoracic aorta and, in selected cases, the distal aortic arch, the use of endografts for repair of suitable anatomic conditions is emerging as a promising, nonsurgical alternative. Currently, catheter-based endovascular TAA repair (TEVAR) is undergoing clinical investigation [75–81]. As expected, outcomes have improved with growing technical expertise, use of commercially manufactured endografts, and the availability of appropriate patient selection criteria. Overall, technically successful device deployment is currently achieved in 85–100 % of cases. Data from 457 patients treated with endografts (113 emergency and 344 elective cases) and enrolled in the Talent Thoracic Retrospective Registry showed that among the 422 patients who survived the interventional procedure, mortality during follow-up was 8.5 % [80]. Of the 36 patients who died, 11 died from the aortic disease. Persistent endoleaks were reported in 64 cases, of which 44 cases were primary (9.6 %) and 21 occurred during follow-up (4.9 %). Kaplan-Meier overall survival estimates were 90.9 % at 1 year, 85.4 % at 3 years, and 77.5 % at 5 years [80
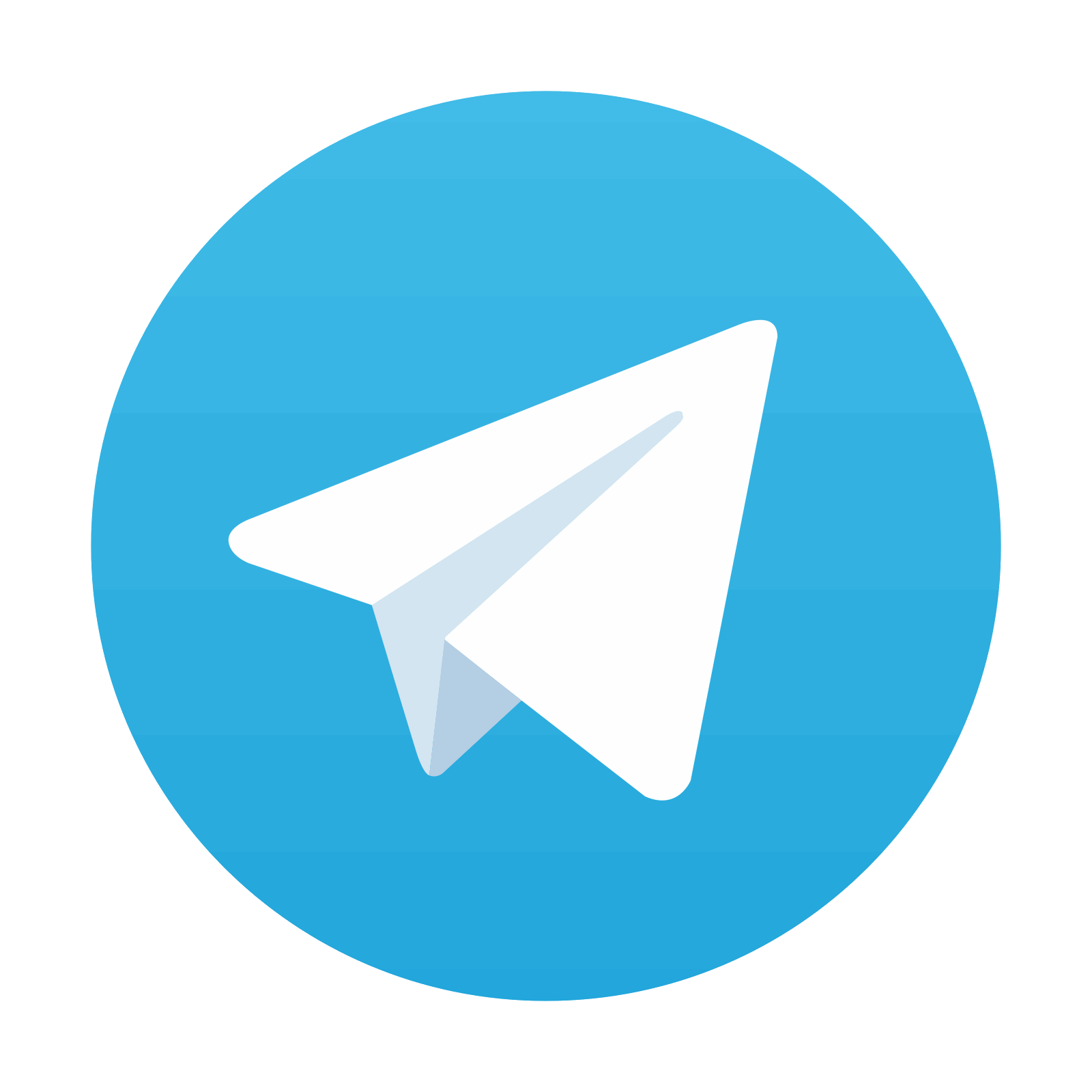
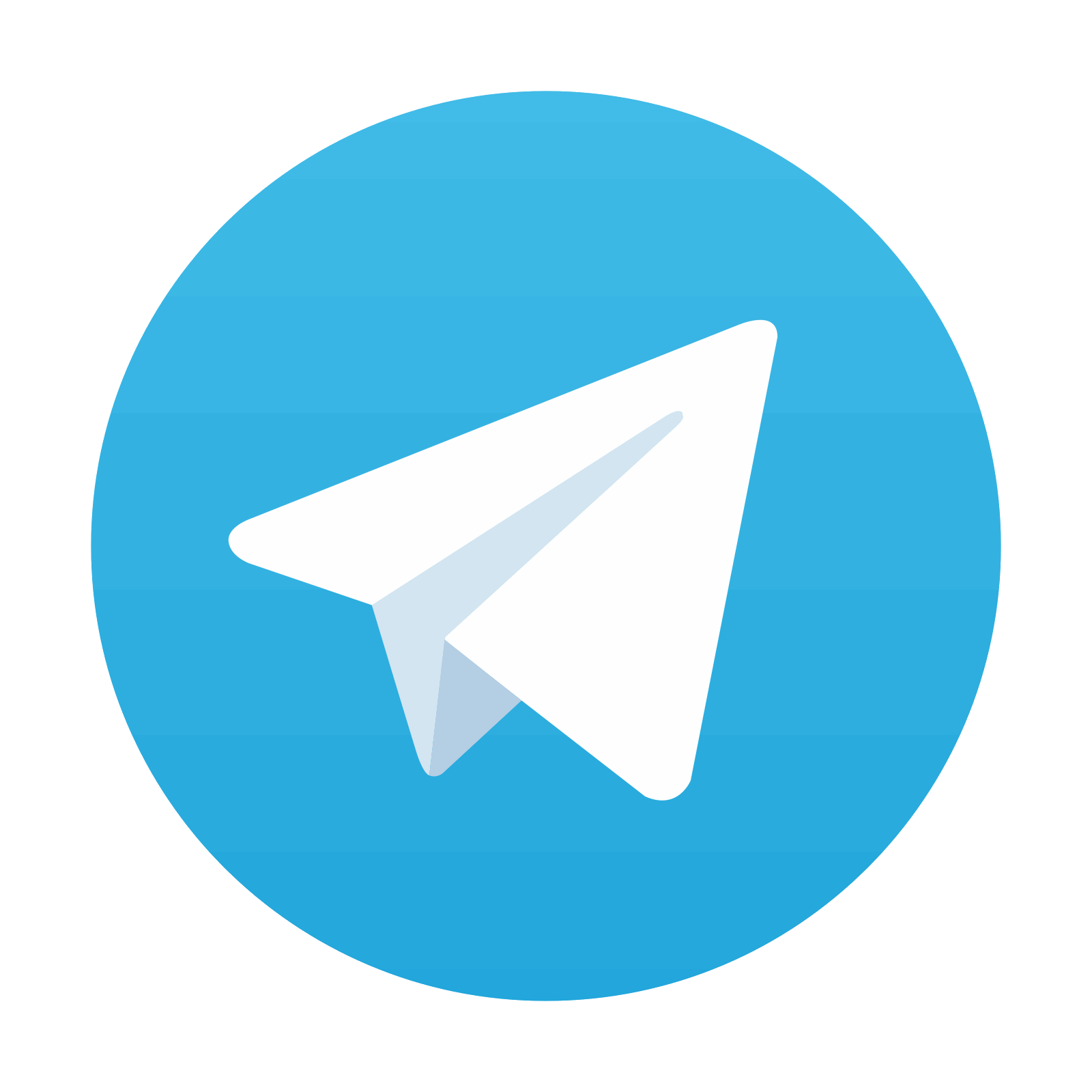
Stay updated, free articles. Join our Telegram channel

Full access? Get Clinical Tree
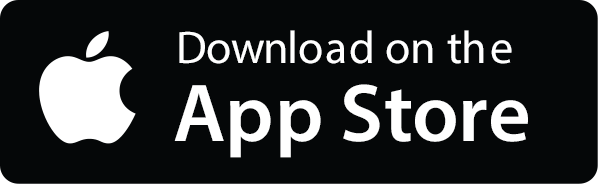
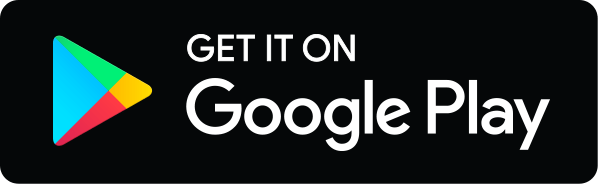