Spinal arteriovenous lesions (SAVLs) are rare disorders, the diagnosis of which can be established using various imaging modalities. To discern the various types of SAVL, spinal angiography of the entire neural axis is required. Surgery is the standard treatment of choice; however, ever advancing endovascular technology can provide a viable alternative. This article discusses the normal anatomy, arterial supply, and venous drainage of the spinal cord. The classification, pathophysiology, epidemiology, clinical presentation, natural history, and pathophysiology of SAVLs in adults are reviewed. Finally, endovascular treatment of these lesions is discussed.
Key points
- •
Spinal arteriovenous lesions (SAVLs) are rare.
- •
Spinal dural arteriovenous fistulas (SDAVFs) are the most common SAVLs.
- •
Diagnosis is difficult, and a high clinical index of suspicion is recommended.
- •
Magnetic resonance imaging should be the noninvasive imaging modality of choice to confirm the diagnosis.
- •
Selective spinal angiography remains the gold standard for the evaluation of SAVLs.
- •
The optimal treatment modality, either endovascular or surgical, remains to be established.
Introduction
Spinal arteriovenous lesions (SAVLs) are rare. SAVLs represent only 3% to 4% of all spinal cord lesions, and can be associated with considerable morbidity and mortality if left untreated. Elsberg was the first person to surgically treat an SAVL in 1914. He successfully treated a spinal dural arteriovenous fistula (SDAVF) with medullary venous drainage. In the early 1960s in the United States, Di Chiro and colleagues pioneered selective spinal arteriography, which led to real-time recognition of the spinal cord vascular anatomy and pathology. At the same time in France, Djindjian was the first to describe the technique of selective spinal angiography. Since this time, there has been an evolution in imaging, endovascular, and surgical techniques. These technological advancements and a better understanding of spinal cord pathophysiology now allow us to manage SAVLs more effectively within the context of a multidisciplinary approach involving neurointerventional radiology and cerebrovascular neurosurgery. However, there is still a lack of a comprehensive understanding of spinal cord pathophysiology, consensus in the clinical classification of the various types of SAVLs, and ambiguity in anatomic spinal cord terminology. Moreover, there are conflicting reports in the literature advocating either an endovascular technique or surgery as the first line of treatment. This article discusses the anatomy, epidemiology, presentation, natural history, pathophysiology, and endovascular treatment of the various types of SAVLs in adults.
Angioarchitecture of normal blood supply of the spinal cord
Arterial Supply
The neural plate starts development during the third gestational week and is derived from the embryologic ectoderm. The process is induced by the underlying notochord, and derives arterial blood supply from individual metameric/mesodermal segmental vessels that originate from the corresponding dorsal and ventral aortic arches at around the third week of gestation. Neural-tube formation commences early in the fourth week (days 22–23), with closure of the cranial and caudal neuropores by days 25 to 27, which coincides with establishment of the intrinsic circulation to the spinal cord. Cellular differentiation and proliferation of the arterial supply to the spinal cord in an embryo closely resembles that of an adult-type pattern by the 10th week of gestation.
In the adult, segmental arteries from the vertebral, deep, and ascending cervical arteries, intercostal and lumbar arteries, median and lateral sacral arteries, and iliolumbar arteries supply the spinal cord, meninges, vertebral bodies, paraspinal muscles, and soft tissues at each level of the spinal column. As the primitive notochord elongates the segmental vessels coalesce in the midline, with the developing anterior spinal artery typically originating from the V4 segment of both vertebral arteries. The individual segmental artery branches supplying the spinal cord regress in their proximal portion in response to the dominant developing longitudinal ventral arterial axis (anterior spinal artery) (ASA) that annexes the distal portion. However, there is usually more than 1 persistent segmental arterial branch that remains patent throughout its length and forms the radiculomedullary artery, which typically follows the course of the radicular nerve root sleeve. The radiculomedullary artery supplements the anterior and posterior spinal arterial supply to the spinal cord ( Fig. 1 A). The dominant radiculomedullary artery in the lower thoracic or lumbar region is called the artery of Adamkiewicz, or arteria radicularis magna. The number and location of the radiculomedullary arteries are unpredictable in a given patient. The course of the radiculomedullary artery has a very typical appearance of a smaller ascending and larger descending branch, with a characteristic “hairpin” loop at the point of entrance to the anterior fissure of the spinal cord. The branchlet from the radicular artery at each segmental level supplying the dura is called the radicular/radiculomeningeal artery, and usually accompanies the corresponding nerve root in its dural sleeve to reach its destination. There are often separate sub-branches supplying the pial surface called the radiculopial arteries.
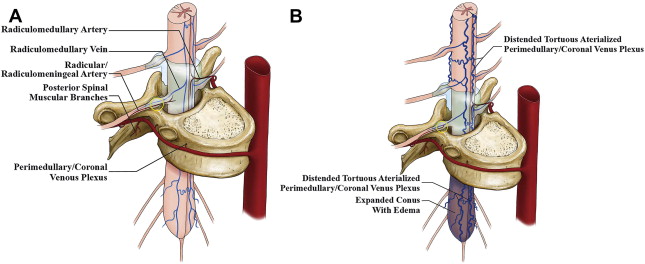
The most common pattern of arterial supply to the posterior spinal cord is via 2 parasagittal arterial axes called the posterior spinal arteries, which are smaller in comparison with their anterior counterparts and form a more discontinuous arcade of vessels. There is free communication between these posterior networks and the sulcocommissural branches from the anterior spinal arterial axis around the lateral aspects of the spinal cord. From these rings (vasa corona) there are penetrating vessels (rami perforantes) supplying the spinal cord parenchyma, with communication from the adjacent level branches.
Venous Drainage
Unlike the brain, the venous drainage of the spinal cord is important in understanding the etiology of the clinical manifestations of SAVLs. Arterial ischemic strokes of the spinal cord are rare. Venous hypertension is the usual cause of congestive myelopathy in SAVLs, and is the final common pathway that causes neuronal ischemia. The venous system of the spinal cord consists of 3 interconnected venous groups: intradural (extramedullary and intramedullary) veins, extradural/epidural veins, and the intraosseus/paravertebral plexi. In addition, there are longitudinal veins, also called the coronal spinal veins, on the ventral and dorsal aspect of the cord. The intradural (coronal) venous plexi are connected to the epidural venous plexi via the radicular/radiculomedullary veins, also termed the bridging veins (see Fig. 1 A), because they do not follow the spinal nerves like the radiculomedullary arteries. There is much more communication between the levels of the venous system than the arterial arcade along the surface and intrinsic/transmedullary pathways of the spinal cord. The transition of a median vein into a radicular vein follows the same characteristic hairpin loop as seen in the radiculomedullary artery. The venous drainage from the spinal cord is centrifugal from the intradural venous plexus into the epidural veins via the radiculomedullary veins, and then ultimately via the azygos and hemiazygos systems into the superior vena cava. The point of dural transgression of the radiculomedullary vein is hypothesized to act as a natural valve that retains the centrifugal venous blood flow of the spinal cord.
Introduction
Spinal arteriovenous lesions (SAVLs) are rare. SAVLs represent only 3% to 4% of all spinal cord lesions, and can be associated with considerable morbidity and mortality if left untreated. Elsberg was the first person to surgically treat an SAVL in 1914. He successfully treated a spinal dural arteriovenous fistula (SDAVF) with medullary venous drainage. In the early 1960s in the United States, Di Chiro and colleagues pioneered selective spinal arteriography, which led to real-time recognition of the spinal cord vascular anatomy and pathology. At the same time in France, Djindjian was the first to describe the technique of selective spinal angiography. Since this time, there has been an evolution in imaging, endovascular, and surgical techniques. These technological advancements and a better understanding of spinal cord pathophysiology now allow us to manage SAVLs more effectively within the context of a multidisciplinary approach involving neurointerventional radiology and cerebrovascular neurosurgery. However, there is still a lack of a comprehensive understanding of spinal cord pathophysiology, consensus in the clinical classification of the various types of SAVLs, and ambiguity in anatomic spinal cord terminology. Moreover, there are conflicting reports in the literature advocating either an endovascular technique or surgery as the first line of treatment. This article discusses the anatomy, epidemiology, presentation, natural history, pathophysiology, and endovascular treatment of the various types of SAVLs in adults.
Angioarchitecture of normal blood supply of the spinal cord
Arterial Supply
The neural plate starts development during the third gestational week and is derived from the embryologic ectoderm. The process is induced by the underlying notochord, and derives arterial blood supply from individual metameric/mesodermal segmental vessels that originate from the corresponding dorsal and ventral aortic arches at around the third week of gestation. Neural-tube formation commences early in the fourth week (days 22–23), with closure of the cranial and caudal neuropores by days 25 to 27, which coincides with establishment of the intrinsic circulation to the spinal cord. Cellular differentiation and proliferation of the arterial supply to the spinal cord in an embryo closely resembles that of an adult-type pattern by the 10th week of gestation.
In the adult, segmental arteries from the vertebral, deep, and ascending cervical arteries, intercostal and lumbar arteries, median and lateral sacral arteries, and iliolumbar arteries supply the spinal cord, meninges, vertebral bodies, paraspinal muscles, and soft tissues at each level of the spinal column. As the primitive notochord elongates the segmental vessels coalesce in the midline, with the developing anterior spinal artery typically originating from the V4 segment of both vertebral arteries. The individual segmental artery branches supplying the spinal cord regress in their proximal portion in response to the dominant developing longitudinal ventral arterial axis (anterior spinal artery) (ASA) that annexes the distal portion. However, there is usually more than 1 persistent segmental arterial branch that remains patent throughout its length and forms the radiculomedullary artery, which typically follows the course of the radicular nerve root sleeve. The radiculomedullary artery supplements the anterior and posterior spinal arterial supply to the spinal cord ( Fig. 1 A). The dominant radiculomedullary artery in the lower thoracic or lumbar region is called the artery of Adamkiewicz, or arteria radicularis magna. The number and location of the radiculomedullary arteries are unpredictable in a given patient. The course of the radiculomedullary artery has a very typical appearance of a smaller ascending and larger descending branch, with a characteristic “hairpin” loop at the point of entrance to the anterior fissure of the spinal cord. The branchlet from the radicular artery at each segmental level supplying the dura is called the radicular/radiculomeningeal artery, and usually accompanies the corresponding nerve root in its dural sleeve to reach its destination. There are often separate sub-branches supplying the pial surface called the radiculopial arteries.
The most common pattern of arterial supply to the posterior spinal cord is via 2 parasagittal arterial axes called the posterior spinal arteries, which are smaller in comparison with their anterior counterparts and form a more discontinuous arcade of vessels. There is free communication between these posterior networks and the sulcocommissural branches from the anterior spinal arterial axis around the lateral aspects of the spinal cord. From these rings (vasa corona) there are penetrating vessels (rami perforantes) supplying the spinal cord parenchyma, with communication from the adjacent level branches.
Venous Drainage
Unlike the brain, the venous drainage of the spinal cord is important in understanding the etiology of the clinical manifestations of SAVLs. Arterial ischemic strokes of the spinal cord are rare. Venous hypertension is the usual cause of congestive myelopathy in SAVLs, and is the final common pathway that causes neuronal ischemia. The venous system of the spinal cord consists of 3 interconnected venous groups: intradural (extramedullary and intramedullary) veins, extradural/epidural veins, and the intraosseus/paravertebral plexi. In addition, there are longitudinal veins, also called the coronal spinal veins, on the ventral and dorsal aspect of the cord. The intradural (coronal) venous plexi are connected to the epidural venous plexi via the radicular/radiculomedullary veins, also termed the bridging veins (see Fig. 1 A), because they do not follow the spinal nerves like the radiculomedullary arteries. There is much more communication between the levels of the venous system than the arterial arcade along the surface and intrinsic/transmedullary pathways of the spinal cord. The transition of a median vein into a radicular vein follows the same characteristic hairpin loop as seen in the radiculomedullary artery. The venous drainage from the spinal cord is centrifugal from the intradural venous plexus into the epidural veins via the radiculomedullary veins, and then ultimately via the azygos and hemiazygos systems into the superior vena cava. The point of dural transgression of the radiculomedullary vein is hypothesized to act as a natural valve that retains the centrifugal venous blood flow of the spinal cord.
Classification
SAVLs are a diverse group of diseases. The traditional classification of SAVLs was purely descriptive and was based on histology, because of a lack of a clear understanding of the pathophysiology of each separate lesion. The evolution of spinal angiography has immensely improved our ability to examine the angioarchitecture of these lesions, which has led to a more accurate classification system based on topographic and anatomic criteria. However, despite these classification refinements an element of subjective judgment persists, because not all lesions conform to the classification system.
The Bicetre group classified SAVLs into 3 main groups. (1) Genetic hereditary lesions are caused by a genetic disorder affecting the vascular germinal cells. The vascular lesions of Hereditary hemorrhagic telangiectasia (HHT) belong to this category. (2) Genetic nonhereditary lesions share metameric links such as Cobb syndrome (or spinal arteriovenous metameric syndrome), which affects the whole myelomere. These patients typically present with multiple shunts of the spinal cord, nerve root, bone, paraspinal muscle, subcutaneous, and skin tissue. Klippel-Trenaunay and Parkes-Weber syndromes are included in this group. (3) Single lesions may reflect incomplete expression of one of the previously mentioned conditions and include spinal cord, nerve root, and filum terminale lesions. The majority of the SAVLs fit this last category.
SAVLs can also be classified into 2 main groups based on hemodynamic criteria: (1) the spinal arteriovenous fistula (AVF) with a direct shunt between the artery and the vein, and (2) an arteriovenous malformation (AVM) with the presence of an intervening nidus of vessels between the artery and the vein ( Box 1 ). Other vascular lesions, such as a capillary telangiectasia and a cavernous hemangioma, do not contain an arteriovenous (AV) shunt and are not amenable to endovascular therapy. The arterial feeders to a spinal dural or pial AV shunt are similar to their cerebral counterparts and are supplied by a radiculomeningeal or a radiculomedullary artery, respectively.
Topographic Classification of Spinal Arteriovenous Lesions
Classification
AVM
- 1.
Intramedullary (also classified as type II glomus-type AVM)
- 2.
Pial
- 3.
Epidural
- 4.
Intramedullary and extramedullary (also known as type III, intradural-extradural, juvenile AVM, or metameric AVM)
- 1.
AVF
- 1.
Pial AVF (also known as type IV, spinal cord AVF, ventral intradural AVF, or perimedullary AVF)
- a.
Small
- b.
Large
- c.
Giant
- a.
- 2.
Dural AVF (also known as type I or a dorsal intradural AVF)
- 3.
Epidural AVF (also known as extradural AVF)
- 1.
In a recent uniform classification of dural AV shunts, both cerebral and spinal AV shunts were categorized into 3 groups based on the embryologic development of the venous drainage of the surrounding structures: the ventral, dorsal, and lateral epidural groups.
The ventral epidural group consists of shunts into those veins that normally drain structures developed from the notochord (ie, the vertebral body). These veins are known as the basivertebral venous plexus, which subsequently drains into the anterior internal vertebral venous plexus, located at the ventral epidural space of the spinal canal, which joins the basilar venous plexus and cavernous sinus cranially. The previously termed “epidural,” “osteodural,” or “paravertebral” AV shunts can be categorized in this group. Because the draining veins of these shunts do not drain the spine but the bone, these shunts will not become symptomatic because of venous congestion of the cord. These shunts can become symptomatic as a result of compression of the spinal cord or nerve roots by enlarged epidural venous pouches. There are a few case reports describing associated perimedullary reflux causing congestive myelopathy.
The dorsal epidural group of AV shunts is related to veins that normally drain the spinous process and lamina at the spinal level. These shunts are related to the major dural venous sinuses (superior sagittal sinus, torcula, and transverse sinuses) at the cranial level; the corresponding veins at the spinal level are poorly developed and consist of a pair of longitudinal channels (ie, the posterior internal venous plexus). Patients with dural AV shunts within this space can present with spontaneous epidural hematomas. These shunts are rare.
The most common, classic types of SDAVFs are the lateral epidural AVFs (see Fig. 1 B). These AV shunts develop in the lateral epidural space at the junction of the bridging (or radicular) veins that connect the spinal cord drainage to the epidural venous system. Outflow obstruction of its adjacent venous outlet, caused either by thrombosis or fibrosis related to aging, will then lead to immediate drainage into the perimedullary veins. As a result, patients in this group present with aggressive clinical symptoms related to perimedullary venous congestion.
Epidemiology, clinical presentation, and natural history in the adult population
Spinal Cord Arteriovenous Malformation
Spinal cord arteriovenous malformations (SCAVMs) represent 20% to 30% of SAVLs. SCAVMs are high-flow lesions supplied by more than 1 branch of the ASA and/or posterior spinal artery (PSA), with a discrete nidus of vessels that drain into the spinal veins ( Fig. 2 ). Associated aneurysms of the feeding arteries and the nidus are common.
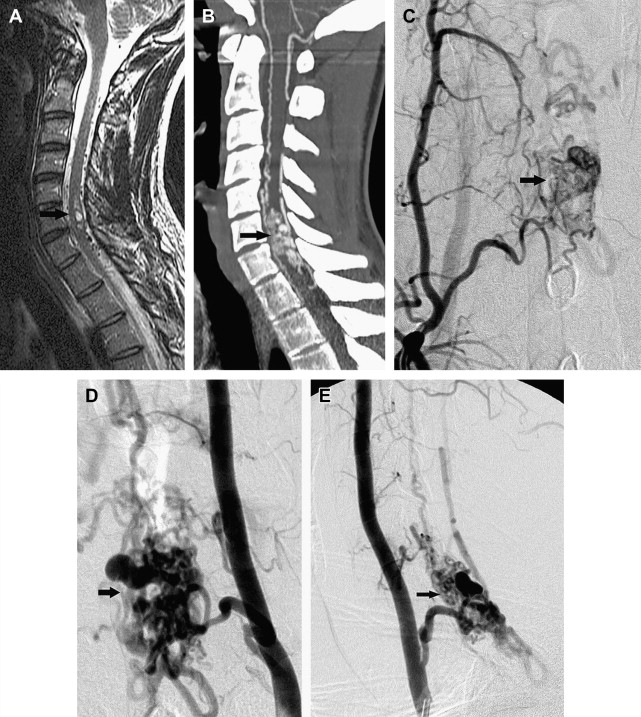
SCAVMs are evenly distributed along the spinal cord axis, and may be located within the parenchyma (intramedullary) (also known as type II or glomus-type AVM), the surface of the spinal cord (pial), or the epidural space (epidural), or they may have a complex anatomy with both intramedullary and extramedullary components (also known as type III, intradural-extradural, juvenile AVM, or metameric AVM). The conus medullaris AVM represents a distinct type located on the conus medullaris or cauda equina, and can extend along the filum terminale.
SCAVMs typically appear in childhood or early adulthood, with sudden onset of symptoms attributable to hemorrhage or compression-induced myelopathy. Patients may present with motor and/or sensory deficits, bladder and bowel disturbances, and pain. Most patients have partial improvement after the initial event, but new events are likely and result in progressive deterioration of spinal cord function. Arterial steal and venous hypertension may occur, and can result in progressive myelopathy. Conus medullaris AVMs frequently produce radiculopathy and myelopathy at the same time.
The prognosis for untreated SCAVMs is poor, with 36% of patients younger than 40 years developing severe impairment 3 years after diagnosis. In a report by Hurth and colleagues 13%, 20%, and 57% of patients had severe clinical deterioration 5, 10, and 20 years after diagnosis, respectively.
Pial Arteriovenous Fistula
Pial AVF is characterized by a single or a few intradural direct AV shunts without an intervening nidus. It is usually located on the pial surface of the cord. Arterial supply originates from 1 or more arterial feeders from the ASA or PSA, and the shunt drains into the spinal cord veins ( Fig. 3 ).
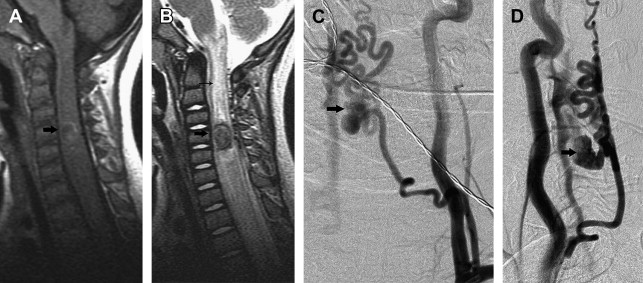
The pial AVFs are subdivided into small (type I), large (type II), and giant (type III), according to the size and flow of the direct shunt. Small AVFs correspond to a single slow-flow shunt between a nondilated ASA and a slightly dilated spinal vein, and are located in the anterior aspect of the conus medullaris or the filum terminale. Small spinal cord AVFs of the conus medullaris can be easily confused with dural arteriovenous fistulas (DAVFs).
Large AVFs correspond to a single or a few shunts, with greater flow than the small AVFs and moderate dilation of the draining vein. Large AVFs are usually located in the posterolateral aspect of the conus medullaris and are supplied by 1 or more mildly dilated arterial feeders from the PSA. Large AVFs can also occur anteriorly, in which case the feeder is a branch of the ASA. Regardless of the location, large AVFs have many arterial feeders, in contrast to 1 or a few shunts.
Giant AVFs have a single or a few high-flow shunts with 1 or more dilated arterial feeders from the ASA and the PSA. The arterial feeders converge to a single shunt, draining into massively dilated arterialized draining veins. Giant AVFs are more common in the conus medullaris region and can be associated with complex vascular malformation syndromes. Giant AVFs can also be seen in the cervical and thoracic levels of the spinal cord.
Small spinal cord AVFs present later in life, with progressive neurologic deficits related to venous hypertension, subarachnoid hemorrhage (SAH) being rare. Hematomyelia has also been observed after rupture of the anterior spinal vein, which is subpial in location.
The large and giant spinal cord AVFs usually present in childhood and adolescence with a variety of clinical scenarios. Acute onset of symptoms can occur secondary to SAH, whereas progressive motor and sensory deterioration and sphincter disturbance usually result from vascular steal, venous hypertension, or mass effect on the spinal cord and/or nerve roots from the dilated veins. SAHs usually occur as a result of venous rupture. The mass effect on the cord or nerve roots from dilated veins explains the occasional asymmetric nature of deficits.
Spinal Dural Arteriovenous Fistula
SDAVFs are the most frequent vascular malformation of the spine, and account for approximately 70% of all SAVLs ( Fig. 4 ). SDAVFs have a male predominance, and diagnosis is most frequent in the sixth decade. The incidence of SDAVF in the younger population is very rare, especially when compared with other SAVLs. Most fistulas are solitary lesions. The most common location of SDAVFs is in the thoracic region, followed by the lumbar region. Cervical location of a fistula is rare; however, some patients can have a fistula in the cervical region below C2 that may present with arm weakness. This presentation is only encountered in a cervical SDAVF, and when present has a localizing value. Sacral lesions occur in approximately 4% of patients.
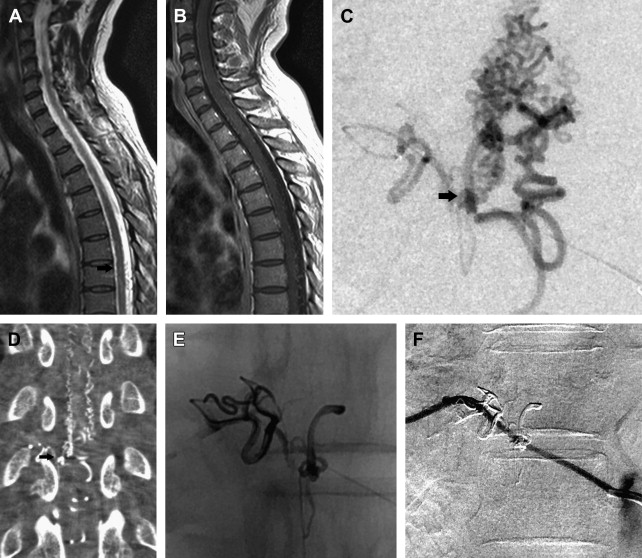
Initial symptoms of venous congestion are nonspecific and include difficulty in climbing stairs, gait disturbances, paresthesias, diffuse or patchy sensory loss, and radicular pain that may affect both of the lower limbs or initially only one limb. Lower back pain without radicular distribution is also frequently encountered. The neurologic symptoms are progressive with time and are often ascending. Bowel and bladder incontinence, erectile dysfunction, and urinary retention are more often seen late in the course of the disease. SDAVFs may mimic more benign conditions such as lumbar spinal canal stenosis, which may lead to a delay in diagnosis or an inappropriate surgery. Leg claudication with exertion tends to be more common in patients with thoracic fistulas.
Spinal cord hemorrhage from an SDAVF is rare, and points toward the presence of a perimedullary (ie, pial) shunt rather than a true SDAVF. Rarely an SDAVF at the level of the foramen magnum with cranial reflux can present with subarachnoid hemorrhage. Two-thirds of the patients at the time of presentation show a combination of gait difficulty, sensory disturbance, fecal or urine incontinence, and sexual dysfunction. In addition, upper and lower motor neuron involvement can coexist in the same patient, as originally observed by Foix and Alajouanine. Hence, physical examination cannot localize the level of the fistula nor does it narrow the differential diagnosis. Confirmation of an SDAVF is based on the findings seen on magnetic resonance (MR) imaging and a spinal angiogram.
Epidural Arteriovenous Fistula
The epidural AVF is a rare lesion associated with significant neurologic morbidity, representing an abnormal shunt between an artery and an epidural vein/venous plexus ( Fig. 5 ). Neurologic symptoms occur by mass effect on the spinal cord and/or nerve roots from the enlarged draining veins, arterial steal, or venous hypertension. Epidural AVFs are primarily described in case reports and small case series, with the cervical spine the most common location.
Pathophysiology
SAVLs may be associated with myelopathy (sensory and motor deficits, bladder and bowel dysfunction), radicular pain or deficit, back pain, or spinal column deformity. Hemorrhage, venous hypertension, arterial steal, and mass effect are the possible mechanisms for spinal cord damage, and their importance varies for each type of lesion. Hemorrhage can occur in the cord parenchyma and/or subarachnoid space, leading to acute onset of neurologic deficits.
The risk of hemorrhage is greater in SCAVMs. Large and giant spinal cord AVFs and cervical DAVFs may also present with hemorrhage, whereas small spinal cord AVFs and thoracic and lumbar DAVFs are less likely to bleed. Spinal artery and intranidal aneurysms are associated with a high risk for hemorrhage. Rarely, SCAVMs with intracranial venous drainage may lead to intracranial hemorrhage. Venous hypertension is typically associated with SAVLs with perimedullary venous drainage.
The classic lesion associated with venous hypertension is the SDAVF, but this phenomenon can be seen with any lesion that has perimedullary venous drainage, such as the pial AVF. The pressure in the perimedullary veins is abnormally increased by the direct AV shunt, and this increased pressure is transmitted to the intrinsic veins of the cord owing to the lack of valves, resulting in “arterialization” of these veins, with thickened and tortuous walls, decreased intramedullary AV pressure gradient, decreased tissue perfusion, and hypoxia of the spinal cord. In addition, loss of autoregulation of the intrinsic cord vessels leads to cord edema and disruption of the blood-cord barrier. Because the conus medullaris is the lowest part of the spine in the upright position, venous hypertension usually predominates there, aided by the valveless venous system. The pressure in the draining veins varies with arterial pressure, resulting in exacerbated symptoms during exercise. Venous hypertension can be confirmed with angiography of the artery of Adamkiewicz by demonstrating severe prolongation of the venous phase. Lesions with high-flow AV shunts may lead to steal of arterial blood from adjacent normal spinal cord tissue. Lesions in the dorsal aspect of the cord that are fed by the ASA are also prone to arterial steal because of the low potential for collateral arterial supply to the normal cord tissue. Mass effect is a rare mechanism for myelopathy. Large aneurysms and large dilated veins/varices like the ones seen with giant spinal cord AVFs may compress the spinal cord or nerve roots.
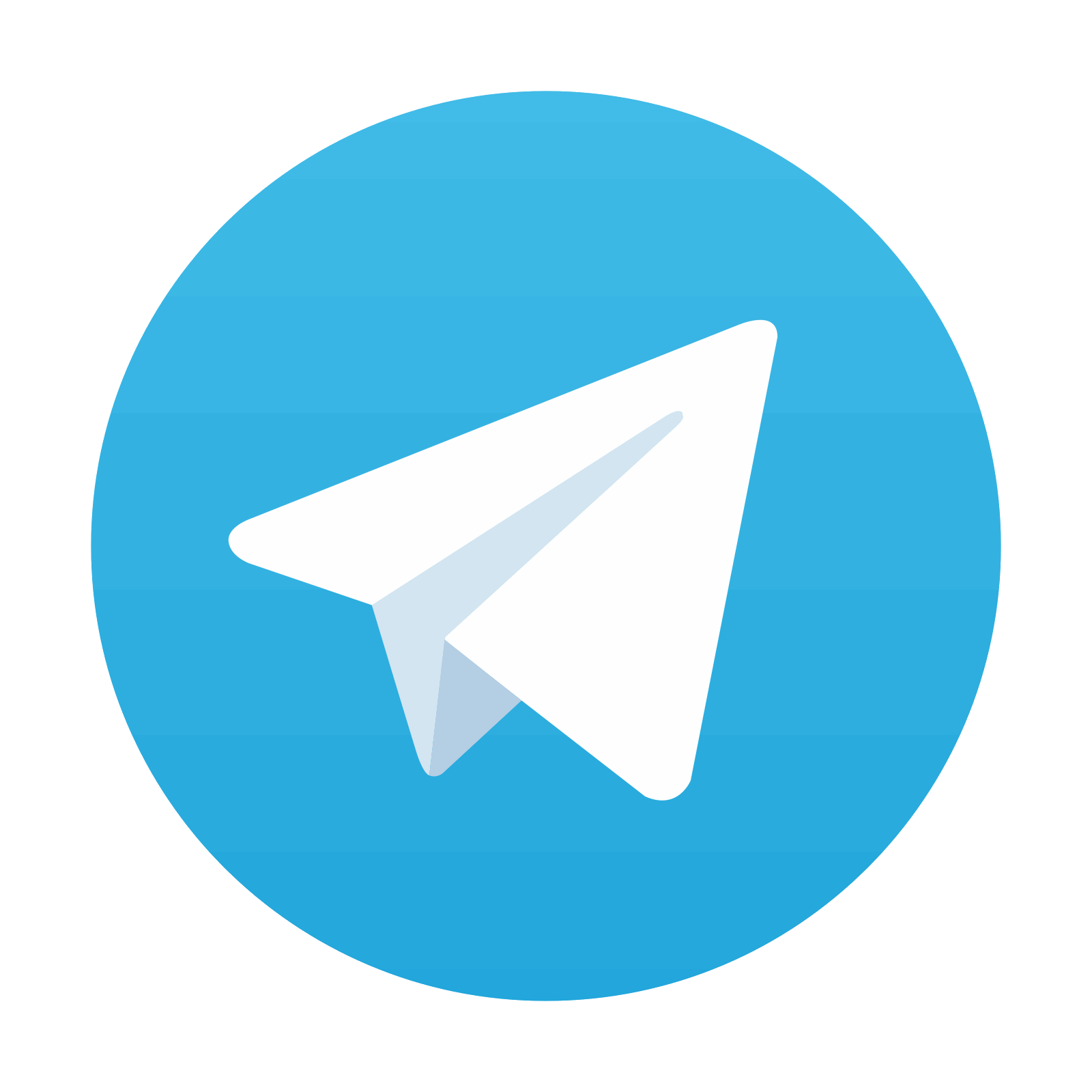
Stay updated, free articles. Join our Telegram channel

Full access? Get Clinical Tree
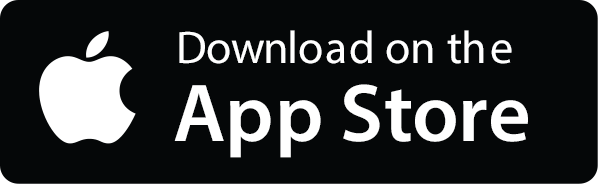
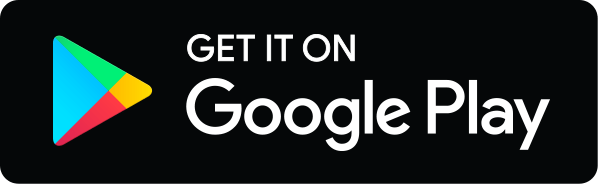