Janos Stumpf, Bagyam Raghavan, Ratna Devi, Mohammed Sathik In the field of oncology, the role of radiotherapist is quite similar to the role of surgical oncologist/surgeon. Both are applying their tools, skill, knowledge and expertise to control cancer locally/locoregionally. In other words, they are targeting both the primary and the regional spread of a solid tumour. The basic principle of surgical oncology in treating a solid tumour is “en block” removal of cancer. Similarly, radiotherapy (RT) targets the primary tumour and its likely spread (through lymphatic) to kill the malignant growth. Radiology itself has bifurcated for diagnostic and therapeutic arms. The past seven decades have witnessed never seen before changes/developments in both. Computers have revolutionalized both specialties. By now, one applies the word/term “image sciences” for the first and “Radiation oncology” for the second. Though that too has changed: one refers to radiology more for the diagnostic and RT for the therapeutic arm. They are “twins” with a never separable path of development. RT treatment planning (TPL) starts at the point of care where diagnostic radiology ends. They opine about the patient’s pathology; based on a test (a digitized – data pool by now). The report goes to the clinicians, and that is the practical end of their task. However, it is special in therapeutic radiology; the digitized data pool itself reaches the computer of the radiotherapist for further procedures. The radiotherapist decides on the dose and type of radiation and starts preparation for RT. The steps are as follows: CT in the treatment position, definition of target and organs of risk on the TPL image pool and computing dose distribution. The plan is approved, and the actual treatment starts. Indication: RT today means more than radiation oncology. Irradiation is being aimed at both malignant and nonmalignant pathologies. Radiation is applied not only for treating malignant pathologies to kill cancer. It is given to arrest the growth of benign cellular tumours, to arrest blood flow in vessel malformations (AVM). Even functional disorders such as trigeminal neuralgia can be successfully controlled by extremely precise radiation. This is why one may say it is not only radiation oncology but RT. Modus operandi: RT acts by delivering certain kind of radiation to the target/pathology in any part of the patients. It has the capacity to destroy cancer and to treat benign conditions. The prescribed dose was limited in the interest of preserving normal tissues. The substantial development, in both diagnostic and therapeutic radiology, provides us the right and the option to go away from the previous “one size fits all” principle in RT dose prescription. RT has undergone enormous change/development during the past few decades especially in targeting deep-seated pathologies. Those could not be assessed with simple clinical examination or tools such as simple X-ray. The past seven decades have witnessed never seen before changes/developments in diagnostic radiology and imaging sciences and RT. Role of modern image sciences is huge. Especially for the precision of the treatment. Without proper understanding/interpreting images, one cannot speak about RT of the “present age”. All these developments are possible because of the development of the image sciences/CT, MR, etc. Computer sciences assure 3D reconstruction of anatomy and pathoanatomy, fusion of different images for precise target delineation, computation of dose distribution in RT. There is substantial difference how a specialist in diagnostic versus therapeutic radiology would understand and interpret images. The job and the interest of a radiologist is to describe the pathology and to give a “qualitative” assessment: “What do I see?” In therapeutic radiology, the size matters, larger the size more the “quantitative” character it has. “How much will I irradiate?” Curative RT goals vary from total control of a malignant tumour, to arrest of the growth of a benign through obliteration of a vessel anomaly (AVM), to arrest of conductivity of a malfunctioning (trigeminal) nerve. All these achievements are supposed to be done with minimal damage to the surrounding normal structures. Intention, however, can be palliative or symptomatic too. Principally in RT, much depends on radiobiology. Sensitivity to radiation: The specialist in RT must know not only the radio sensitivity of the target but also the radio resistance of the surrounding healthy tissues/organs. The success strongly depends on both precise knowledge of the nature of the pathology and its “geographic” environment. Ideally, the radiotherapist should have extensive knowledge in reading the images, and strong understanding about doubts/bias; “When and how the diagnostic radiologist has to get involved in the RT preparation/TPL?” Their expertise helps in proper delineating of the target (first step of radiation TPL) without which no proper result can be expected. Radiobiological studies and clinical experience have supported the main principle in radiation oncology: Cancer cells are likely to die out due to substantial exposure to radiation, whereas the damage caused by the same exposure to the normal surrounding tissue will be reversible/tolerated; the function preserved. Every textbook in radiation oncology has a chapter on organ tolerance. These data, however, are from the past and usually refer to the tolerance of the organ when its total volume is exposed. Precise irradiation has resulted in better dose distribution: homogeneous exposure to the target and steep dose fall-off around it. In consequence of the same, only a part of the healthy neighbouring organ is exposed to a high level of dose. Majority of its volume receives far less. Thus has brought up a new a very practical question “What is the practical radio tolerance of a structure as a function of the percent of its volume exposed?” Impressive example of this phenomenon is the tolerance of the optic nerve. The damaging threshold was understood as less as 8 Gy in a single exposure. (In routine RT, four fractions of 200 cGy make 8Gy) Experience with high precision radiation (HPI), however, has shown that small fragment of the optic nerve can tolerate considerably higher exposure. In consequence, dose escalation, even in close vicinity of the optic nerve, has become a practice (Fig. 1.32.1). HPI gives the freedom/opportunity to prescribe and deliver higher doses with better chance of tumour control in many places/many cases. This dose escalation has brought considerably better treatment outcomes, especially in the tumours when higher dose meant higher local/loco regional control (Fig. 1.32.2). In general, it is known that some cancers are very much sensitive, others are fairly sensitive and there are those which are radioresistant. In the earlier days, RT used to have no chance to control a resistant tumour in a sensitive neighbourhood. Today, one hardly can find a part of the human body, where well-prescribed and applied RT would have no chance. Today RT is being given to benign lesions and functional RT (arrest of trigeminal neuralgia) too is being practiced. The old knowledge was that one can apply RT to a region only once. Today, reirradiation too is everyday’s practice. There is enormous technical development in RT both in external beam (EBRT) and in brachytherapy. Decades ago, radiation used to be directed to the histologically proven pathology based on clinical examination and with the help of some simple tests only. They were helpful but far from precision in delineating the pathology within its normal tissue environment. Needless to say that the RT machines too were not sophisticated. Cobalt units were preferred for quite some time; they were simple and reliable, but accelerators have been dominating the scenario since the last quarter of the 20th century. The working principle of a linear accelerator (LA) is similar to that of an X-ray tube: electron accelerated hits the target, and photons are produced. LAs have become the mostly used photon treatment units. The LA is rotating around the gantry just like the tube does in the CT. If the table moves through the gantry during the treatment, it is called tomotherapy, principally like the spiral CT. Photon energy and the output of the LA can be modulated to achieve a homogeneous dose distribution over the whole target. Thus, it has resulted in intensity-modulated RT (IMRT). With the improvement of delivery precision, the identity of the setup has gained importance. There was a need for diagnostic imaging in the treatment room. Image-guided RT (IGRT) was invented. By now, LAs dominate the RT scenario. However, there is a unit with cobalt sources surviving Leksell’s Gamma knife, developed 70 years ago for stereotactic irradiation in the brain. Today’s precision was absent in both the diagnostic and therapeutic radiation. Special X-rays and special calculation of coordinates were needed. Dose computation in complex arrangements was not yet there. But the unit concentrated gamma radiation to a certain point in the space, and the coordinates of the same were known. It was possible to place the head/the target so that it was irradiated with high dose with considerably less exposure to the normal brain. It was a single exposure originally and that was the start of radiosurgery (RS). Modern imaging, modern TPL systems and modern RT machines have improved the RT precision enormously. Decades ago, RT was given with less sophisticated machines to the whole area where the cancer was likely present but not necessarily seen. Big volumes were exposed. The success of radiation oncology used to be based upon the limited difference between radio sensitivity of the cancer versus the surrounding normal tissue. Development in therapy with particles especially with protons has been there for long. Proton is a charged particle; its acceleration is theoretically relatively simple and technically complex though. Benefit/advantage is based on the absorption pattern with the Bragg’s peak. Without computer technology and image precision, proton treatment could be/was used in special conditions only. Technical progress has resulted in pickup in proton treatment. CT/image sciences have changed the scenario dramatically especially in EBRT. They provide far more precise knowledge about the extension and shape of the disease in comparison with clinical examination or simple X-ray/ultrasound. By now, one can see the pathology even deep in the patient’s body. One can outline it with good precision; therefore the irradiated target has shrunk to the bulk of the pathology; to the target area where high therapeutic dose is inevitable. This was not like that before the advent of modern image sciences. Similarly to EBRT, TPL CT is being used in brachytherapy as well. It is important for the dose distribution around the radioactive sources and how it may cover for the target. Theoretically, TPL CT fused with MR would be the best combination to assure that a malignant soft-tissue tumour is properly covered by the brachytherapy dose distribution. Its use in everyday’s practice is in its nascent phase. After the diagnosis has been established, doctors see into the matter of treatments. Once they concluded that RT was the right treatment of choice, the case is with the radiation oncologist/radiotherapist. The chain of actions starts with prescription of dose, type and time frame of treatment. It is followed by a chain of action. Preparation for RT: special image studies (directly related to the pathology and to the RT) are done in treatment position (Fig. 1.32.3). TPL/dose planning will be done on a special computer called treatment planning system (TPS). Physicist develops the dose plan in collaboration with the radiotherapist. With further development of computer technology, 3D reconstruction of the anatomy and pathoanatomy has become possible. Radiation oncologist could not only see the 3D shape of a cancerous growth, but computerized dose planning and sophisticated/precise dose delivery have improved efficacy of RT. It has become an everyday practice to hit the target with a never-seen-before dose, with a never-seen-before precision. The “spillover” radiation to the normal tissues could be tamed and kept within the level of tolerance. This has resulted in considerably improved tumour killing with preservation of the function of the normal tissues/organs. CT has become the starting test to understand the individual anatomy of the patient and his/her pathology. It is a plain CT in the treatment position, usually with body fixatives to prevent moving during the treatment. Target will be marked, critical organs will be marked, planned/expected radiation doses at different places will be prescribed for computation of the dose plan. Precise delineation of the target is at the centre stage of TPL. TPL CT shows all the organs around so that the dose planning can consider their position saving the neighbouring organs from over exposure. Development in RT is substantial but still the radiation must travel through normal tissues to reach the pathology. Outlining the “vulnerable” organs around the target is a must to keep the level of radiation within their tolerance. Some of them can be outlined well on the CT itself, and some of them appear well on MR only. Advent of MR has improved the precision in visualization of a “soft-tissue” target. Absorption/attenuation of the diagnostic X-ray (in a CT) is very much in the same range for normal and malignant soft tissues. That makes it difficult to see/differentiate between cancer tissue against the normal neighbouring structures. CT with contrast does help a lot if the cancerous soft tissue has rich vascularity. Contrast CT may show cancerous tissue based on contrast enhancement. However, the vascular pattern of a malignant pathology may not be even/homogeneous. Contrast enhancement helps a lot though it has to be considered with caveat in outlining the target. In a solid tumour, the target must cover the less vascular part of the pathology as well. MR is far superior in differentiating of organs and pathologies in a soft-tissue environment. Therefore, MR has a leading role in planning of an intervention in both surgical and RT. There is, however, considerable difference between surgery and RT. A surgeon opens the body, explores the pathology and has additional impression about the extension of the tumour/pathology both visually and “clinically”. Beyond visual appearance, the finger of the surgeon appreciates the texture of the tissue. These are important factors to decide what to cut out and what to leave intact. The radiotherapist does not have this additional information to support his decision what to irradiate and what not. It is commonplace that MR shows far more details of intracranial structures/brain. It has a leading role in showing/outlining an intracranial pathology/target. Signal intensity in MR, however, has not much to do with absorption radiation no matter it is photon, electron, proton or any other type of exposure like neutron. In RT dose planning, its computation is based on CT as a default especially for photon rays; its absorption pattern of a voxel is the basis of the 3D dose computation. The controversy is that MR shows a (soft-tissue pathology) well, but CT may not. It shows the body on the way how the TPL system understands it; based on voxel density, it is essential to compute how the exposing therapeutic radiation would be absorbed. To overcome this difficulty, CT and MR images can be fused. With the help of the same, one can outline the target precisely on the MR and that automatically appears on the CT (Fig. 1.32.4).
1.32: Evolving role of imaging sciences in radiotherapy
Introduction
Radiotherapy in general
Main questions of the treatment
Role of images; cooperation and coordination
Purpose of radiotherapy; outcome (goals and results)
Results, risks and radiobiology
Organ tolerance
Comment: Tumour has invaded the orbit. Lens, retina, optic nerve are all radiosensitive tissues. They start suffering at a fragment of the cancer killing dose if the whole organ is exposed. High precision irradiation (HPI) is focussed very well to the target; small part of the organ volume receives higher dose. The patient developed cataract in the left eye (underwent cataract surgery) and visual field defect, most probably a radiation-induced damage. But vision was preserved!
Dose escalation
Comment: Carcinoma prostate is radioresistant, and the host organ is moving. Rectum, a radiosensitive organ next to it, is just abutting the prostate. Very-high-dose 78Gy and above is needed for a good cure rate, whereas the rectum starts suffering around 50Gy especially if a long segment and the whole circumference is exposed. Tight dose fall-off around the target minimizes the exposure to the posterior wall, thus preventing major radiation injury even with a very high dose to the target. As per, the nature of this tumour treatment result can be assessed after years only. This particular patient had PSA control for a while. PSA started rising again most likely due to active metastasis; MR of the primary has shown no progress.
Precision in radiotherapy
Practice of high precision irradiation
Indication, radiotherapy preparation
Treatment planning images.
Target definition.
Organs at risk.
Targets and other organs
Comment: There was no promising treatment option for this patient with a corpus callosum giant AVM before radiosurgery (RS) has been practiced, TPL CT is plain; the lesion is hardly visible. Contrast CT shows the lesion well especially since water in the ventricles gives good contrast. However, MR is needed to see exactly the other neighbouring structures and clarify doubts about the nidus. An AVM consists of pathological, mainly ectatic vessels. But for RS, the fine network of small vessels (nidus) is important. Principle of RS for an AVM is quite different to the radiobiology based on which a cellular lesion will be hit, be it benign or malignant. The (usually) single-dose RS should cause damage to the endothelium resulting in an overgrowth of the endothelial cells, thus obliterating the lumen of the (pathological) vessels in the AVM nidus. Whereas in benign cellular lesions, the aim of the intervention is to arrest further growth, malignant cells in a tumour must be given a dose enough to kill/necrotize them.
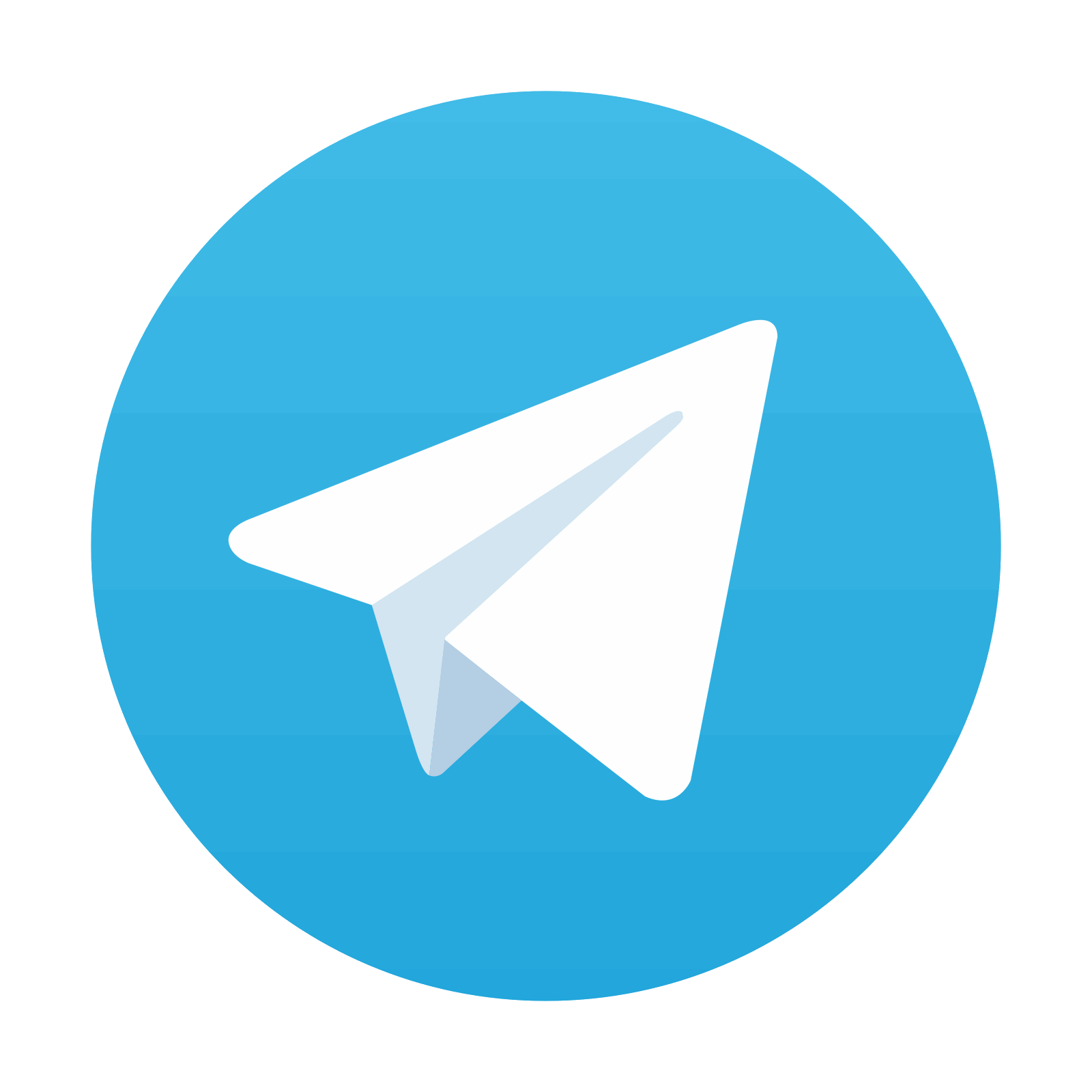
Stay updated, free articles. Join our Telegram channel

Full access? Get Clinical Tree
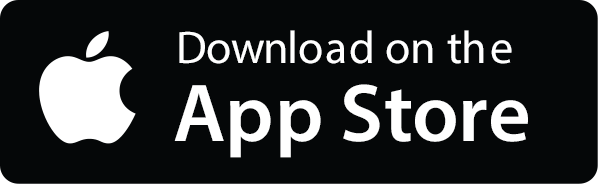
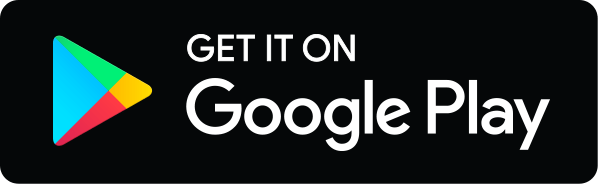