1 Fundamentals
This chapter presents an overview of the most common cerebral findings that are noted in computed tomography (CT) examinations for central nervous system (CNS) trauma and other emergency indications. The contents of the chapter are geared toward the beginner who has no prior experience with cerebral CT.
Clinical Aspects
For many years the brain, enclosed within the cranial vault, was terra incognita for radiologists. The cranial bone encompassing the brain attenuated roentgen rays so strongly that density differences in the brain tissues could not be appreciated on radiographs. The bony skull precludes using a technique similar to mammography, for example, in which tissues with slightly different radiographic densities can be differentiated by imaging them with lowkilovoltage roentgen rays. Standard radiographic films of the brain were only able to detect lesions that differed markedly in attenuation from the cranial bone, such as calcifications (choroid plexus, pituitary) and air.
The introduction of air ventriculography and pneumoencephalography made it possible to detect intracranial masses indirectly by their compressive effect on the air-filled ventricular system. This was followed by cerebral angiography, in which iodinated contrast medium was instilled to opacify the intracranial vessels, and intracranial masses could be detected on the basis of direct or indirect roentgen signs.
Computed tomography, developed by the English physicist Hounsfield in the early 1970 s, used advances in computer technology to register the density differences of brain tissues inside the cranial vault (Fig. 1.1). CT revolutionized neuroradiology by providing a non-invasive means of visualizing soft-tissue structures inside the skull.
Fig. 1.1 Density differences. Tissues with different radiographic densities are assigned different shades of gray on the computed tomography (CT) image. In the example shown, a corpus callosum lipoma situated on the midline has the lowest CT density aside from air (fat has a negative attenuation value and appears black). Calcium at the periphery of the lipoma and the cranial vault have the highest density and appear white. The cerebrospinal fluid (CSF) in the ventricular system has relatively low density. Gray matter has a slightly higher density than white matter. Typical attenuation values in Hounsfield units are:
• Air –1000
• Fat –100
• CSF 0–10
• White matter 30–35
• Gray matter 40–45
• Calcium, bone approx. 1000
The importance of CT in intracranial diagnosis is based on the fact that even a slight increase in intracranial volume can lead to brain herniation and death. On the other hand, invasive diagnostic procedures such as those used in the abdomen (exploratory laparotomy) cannot be safely used for intracranial diagnosis.
Increased intracranial volume can have various causes:
• Intracerebral hemorrhage
• Mass lesions
• Edema
A volume increase that would have no deleterious effects within the chest or abdominal cavity can be devastating inside the cranial cavity.
Increased intracranial volume and pressure tend to produce the following effects on intracranial structures (Fig. 1.2):
• Herniation of the medial temporal lobe (uncus, hippocampus) into the tentorial incisure (Fig. 1.3). This leads to:
– Compression of the ipsilateral oculomotor nerve with mydriasis.
– Compression of the contralateral cerebral peduncle.
– Displacement of the upper brain stem laterally and inferiorly.
– Compression of the upper midbrain against the tentorium, with possible compression of the posterior cerebral artery.
• Herniation of the cingulate gyrus under the free edge of the falx toward the contralateral side.
• Herniation of cerebellar tonsils into the foramen magnum.
Fig. 1.3 Subfalcial and descending transtentorial herniation. Typical CT appearance of subfalcial and descending transtentorial herniation resulting from head trauma. In this postoperative patient, foci of extensive intracerebral hemorrhage are visible in the left frontal and temporal areas, accompanied by intracranial free air. There is a pronounced midline shift, with displacement of the supratentorial ventricular system toward the right side. Right frontal lobe hypodensity may result from anterior cerebral artery compression. Portions of the medial temporal lobe are herniated, obliterating parts of the ambient cistern.
Brain herniation may be caused by a circumscribed mass or by a general rise in intracranial pressure. If the calvarium is intact, the foramen magnum provides the only outlet for relieving the pressure through extracranial tissue displacement. The compartmentalization of the cranial cavity by the hard, relatively rigid falx and by the tentorium tends to prevent the intracranial displacement of brain tissue into other compartments. The high mortality from unrelieved herniation results from direct compression injury of the displaced structures or indirect tissue damage due to ischemia.
Tab.1 Checklist for analyzing cerebral computed tomography scans
• Correct slice thickness?
• Artifacts?
• Scalp normal?
• Visible hematoma?
• Facial soft tissues?
• Discontinuities or displaced fragments in the calvarium?
• Hyperdense subdural or epidural blood bordering the calvarium?
• Sulci visible over all brain areas?
• Effacement of sulci by an underlying mass?
• Individual sulci hyperdense due to subarachnoid hemorrhage?
• Dural sinuses opacified after contrast administration with no filling defects?
• White matter free of circumscribed hyperdensities or hypodensities?
• Ventricular system uncompressed and approximately symmetrical with no midline shift?
• Contrast administration necessary? (for white-matter edema, suspicion of inflammatory brain disease, suspicion of metastases, suspicion of dural sinus thrombosis)
The pathologic changes that are essential in emergency diagnosis involve the white and gray matter of the brain and the intracranial cerebrospinal fluid (CSF) spaces.
Attention should be given to the following changes:
• Density changes.
• Masses.
• Disruptions of the blood-brain barrier, which can be visualized by the intravenous administration of contrast medium.
As in the interpretation of radiographic films of other organ systems (chest, etc.), the beginner should get used to conducting a systematic image analysis in which the anatomic structures are progressively identified and evaluated from the outside in, or from the inside out. This makes it easier to detect abnormalities. An outside-in method of analyzing CT scans is given in Table 1.1.
Fig. 1.4 Beam-hardening artifact. Beam-hardening artifacts from the inner surface of the calvarium are a frequent problem in the CT diagnosis of intracranial hemorrhage. These artifacts make it particularly difficult to evaluate the middle cranial fossa (petrous bone), the posterior cranial fossa, and specific structures such as the internal occipital protuberance. In the case shown, the beam-hardening artifact appears as hypodense and hyperdense streaks in the middle fossa, stretching between the sphenoid wing and the petrous bone on the left side.
Before interpreting a scan, the examiner should perform a brief quality control by asking the following questions:
• Is the calvarium completely imaged?
• Was a smaller slice thickness used for infratentorial scans than for supratentorial scans (e.g., 4 mm vs. 8 mm)?
• Are motion artifacts present? (If beam-hardening artifacts are increased due to motion (Fig. 1.4), they are easily mistaken for hematomas.)
Errors of interpretation can have other causes as well:
• Incorrect positioning of the patient within the scanner (Fig. 1.5).
• Equipment malfunction (Fig. 1.6).
• Defects in the developing machine (Fig. 1.7).
In all trauma patients, the scalp is examined first for any circumscribed expansion that would indicate an extracranial hematoma (Fig. 1.8).
Of course, any patient who has lost consciousness may have fallen and struck the head. The soft tissues of the facial skeleton and the periorbital soft tissues are frequent sites of hematoma formation. These findings should not be overlooked, as they may warrant additional views (e.g., direct coronal CT scans).
Figs. 1.5–1.7 Artifacts caused by faulty positioning. Figure 1.5 illustrates the importance of correctly positioning the patient in the aperture of the CT gantry. The trauma patient was wearing a neck collar that made it difficult to center the head in the aperture. The eccentric position leads to shadowing of occipital brain structures.
Figure 1.6 illustrates a circular ring artifact, which can have various technical causes such as detector malfunction. While most ring artifacts are easily recognized as such, a few can mimic an intracerebral mass. Development errors in Fig. 1.7 mimic round lesions in the frontal white matter and basal ganglia on the right side.
Fig. 1.8 Subgaleal hematoma. An extensive extracranial hematoma has developed over a fracture in the left frontoparietal calvarium.
The cranial bones should be examined for gross displacement due to fractures. The cranial bones cannot be accurately evaluated on CT images acquired with an 8-mm slice thickness and a soft-tissue window. Presumed fractures are difficult to distinguish from cranial sutures, especially for the novice and especially in the occipital region, but sutures are usually distinguished by their symmetrical placement on opposite sides of the skull (Fig. 1.9).
The area adjacent to a skull fracture should always be scrutinized for (epidural) hematoma, just as the area bordering a hematoma should be examined for a fracture. Lytic lesions of the calvarium are frequently missed, especially when solitary (e.g., eosinophilic granuloma), but multiple lytic areas due to metastases (e.g., intracerebral metastases from breast cancer) may also be overlooked.
Proceeding to the cranial contents, the radiologist should look for acute subdural and epidural hematomas, which appear as hyperdense crescents distributed along the inner surface of the calvarium. When narrow, these collections are easily missed on CT scans. This is particularly true if the patient’s head was tilted to one side during the examination, resulting in asymmetry of the CT slices. Concavity (subdural) or convexity of the hematoma (epidural, Figs. 1.10, 1.11) is helpful but is not always a reliable differentiating sign.
One useful distinguishing feature is the fact that epidural hematomas stop at cranial sutures, where the dura is firmly adherent to the inner table of the skull, whereas subdural hematomas may cross the suture lines. If unenhanced CT cannot confirm or exclude a subdural hematoma, scanning should be repeated after intravenous contrast administration. This will increase the density difference between the brain parenchyma and hematoma, because the brain tissue enhances after contrast administration (especially the superficial pial vessels) while the hematoma does not. The most important indirect signs are subtle, because an epidural collection sufficient to cause a midline shift of 2 cm or more should be plainly visible on the scan. The “rabbit ear sign” has been described for frontal subdural hematomas: while the frontal horns of the lateral ventricles normally point forward, the mass effect from a unilateral subdural hematoma can displace the tip of one frontal horn posteriorly, creating a “rabbit ear” pattern. However, some authors refer to this sign in cases of bilateral straightening of the frontal horn, usually secondary to bilateral chronic subdural hematoma.
Fig. 1.10 Differentiation between subdural and epidural hematoma. a Subdural hematoma appears as a hyperdense mass distributed along the inner table of the skull. It typically has a concave medial border and extends across cranial sutures. This hematoma overlies the left frontotemporal convexity and crosses the coronal suture. b Schematic drawing of epidural hematoma that appears as a more localized, biconvex hyperdense mass. The dura itself is not depicted.
Fig. 1.11 Differentiation of subdural and epidural hematoma (see Fig. 1.10). a Left frontotemporal hematoma crossing the coronal suture. b A significant mass effect (midline shift) is often present. c What is your diagnosis based on the information in this newspaper article (from the Sydney Daily Telegraph of 14 July 1998, p. 2)?
Doctor Knows the Right Drill
By Chris Hamilton
A BRITISH doctor has been hailed as a hero after saving a man’s life in the Australian outback by performing brain surgery with a rusty drill found in a school tool shed.
Steve Hindley, 42, was on just the second day of his job as the only doctor in Ravensthorpe, in the south of Western Australia, when Australian Rules footballer Hayden McGlinn was brought in to the tiny hospital. The 23-year-old had collapsed after hitting his head in a collision with another player.
As his condition worsened Dr Hindley realised he had to act quickly to relieve pressure on the brain from a blood clot. With no facilities to perform the intricate neurosurgery he had to improvise with a manual brace-and-bit drill normally used for woodwork.
After a frantic search the player’s team mates and friends managed to find a drill in the school shed and after sterilising it and consulting with neuro-surgeons at Perth’s Sir Charles Gairdner Hospital, Dr Hindley carried out the delicate operation.
Mr McGlinn is now in a stable condition at the Perth hospital where spokeswoman Priscilla Fouracres said he owed his life to the British doctor.
The sulci on the cerebral surface are evaluated not just in themselves but also as a potential indicator of intracerebral masses (Fig. 1.12).
An acute infarction of the middle cerebral artery (MCA), even when complete, may be manifested only by effacement of the cortical sulci in the corresponding territory on one side (Fig. 1.12 b). Analysis of the CT scan should include evaluating the width of all the sulci, and therefore the entire brain surface should be surveyed in all planes. Widening of individual sulci may result from an old infarct or from a small aneurysm that has led to local cortical atrophy due to microhemorrhages. The widths of the extra-axial CSF spaces over different portions of the brain should be compared with one another and with the width of the ventricles. Normal width of the extra-axial CSF spaces accompanied by ventricular enlargement may indicate a rare case of normal-pressure hydrocephalus and distinguish it from other causes of brain atrophy, which may be associated with widening of the sylvian fissures.
Fig. 1.12 Effacement of cortical sulci as evidence of a cerebral mass. Subdural hematomas may be approximately isodense to the adjacent brain, especially when several weeks old. The left superior parietal hematoma in a is isodense, and is manifested chiefly by the effacement of cortical sulci, which are well defined on the right side. The left-sided subdural effusion shows a heterogeneous density, probably due to loculation. a Chronic left subdural hematoma. b Acute infarction of the right middle cerebral artery. c Bilateral brain edema.
A CT hallmark of subarachnoid hemorrhage is hyperdense sulci (Fig. 1.13). These “white” sulci contrast with the majority of the cortical sulci, which are hypodense to the adjacent brain parenchyma and appear dark.
Subarachnoid hemorrhage is most commonly found in the region of the basal cisterns, known as the pentagon. Normally the basal cisterns are hypodense to brain, as they are filled with CSF. Subarachnoid hemorrhage reverses this pattern, and the pentagon becomes hyperdense (Fig. 1.14).
Fig. 1.13 Subarachnoid hemorrhage in the extra-axial cerebrospinal fluid spaces. Individual sulci show a reversal from hypodensity to hyperdensity as a result of the subarachnoid hemorrhage.
Like intraparenchymal hemorrhage, a subarachnoid hemorrhage–especially if caused by a ruptured aneurysm–can rupture into the ventricular system and spread as far as the fourth ventricle. This commonly results in hydrocephalus with pressure cones. The basal cisterns are a frequent site of occurrence of subarachnoid hemorrhage from a bleeding aneurysm in the circle of Willis. The topographic relationships are shown in Fig. 1.15.
Fig. 1.14a, b Density reversal in the pentagon caused by subarachnoid hemorrhage.
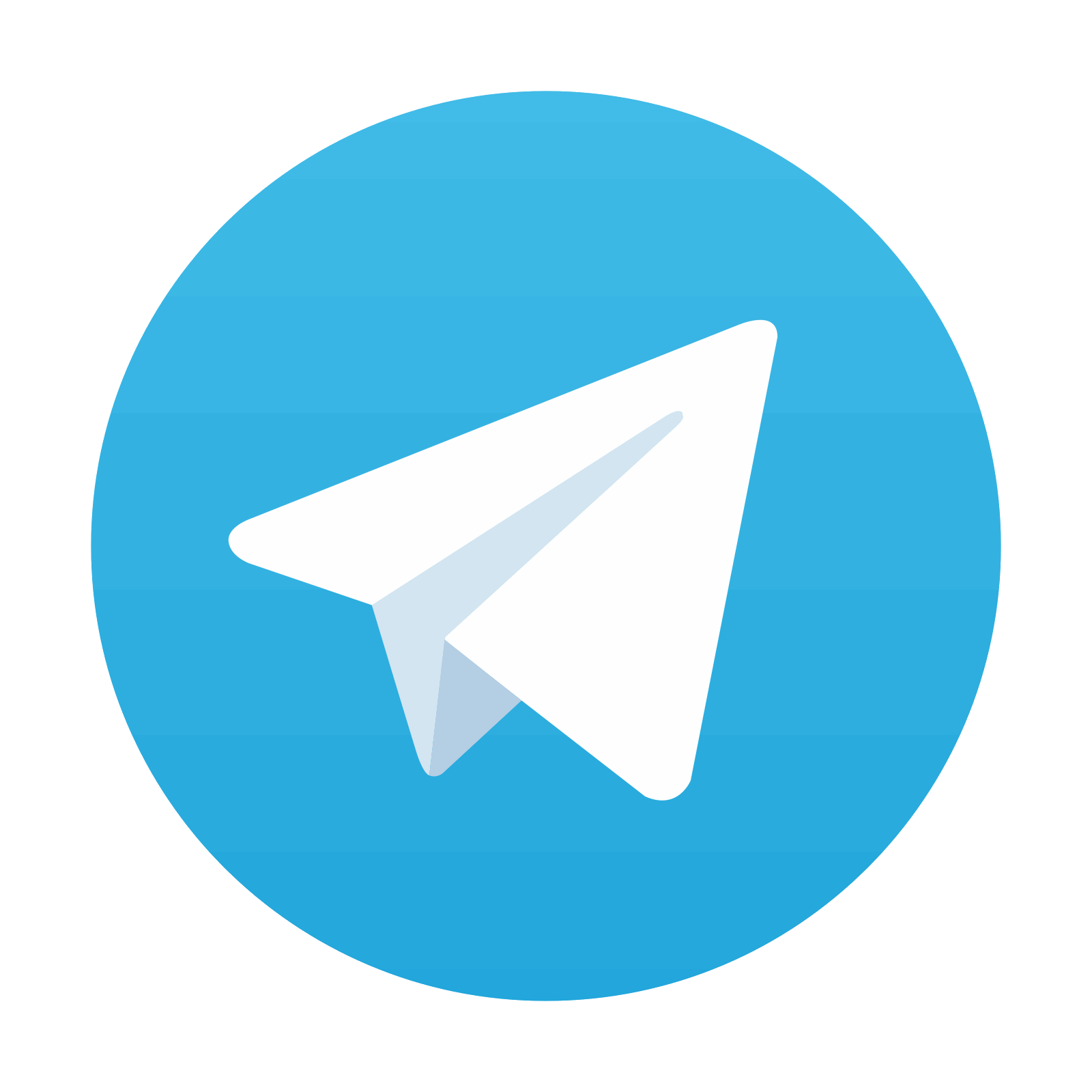
Stay updated, free articles. Join our Telegram channel

Full access? Get Clinical Tree
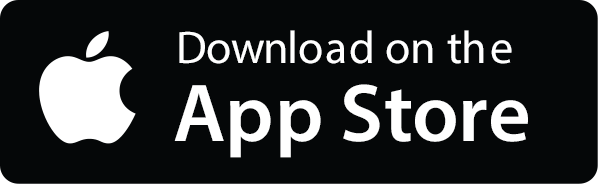
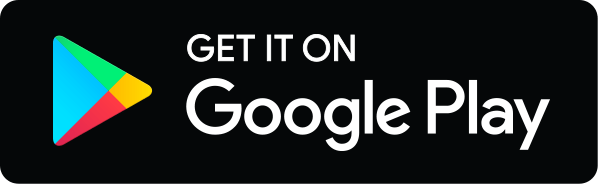