Fig. 12.1
(a) Preoperative CT scan of 1 cm breast cancer. Lumpectomy demonstrated a 1 × 0.7 × 0.5 cm invasive cancer with 7 × 7 × 2 cm lumpectomy specimen with margin of 2 mm. (b) Postoperative CT scan of surgical bed, which was 40 cm3, 100-fold greater than 0.4 cm3 shown in (a)
Four to six weeks after surgical resection, if re-excision is not needed, radiation therapy is administered. In the USA, a standard course of radiation encompasses the whole breast and requires an additional 5–7 weeks, with treatment delivered daily, Monday–Friday. The overall duration of local therapy/BCT (i.e., lumpectomy + radiation therapy) is a minimum of 11 week. As many as 35 % of elderly patients who live a long distance from a treatment facility decide against RT after BC surgery—decisions that contribute directly to decreases in overall survival [8].
Hypofractionated Whole Breast Irradiation and Accelerated Partial Breast Irradiation
In order to reduce treatment costs and increase utility of radiotherapy, hypofractionated whole breast irradiation (HWBI) and accelerated partial breast irradiation (APBI) were developed. HWBI decreases the duration of whole breast radiation therapy to 3–4 weeks. However, patients are still faced with quality-of-life difficulties associated with multiple repeat visits over a long time period. APBI is radically different in that only the surgical bed, rather than the whole breast, is targeted for irradiation. Treating smaller volumes allows safe delivery of higher doses of radiation per treatment, obtaining the same biologic effectiveness in only 1–5 days [9–13]. Early results of several large prospective phase III trials comparing APBI with whole breast irradiation demonstrated similar outcomes, supporting the hypothesis that for select patients [9, 14, 15], whole breast irradiation may not be necessary. In NSABP B-39, the largest phase III trial comparing whole breast radiation and APBI, APBI can be delivered using invasive approaches via various brachytherapy applications or 3D conformal RT (3D-CRT). On this study, more than 70 % of patients randomized to APBI are treated using 3D-CRT as compared with brachytherapy, even though 3D-CRT treats a volume approximately 5× larger than the brachytherapy techniques [16]. This larger volume irradiated leads to a concern that 3D-CRT could result in a higher rate of subcutaneous fibrosis [17] and unacceptable cosmesis [18, 19], as volume is one of the most important predictors of morbidity [20]. Yet many patients choose to receive 3D-CRT instead of brachytherapy because of the distinct advantage of being completely noninvasive.
Role of Technologic Advances in Delivery of RT
Over the last 30 years, advances in immobilization, computer-based treatment planning and delivery, and image guidance have revolutionized the ways in which small tumors are treated with RT. Stereotactic radiosurgery (SRS) was introduced in 1968 as a tool for noninvasive neurosurgery but was not widely adopted until 3D imaging became available. The pinpoint accuracy and high dose concentration achievable with SRS allow safe delivery of “ablative” doses of radiation. SRS has been a success story for patients with brain metastases by improving local control, quality of life, and survival [21]. Its success has hinged on minimizing the volume of normal tissue receiving a “significant” dose of radiation while maximizing dose to the tumor.
SRS outside the brain, called stereotactic body RT (SBRT), has been more challenging [22]. Unlike intracranial targets that do not move in reference to a rigid stereotactic head frame, targets located outside the brain cannot be perfectly immobilized and the organ/tumor motion requires the physician to add a margin to ensure appropriate dose coverage of the target volume. The planning target volume (PTV) is defined by an additional margin to account for tumor motion and setup uncertainties. This added margin increases the volume of tissue irradiated, which theoretically increases the risk of side effects [23]. Over the last 15 years, solutions have allowed SBRT to treat tumors outside the brain [24, 25]. In fact, in lung cancer, the results [26, 27] have been so impressive that investigators are currently challenging the paradigm of surgery as the standard of care for patients with small lung cancers [28].
SBRT of breast cancer has been addressed in only two published reports [29, 30]. These studies have severe limitations because of poor immobilization and lack of adequate image guidance, allowing only subtherapeutic doses of radiation and possible marginal misses because of set up uncertainty. One of the major challenges of SBRT for breast cancer is that the breast is pliable. These challenges have been solved in the brain, thorax, and abdomen with commercially available immobilization devices. In addition in the breast, surrounding structures have placed limitations on beam delivery. These technical obstacles have been solved through an NIH-SBIR grant mechanism (4R44CA132254-02) at the University of Maryland by Yu et al. [31] with the inception of GammaPod™ technology, commercialized by the Xcision Medical System, LLC (Xcision). A detailed description of the GammaPod™ device and underlying technology will be provided in subsequent sections.
The GammaPod™ device has several advantages over current technologies capable of SBRT, including but not limited to (1) the vacuum assisted breast immobilization device has improved accuracy and reproducibility. In addition, the patient is treated in the prone position, similar to positioning for stereotactic breast biopsy or MR imaging of the breast, thus minimizing the effect of breathing motion. Subsequently, the margin required for PTV can be decreased from 1.0 to 0.3 cm, potentially reducing the volume radiated by threefold [32]. (2) This device uses 36 noncoplanar Co-60 sources that rotate during treatment to form 36 noncoplanar arcs, producing a dose distribution similar to that of the GammaKnife™ for intracranial targets [33]. (3) This system allows differential dosing of target volumes [34] (dose painting; similar to that in intensity modulated RT techniques), which cannot be accomplished with any invasive APBI approaches. This allows higher doses to the gross tumor with lower “subclinical doses” to ablate microscopic foci, which can lie 1.0–1.5 cm beyond the index lesion [35, 36].
GammaPod™ offers the opportunity to deliver curative doses of radiation using SBRT more precisely than current surgical standards, thereby minimizing the amount of breast tissue ablated and improving cosmesis. If this treatment is as effective as it has proven for lung cancer, SBRT could lead to a paradigm shift similar to that seen in lung cancer, with completely noninvasive treatment of early breast cancer with only a few high dose “ablative” treatments delivered over the course of less than 1 week.
Role of Preoperative RT in Early Stage Breast Cancer
In preparation for developing a clinical trial using SBRT with ablative doses of RT, we investigated the role of preoperative 3D-CRT APBI as a method to reduce morbidity and allow greater utility of APBI. We have demonstrated that using a preoperative target volume dramatically decreases the PTV by a mean average value of 56 % when compared with the standard postoperative target [32]. In a subsequent publication, we reported that preoperative 3D-CRT APBI showed significant clinical advantages by decreasing dose to all surrounding normal tissues and substantially increasing (by 60 %) the number of patients eligible for APBI using 3D-CRT [37]. Based on these dosimetric advantages, we are currently testing this hypothesis on a prospective trial at the University of Maryland. This trial uses the same treatment guidelines (i.e., clinical treatment volume and PTV expansions) and dose fractionation (38.5 Gy delivered in ten equal fractions over 5 days) as NSABP B-39, with the only difference being the sequence of surgery and RT. Based on early data from the first 25 patients treated on study, a complete pathologic response was achieved.
These pathologic findings have been quite exciting for two reasons. First, only a low “adjuvant” dose of radiation was administered, and, second and more important, a response was actually seen. These tumors are slow growing and can take a long time to respond. Prostate cancer, which has similar growth patterns, takes years to show histologic resolution of tumor following radiotherapy. Therefore, we believe that small, early stage breast cancer, SBRT may replace lumpectomy and postoperative RT. With the less aggressive, slower growing tumors in the breast, it is likely that SBRT will approach or surpass the efficacy of 90 %+ local control rates seen in SBRT for lung cancer. Although clearly many studies will be necessary to prove this hypothesis, in order for SBRT to be realized as a standalone treatment, a noninvasive measure of success is key which will require functional imaging such as MRI or PET. If successful and implemented clinically in the future, select patients will receive SBRT alone. The geometric accuracy will likely improve cosmesis by causing minimal-to-no volume loss, eliminate surgical scarring, improve quality of life issues currently associated with prolonged treatment periods, and enhance overall outcomes.
GammaPod™ Technology
The GammaPod™ device comprises three major components: the gamma irradiation unit housing the Cobalt-60 radioactive sources and collimation structure, a breast immobilization unit to facilitate stereotactic localization and prone patient support and a continuous trajectory patient positioning assembly.
Irradiation Device
The GammaPod™ irradiation unit comprises of two mechanical structures: a source carrier and a collimation unit as shown in Fig. 12.2.
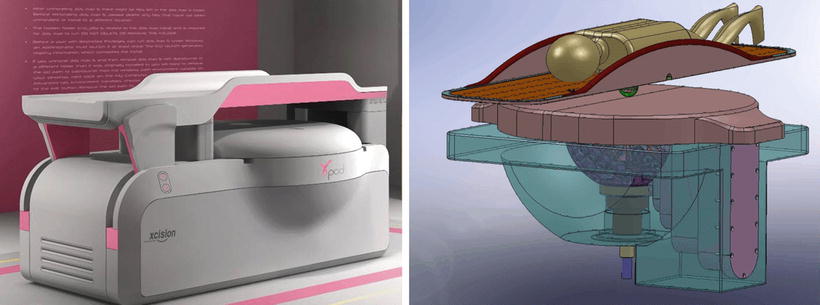
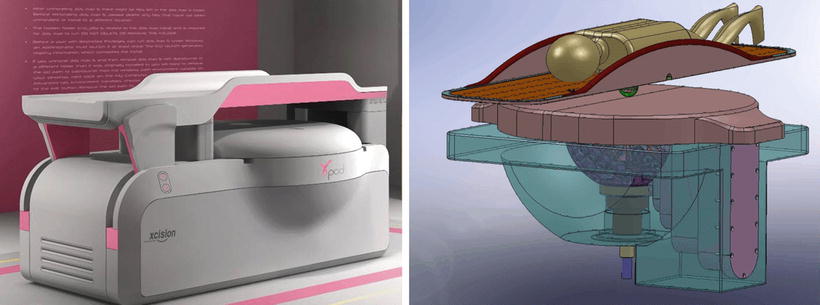
Fig. 12.2
An artist rendering (left) and 3D model drawing (right) of GammaPod™ depicting prone placement of a patient. (Courtesy of Xcision Medical Systems.)
The hemispherical housing structure contains the radionuclide source in the form of 36 Cobalt-60 pellets. These pellets are positioned equidistantly, 38 cm from the isocenter (source to focal distance, SFD). With an SFD of 38 cm, achieving an initial dose rate greater than 5 Gy/min inside a large breast requires an estimated total Cobalt-60 activity in the range of 4,000–5,000 Curies. The Cobalt-60 source is double-encapsulated with the inner capsule packed tightly with smaller Cobalt-60 pellets. The source shell dimension is customized to hold the required number of radioactive pellets. The cylindrically shaped inner capsule of the source shell measures approximately 11 mm in height and 3.4 mm in diameter, reflecting the true radiation source size of a single source.
Sources are aligned in six columns across the 2π surface of the source carrier, with 60° longitudinal separation. Each source column is arranged in a spiral order, such that a source is positioned at every 10° longitudinally. The latitude of each column is also designed such that all 36 sources span an angular section between 18 and 53°, i.e., with 1° of latitudinal separation. This arrangement ensures that each of the 36 sources occupies a unique longitude and latitude coordinate when the source carrier is rotated.
Sources are collimated by the primary collimator, located at the exiting end of the source shell. The secondary collimator, with the same hemispherical shape, is nestled within the primary collimator. Collimation is performed by pre-drilled circular holes aligned with the sources. By altering the alignment of the source carrier and collimator, three different collimation modes can be chosen (two open, one blocked). The GammaPod™ is designed to have two collimator opening sizes, 1.5 and 2.5 cm, in order to increase the dose shaping capabilities of the device. In contrast to radiosurgery of intracranial lesions, in breast cancer larger collimator sizes are selected due to the typical tumor sizes and the need to sterilize the tissues surrounding the tumor owing to its multicentricity nature.
When the selected collimator opening is aligned with the source positions, the source carrier and the collimator are rotated together to create 36 noncoplanar conical arcs all converging at the isocenter. In addition, the 36 source blocking positions on the primary collimator are made of tungsten rods, effectively reducing the radiation to acceptable leakage levels at the beginning and end phases of the fraction.
The GammaPod™ irradiation unit is encased in a hemispherical shielding body made of cast iron. There are two shielding doors on top of the irradiation unit which opens during the irradiation and stays closed at other times. The shielding body and doors are designed to bring leakage radiation levels below those set by the regulatory bodies when the device is not in use. The irradiation unit and shielding doors are equipped with an uninterrupted power supply (UPS) unit to ensure that treatments can be terminated safely in the event of facility power failures. The source carrier and the shielding doors can also be controlled manually using a hand crank should the other safety measures fail.
Breast Immobilization Apparatus
The GammaPod™ system utilizes a two-layered breast cup in order to immobilize the breast (Fig. 12.3). Several inner cup sizes accommodate patients with varying breast sizes. The outer layer is composed of a solid plastic material and connected to a vacuum system working at a comfortable level of negative air pressure. While the patient is in the prone position, the treated breast is positioned inside the inner cup of the breast cup. The inner cup is perforated so that when a vacuum is applied to the space in between the outer and inner cups, the breast volume fills the entire contour of the inner cup and the coordinate frame is transferred to the patient’s anatomy. The inner and outer cups are tightly coupled with a silicone flange, which is attached to the patient’s chest wall with a thin layer of silicone restricting breast mobility with respect to the inner cup. In preliminary studies [38], the GammaPod™ immobilization system was shown to reduce the setup uncertainty to 0.2 ± 0.1 (2σ) cm, and therefore the amount of margin necessary for PTV expansion may conservatively be set at 0.3 cm for radiosurgery applications.
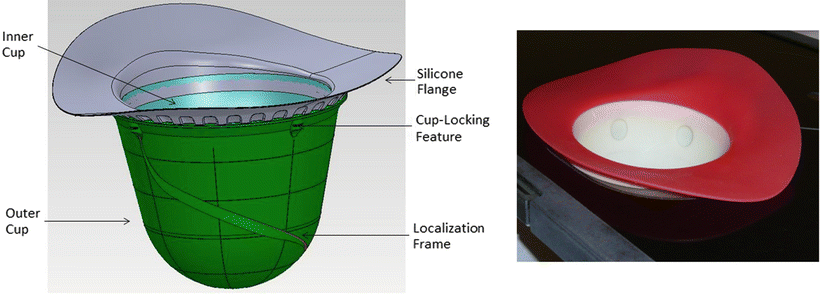
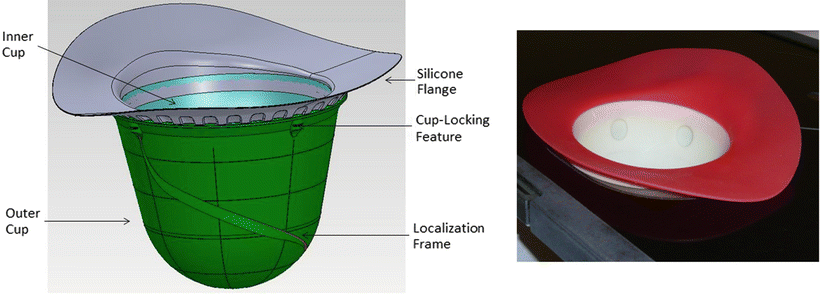
Fig. 12.3
A schematic drawing for the two-layered breast immobilization cup is shown on left panel with embedded stereotactic frame imaging fiducial wire. A photo of the prototype breast cup is also shown on right. (Courtesy of Xcision Medical Systems.)
The breast cup immobilization system also has the benefit of gently pulling of the breast volume away from the patient’s chest wall, therefore providing larger separation between the targeted area and the ribs, lung and the heart in the case of left breast treatments. The increased separation is an added advantage to the similar geometric advantages caused by the prone positioning of the patient’s breast especially for pendulous breasts. To further increase comfort and immobilization, a wide strap is affixed to the base of the cup, which wraps around the chest of the patient.
The outer cup also incorporates a stereotactic localization frame with the help of a spirally shaped CT and MR-compatible fiducial as shown in Fig. 12.3. The frame consists of a helical hollow plastic tube wrapped diagonally around the outer cup. The plastic tube is filled with either MRI contrast solution or copper wires, and is solidly secured to the machined groove on the outer cup. Once the fiducials have been identified a shared coordinate frame system between the immobilization device and the patient’s anatomy is created.
Continuous Trajectory Patient Positioning System
The couch and the patient positioning system are critical components of GammaPod™ stereotactic radiotherapy device. The couch top has built-in pegs that are machined to fit perfectly with the breast immobilization device, therefore creating a rigid registration system. Using this registration protocol, the shared coordinates between the immobilization device and the patient anatomy become a global coordinate frame between the treatment unit and the patient’s anatomy.
Two couch systems exist for GammaPod™ treatments: The imaging couch top and the treatment couch. In both systems, the couch-tops are brought to an upright position using a pivoted motor assembly that enables patient docking. Both couch-tops consist of two holes to accommodate the treated left or right breast while the contralateral hole is covered with a lid. Once docking is accomplished, the patient is lowered to the treatment or the imaging unit (CT or MR device) via a cantilever action of the couch actuators. This automatic patient positioning mechanism reduces the amount of motion and effort required by the patient, which could otherwise compromise breast immobilization.
The treatment couch unit is also equipped with three-dimensional motor controls which move the patient in relation to the radiation isocenter of the GammaPod™. This 3D motion trajectory also enables continuous shaping of the dose distribution across the target volume. Therefore, instead of packing multiple spherical and static dose distributions, generally referred to as shots, which result in large dose heterogeneities, the GammaPod™ attains a more uniform dose distribution. This dose shaping allows for the treatment of different target volumes at different dose levels (such as primary gross tumor volume (GTV) versus the surrounding rind of subclinical disease extent receiving higher and lower dose levels, respectively).
The GammaPod™ treatment couch is also equipped with redundant safety features to prevent any collision of the device components with the patient. One of the safety features employs metal ports mounted on the shielding door which prevent the lowering of the treatment couch when the shields are closed. The treatment couch also acts as a physical block, preventing the shielding doors from closing while the patient is lowered into the treatment position. Knowing the outer breast cup dimensions also allows for the imposition of trajectory limits for treatment plan optimization and thus prevents inadvertent collisions with the inner surface of the hemispherical collimator structure.
GammaPod™ Treatment Planning System
The GammaPod™ stereotactic device is distributed with a dedicated treatment planning system, called Ceres. In its current implementation, Ceres provides capabilities for multimodality image import, stereotactic registration and localization, manual contouring and automatic detection of the breast/body surfaces, inverse optimization, dose calculation and evaluation, treatment export and various quality assurance (QA) utilities.
After the breast CT/MRI images are imported, breast cup fiducials are identified in a single cross-sectional image, which initiates automatic detection of the entire spiral. Refinements to the detected fiducial geometry are performed to match the known helical geometry of the stereotactic frame. After the registration of the stereotactic coordinate frame of reference to the patient image set, the software detects the actual breast contour within the breast cup and creates the whole breast volume. At this point other organs of interest, such as chest wall and ribs, lungs, and/or heart volume, can be manually drawn. The target volume creation starts with the delineation of the primary GTV and followed by the generation of other concentric targets using margin expansions from the GTV, e.g., CTV. During the margin expansion process, the automatically generated contours are restricted from expanding beyond the 0.5 cm distance to the breast skin surface.
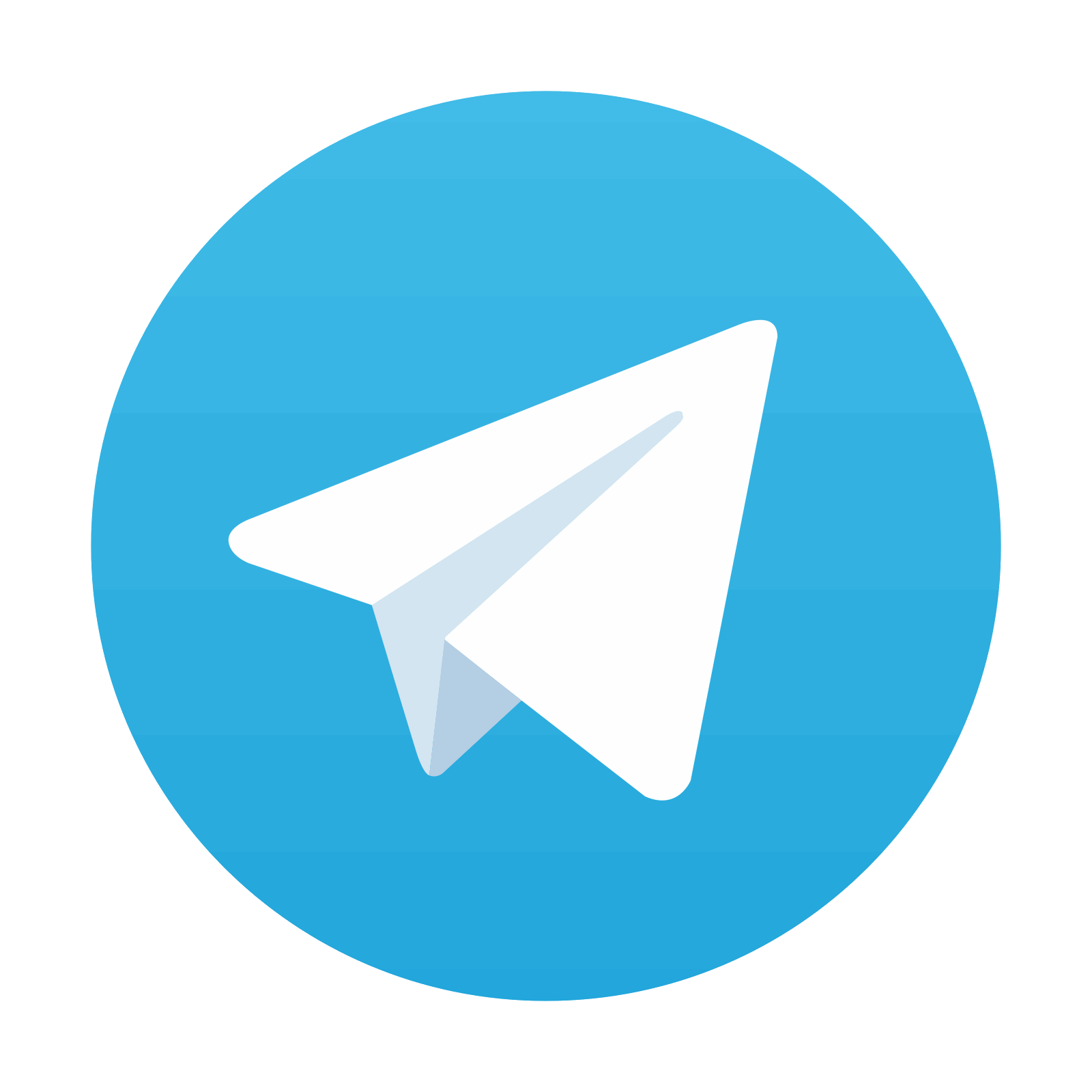
Stay updated, free articles. Join our Telegram channel

Full access? Get Clinical Tree
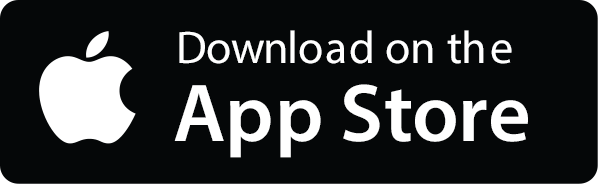
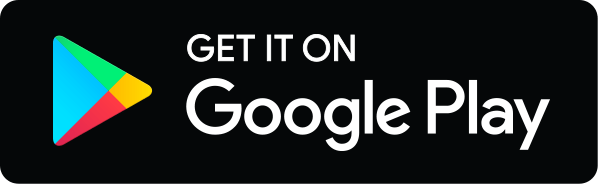