Abbreviations
ADC
apparent diffusion coefficient
AUC
area under the curve
CAPIRINHA
Controlled aliasing in parallel imaging results in higher acceleration
CD
Crohn disease
CE
contrast-enhanced
CRC
colorectal cancer
CT
computed tomography
DWI
diffusion-weighted imaging
EUS
endoscopic ultrasound
FDG
[18F]fluorodeoxyglucose
FSE
fast spin echo
GI
gastrointestinal
HASTE
Half-Fourier acquisition single-shot turbo spin echo
IBD
inflammatory bowel disease
M
metastasis
MRI
magnetic resonance imaging
N
node
PET
positron emission tomography
SCC
squamous cell carcinoma
SI
signal intensity
SMS DWI
simultaneous multislice diffusion weighted imaging
SUV
standardized uptake value
SUVmax
maximum standardized uptake value
T
tumor
UC
ulcerative colitis
VIBE
Volumetric interpolated breath-hold examination
Introduction
The unmatched sensitivity of positron emission tomography (PET) combined with the high soft tissue contrast resolution of magnetic resonance imaging (MRI) makes PET/MRI suitable for the investigation of both gastrointestinal (GI) malignancies and inflammatory bowel disease (IBD) ( ; ; ). Furthermore, this modality presents a reduced radiation dose when compared to similar imaging methods, with estimated mean effective dose about 20%–60% less than PET/computed tomography (CT) with low-dose CT or diagnostic-quality CT ( ; ; ). This is especially important for patients with IBD, who may undergo several scans over their lifetime ( ).
GI cancers are a significant health problem worldwide. In 2020, they represented 27% of all new cancers and 37% of cancer-related deaths ( ). Interventions to prevent, timely diagnose, and accurately stage these cancers are essential to diminish the large burden they cause. Although imaging plays a relatively limited role in prevention, it is critical for prompt diagnosis and proper staging of GI cancers. Imaging that is both sensitive and specific is essential to define the appropriate treatment for these patients. PET/MRI might improve the staging and change the clinical management in patients with several neoplasms, including abdominal malignancies ( ; ; ; , ; ; ; ). PET/MRI has also been reported to better demonstrate lymphadenopathy in abdominal cancers when compared to PET/CT. Additional evidence supports improved detection of bony and liver metastases when compared to PET/CT ( , ). Initial data from our group also highlights superior performance than currently used imaging modalities in evaluating peritoneal metastases ( ). As better detailed in the chest chapter, one of the main weaknesses of PET/MRI for oncologic imaging is related to the detection of lung lesions where PET/MRI usually underperforms when compared to PET/CT ( ). Despite this weakness, PET/MRI could still serve as a pivotal whole-body staging method since, in most instances, the missed nodules will not warrant management changes. For example, in colorectal cancer, there is no added survival benefit of lung metastasectomy ( ). Furthermore, most of the lung nodules are encountered in patients suffering anyways from advanced disease (stages III–IV), in whom detection of additional metastases in the lungs might not prompt management changes ( ).
IBD encompasses a group of chronic inflammatory conditions that includes Crohn’s disease (CD) and ulcerative colitis (UC). Those pathologies are associated with considerable morbidity and affect as many as 3 out of 1000 people in North America ( ). In IBD, imaging is used to demonstrate disease activity and investigate possible complications such as abscesses, fistulae, and stenoses ( ). On MRI, diffusion-weighted imaging (DWI), and dynamic postcontrast sequences are effective in detecting bowel wall inflammation ( ; ; ). On PET, the rate of [ 18 F]fluorodeoxyglucose (FDG) uptake, as quantified by maximum standardized uptake values (SUV max ), is also correlated with the degree of inflammation. However, FDG is a nonspecific radiotracer and is therefore unable to differentiate among infection, inflammation, and cancer. Additionally, FDG-PET can result in false positives due to physiologic uptake by normal bowel ( ; ). The combination of PET and MRI data enables better detection of active inflammation, fibrosis, and extraluminal disease in CD compared to PET/CT, which may lead to management changes ( ). Hence, PET/MRI may be a noninvasive answer to the current dilemma of how best to monitor and evaluate treatment response in patients with IBD.
Therefore, the case for PET/MRI’s use in the aforementioned pathologies seems promising. Over the following subsections, we offer an in-depth analysis of PET/MRI’s advantages and pitfalls.
Acquisition protocols
Several acquisition protocols have been reported in the literature. We report a summary of the protocols currently used at our Institution.
Our PET/MRI protocol always simultaneously acquires PET and MRI data, with the length of the PET acquisition influenced by the MRI acquisition time. FDG incubation time is 60 minutes; scan always progress from the midthighs upwards.
GI malignancies
In the case of rectal cancer, we acquire:
- 1.
Pelvic rectal protocol: high-resolution axial T1-weighted Dixon CAPIRINHA (attenuation correction) and triplanar high-resolution T2-weighted FSE sequences.
- 2.
Whole-body core protocol: axial high-resolution T1-weighted Dixon CAPIRINHA, axial T2-weighted HASTE, and axial SMS DWI sequences.
- 3.
Upper abdominal contrast-enhanced (CE) protocol: T2-weighted fat-saturated FSE, coronal T2-weighted HASTE, T1-weighted VIBE before and after contrast.
- 4.
Postcontrast whole-body T1-weighted VIBE
For all other GI malignancies, we acquire the steps 2–4 as above. In the case of esophageal cancer, we add axial thin T2-weighted HASTE to cover the esophagus.
Inflammatory bowel disease
Patients begin drinking a negative oral contrast solution, usually diluted polyethylene-glycol, about 2 hours before being scanned. Five minutes before the scan, butylscopolamine or glucagon is injected intravenously. The field of view is set to include the abdomen and pelvis. The acquired MRI sequences are
- 1.
Non-CE sequences: axial high-resolution T1-weighted Dixon CAPIRINHA, axial and coronal T2-weighted HASTE, and axial SMS DWI sequences.
- 2.
Coronal and axial T1-weighed VIBE before and after contrast administration ( ).
Esophageal cancer
Clinical overview
Esophageal cancer represents approximately 3% of all cancers and 6% of all cancer-related deaths ( ). Although the overall survival rate in the USA has improved from approximately 5%–20% over the past 40 years, there remains significant room for improvement in terms of prevention, early diagnosis, and treatment ( ). Preventive measures include discouraging smoking and excessive alcohol intake, as well as monitoring for the presence of changes due to gastroesophageal reflux disease. Upper endoscopic screening may help with early diagnosis in countries with a high prevalence of esophageal cancer. Once the disease is present, in addition to upper endoscopy (including endoscopic ultrasound (EUS)), other imaging modalities are necessary to define the disease extent, which is critical for making decisions about the best treatment approach. Since neoadjuvant chemotherapy and radiation therapy are a standard approach in many countries for patients with stages T1N1 or T2/3N0-1 disease, adequate staging is essential to identify eligible patients ( ). Accurate initial staging is also important to identify those who are candidates for surgical resection, either initially, or after neoadjuvant therapy. Adjuvant nivolumab is now approved for patients with stage II or III cancers treated with neoadjuvant chemoradiotherapy and with residual cancer at the time of surgery ( ). As with all cancers, imaging is an essential component of evaluating the response to treatment and making decisions about continuing or changing the treatment approach.
Current imaging modalities
The optimal staging of esophageal cancer is multimodal and often includes an EUS, thoracic and abdominal CE-CT, and/or FDG-PET/CT. Currently, stand-alone MRI has a narrow range of applications in the management of esophageal cancer, mostly because of the limitations in the evaluation of superficial tumors. It also has subpar sensitivity and specificity for N staging when compared to EUS ( ). Potential uses of MRI include the assessment of resectability for tumors that may invade into or extend beyond the esophageal wall, as well as radiotherapy planning ( ; ). MRI may also be useful to resolve lesions deemed indeterminate by PET/CT ( ). Despite the theoretical benefits of MRI’s higher soft tissue contrast resolution, current studies do not support its superiority over CT in the setting of esophageal cancer. EUS is currently the best test for locoregional evaluation, notably for T staging, thanks to its depiction of the individual histologic layers of the esophagus and its assessment of local lymph nodes ( ; ; ). However, cross-sectional imaging remains necessary to identify metastases. This role currently may be filled by CT, PET/CT, and, more recently, PET/MRI.
PET/MRI in esophageal cancer
Staging
Sequential, asynchronous PET/MRI was compared to EUS, CT, and PET/CT in a prospective study, where it demonstrated better performance than CT for T staging and the best overall accuracy for N staging ( ). Nonetheless, this preliminary study had several limitations such as a small sample size, lack of intravenous contrast administration, and a limited MRI protocol. These shortcomings make it hard to generalize these findings. The use of sequential instead of simultaneous PET/MRI also impairs precise coregistration, one of the method’s main advantages. This is detrimental in esophageal cancer, in which T staging relies on detection and interpretation of fine details.
Another small series measured agreement between PET/MRI and PET/CT in esophageal and gastroesophageal junction cancer ( ). The agreement was minimal for T staging ( κ = 0.333), and strong for N and M staging ( κ = 0.849 and κ = 0.871, respectively). The lower agreement in T staging might be explained by the greater soft tissue contrast resolution of MRI, which allows for better delineation of the tumor boundaries and the esophageal wall ( Figs. 14.1 and 14.2 ). The inferior soft tissue contrast resolution from PET/CT might otherwise result in inaccurate T staging. Yet, more malignant lymph nodes were detected by PET/MRI than PET/CT. This study suffered from a lack of pathologic confirmation, making its results of limited relevance.
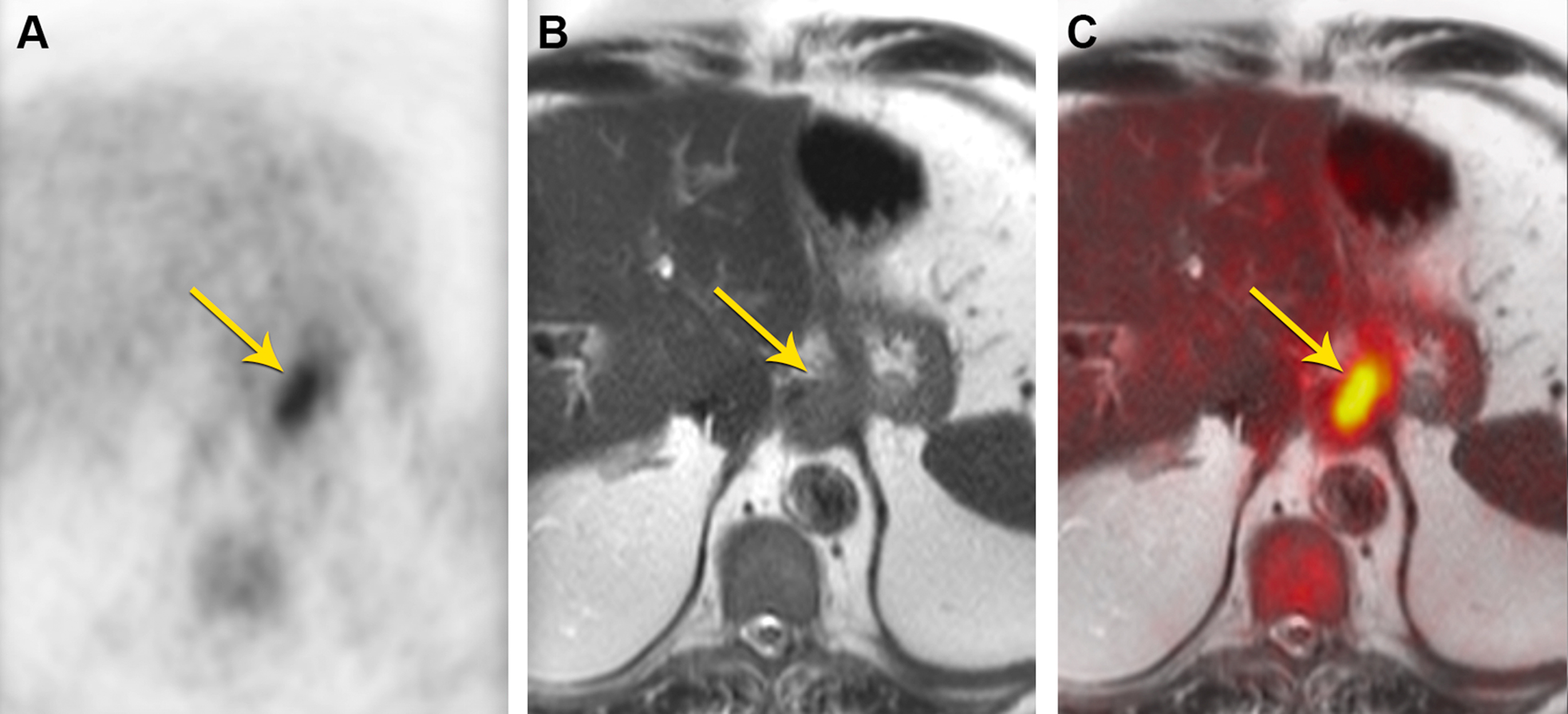
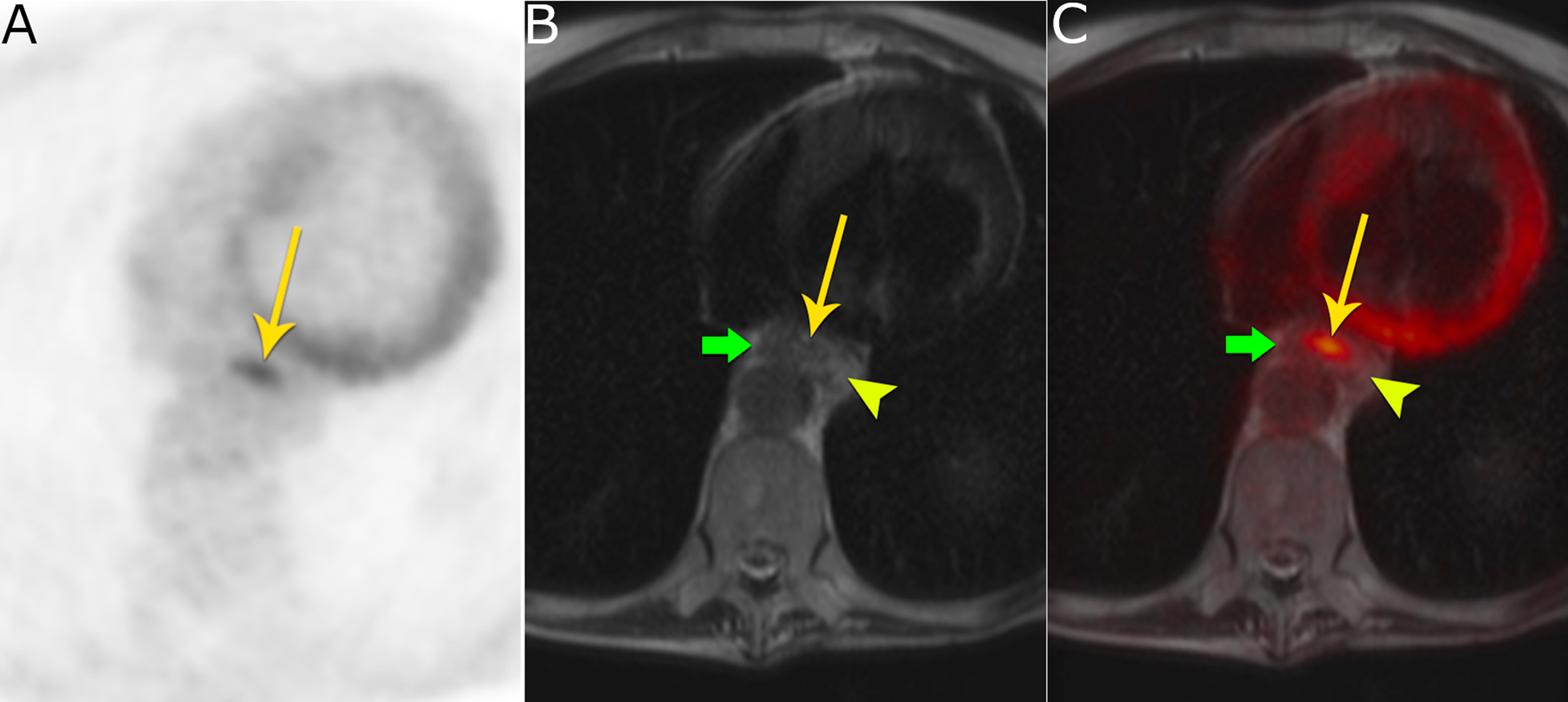
PET/MRI-derived imaging biomarkers
PET/MRI-based imaging biomarkers may provide added prognostic value in esophageal SCC. Measurements of SUV max and calculated apparent diffusion coefficient (ADC) have been shown to correlate negatively with each other. In many other malignancies, higher SUV max values have been shown to carry a worse prognosis. Moreover, the ratio between metabolic tumor volume and minimum ADC significantly correlates to overall and progression-free survival ( ). Thus, besides aiding in the diagnosis and staging of esophageal cancer, PET/MRI might be able to provide both physicians and patients with valuable information regarding the disease prognosis.
In another series with 22 patients, PET/MRI was performed before and after neoadjuvant chemotherapy in patients with gastroesophageal junction adenocarcinoma ( ). In this study, ΔADC (the difference between pre- and posttreatment ADC values) and ΔSUV max (the difference between pre- and posttreatment SUV max ) were correlated with resectability after neoadjuvant treatment. The sensitivity and specificity of PET/MRI for predicting resectability after neoadjuvant chemotherapy were 94% and 80%, respectively. Considering that the Response Evaluation Criteria in Solid Tumors (RECIST) criteria did not present such a correlation in that cohort, this finding was remarkable. This study makes a case for the use of semiquantitative features provided by PET/MRI in the reevaluation of treated patients and its potential use for the selection of surgical or conservative management candidates.
Summary
Based on the limited literature currently available, PET/MRI seems to improve T and N staging when compared to CT and PET/CT in esophageal cancer. T staging might be improved by the increased soft tissue contrast resolution of MRI. This allows for a better estimation of longitudinal and transmural tumor extent. The PET component improves its sensitivity, while dedicated MRI sequences enable the differentiation of the esophageal wall layers. Tumoral features such as high T2-weighted signal intensity, increased DWI signal, and enhancement of postcontrast T1 sequences provide precise anatomic correlates for the PET-detected metabolic abnormalities. Additionally, PET/MRI allows for anatomically resolving pathologic lymph nodes from an adjacent hypermetabolic cancer, which might otherwise obscure them due to excess FDG uptake on PET, resulting in better N staging performance.
EUS remains a superior and irreplaceable test for T and N staging. However, EUS might be used as a proxy for selecting patients that would benefit the most from PET/MRI, i.e., those at greater risk from metastatic involvement. For example, if tissue sampling after endoscopy shows mucosal or submucosal involvement only, without nodal compromise, the distant disease is unlikely and thus PET/MRI should provide little benefit. Conversely, for N+ cases there is an increased risk of metastatic spread, and PET/MRI might help to detect distant foci.
In terms of prognostic evaluation, the inclusion of PET imaging before and after neoadjuvant chemotherapy has the advantage of semiquantitatively measuring response. Those data can be used to estimate survival and define surgical eligibility. Although some of these biomarkers can be measured also with PET/CT, others, like ADC values, can be obtained only through PET/MRI or stand-alone MRI. However, it is conceivable that the superior coregistration from simultaneously acquired PET and MRI in the case of PET/MRI might improve the consistency of measurements and thus the reliability of the calculated differences in SUV and ADC. Therefore, besides an increased diagnostic yield, PET/MRI may also provide useful prognostic information and serve as a tool to stratify patients for surgery or conservative management.
Gastric cancer
Clinical overview
Gastric adenocarcinoma represents approximately 6% of all cancers and is responsible for 8% of cancer-related deaths ( ). Although the incidence of gastric cancer has declined considerably over the past 50 years, it remains a common cancer with significant associated mortality. Incidence remains highest in East Asia with a much lower incidence in western countries. Especially in the latter, the incidence of distal gastric cancer has declined while proximal gastric cancer has increased ( ). This is clinically relevant because potentially resectable gastric cancers arising in the gastroesophageal junction are often treated with the same neoadjuvant chemoradiation therapy as esophageal cancers and therefore have the same stringent imaging requirements ( ). This changing trend of gastric cancer location over time is in part due to a decline in smoking and Helicobacter pylori infections and an increase in Barrett’s metaplasia and obesity, which are primary risk factors for more proximal gastroesophageal junction cancers ( ).
Preventive measures encompass cessation of smoking, treatment of H. Pylori infections, dietary modifications, including those to decrease obesity, long-term suppression of gastritis and gastroesophageal reflux disease, and prophylactic gastrectomy for patients with high-risk genetic alterations (such as germline CDH1 mutations) ( ). For early detection, screening endoscopies have been utilized in countries where the population is at high risk.
Once the diagnosis is established, it is necessary to reliably assess the extent of local, regional, and metastatic disease to develop the most appropriate treatment plan. Neoadjuvant perioperative chemotherapy (most commonly used in western countries), or adjuvant chemotherapy ± radiotherapy after surgical resection (more common in Asia) are used for eligible patients ( ; ). When evaluating metastases, gastric cancers have a relatively higher incidence of peritoneal involvement both synchronously and metachronously, compared to other GI cancers. Current imaging modalities have suboptimal sensitivity to reliably detect peritoneal disease, thus improvements in that regard are critical. Imaging is also crucial for evaluating the response of metastatic disease to treatment.
PET/MRI in gastric cancer
Staging for gastric cancer involves EUS, CT, and/or FDG-PET/CT ( ). FDG-PET/MRI has been scarcely investigated in the management of stomach cancer, with much of the potential benefits yet to be proven in well-designed clinical studies.
The feasibility of MRI for gastric cancer evaluation was studied by comparing T2-weighted HASTE and BLADE sequences, besides T1-weighted sequences, to PET/CT ( ). In a series of 30 patients with gastric cancer, HASTE had the best lesion conspicuity based on reader subjective assessment. The authors propose that HASTE’s subsecond temporal resolution helps to alleviate the motion artifacts introduced by breathing and peristalsis. Overall, FDG-PET/MRI achieved better image quality than FDG-PET/CT.
Regarding staging performance, FDG-PET/MRI was compared to CT in a retrospective study including 42 patients with known gastric cancer. In this setting, PET/MRI outperformed CT in M staging but was not superior in T and N staging. FDG-PET/MRI was superior to CT in the assessment of tumor resectability, with areas under the curve ranging between readers from 0.894 to 0.905 for FDG-PET/MRI, against 0.728 to 0.815 for CT. While these findings further favor a role for FDG-PET/MRI in the staging of gastric cancer, the comparison is not perfect. CT lacks the metabolic input given by PET, which is known to improve the sensitivity for metastases. Moreover, the CT protocol used was unusual, involving acquisition in the left posterior oblique and right decubitus positions, which is difficult to validate externally. Despite FDG-PET/MRI having higher soft tissue contrast than CT, the latter has a superior spatial resolution. Thus, these effects might be counterbalanced and result in the observed similar performance for T staging. In the stomach, specifically, the lower temporal resolution of MRI might have a negative impact due to breathing and peristalsis ( ).
Another study demonstrated a trend toward superior accuracy of FDG-PET/MRI compared to FDG-PET/CT in T staging (77% vs. 58%, respectively) and N staging (54% vs. 34% respectively) in untreated gastric cancers. However, those results were not statistically significant. Just as described in esophageal cancer, there is a negative correlation between ADC values and SUV max for primary gastric lesions ( ). Patients with associated obstruction, bleeding or inflammation were excluded, so there is limited generalizability in those circumstances.
The main limitations faced by hybrid imaging in diagnosing and staging gastric cancer are related to FDG’s weaknesses, namely, false-positive cases due to gastric peristalsis and gastritis and false-negative cases due to small tumor size, mucinous, signet ring cell, or diffuse intestinal histology. On the other hand, FAPI, given lack of uptake in noncancerous stomach, high degree of uptake in gastric cancer subtypes, regardless of their histology, very high tumor to background ratio (11.9 for FAPI vs. 3.2 for FDG) has shown superior performance figures versus FDG (detection rate for primary cancer 100% vs. 36%–50%, and for peritoneal metastases 100% vs. 40%). FAPI will likely play the role of main radiopharmaceutical employed for gastric cancer assessment in the very near future ( ; ).
Summary
PET/MRI had its feasibility validated in gastric cancer, and initial studies show similar or slightly better performance than PET/CT. However, it has to be considered that the sequences used in these studies were potentially suboptimal, and continuous development in MRI protocols leaves the door open for improvements in this regard. Still, the aforementioned studies hint at possible superiority in all of the TNM components, specifically compared to PET/CT for T and N staging, and versus CT for M staging.
Colorectal cancer
Clinical overview
Colorectal cancer accounts for approximately 10% of all cancers and 9% of deaths from cancer ( ). Colonoscopy plays a critical role in prevention, early diagnosis, and tissue sampling in more advanced cases. Imaging is also critical to define the disease stage, thus allowing for the appropriate treatment. One vital application of diagnostic imaging is helping surgeons define candidacy for and the extent of surgery required for both colon and rectal cancers. Moreover, diagnostic imaging is the least invasive method to identify metastatic disease, especially to the liver, distant lymph nodes, and lungs. Additionally, since peritoneal involvement is common, the ability to identify peritoneal involvement is important in defining the optimal approach ( ).
Patients with only local involvement or oligometastatic disease may be eligible for surgery, but those with widespread metastases and/or peritoneal carcinomatosis have no added benefit from invasive procedures in terms of survival. Conversely, isolated or limited metastases – especially in the liver and lungs – are still potentially curable with surgical resection or local ablative therapies. To adequately select patients who will benefit from those techniques, it is essential to define the extent of metastatic disease and regional factors, such as the involvement of critical structures ( ).
Aside from distant disease, the assessment of the loco-regional extent and lymph node involvement is critical. In rectal cancers, for example, neoadjuvant therapy is the primary initial treatment approach for clinically staged T3 or T4 tumors. However, once lymph node involvement is clinically defined, this should be the treatment route regardless of the T stage ( ).
PET/MRI in colorectal cancer
Colorectal cancer staging involves CT of the chest, abdomen, and pelvis, in addition to pelvic MRI for rectal cancer patients ( ). PET/CT, although not routinely indicated, should be considered in patients with potentially curable metastatic disease or those who will proceed to liver-directed therapies ( ). A direct comparison of PET/CT and PET/MRI for the staging of colorectal cancer in 26 patients demonstrated the superiority of PET/MRI, with PET/MRI correctly staging patients who would otherwise be over or understaged by PET/CT in 27% of cases ( ). Arguably, PET/CT’s lacks the soft tissue contrast to accurately determine local extent, thus limiting the T stage evaluation. PET/MRI, on the other hand, has increased contrast resolution to accurately define tumor boundaries for T staging ( Figs. 14.3 , 14.4 , and 14.5 ). Moreover, the morphologic appearance of the tumor on different sequences provides a hint at the histology, such as the characteristic T2 weighted hyperintensity of mucinous neoplasms ( Fig. 14.6 ). N stage evaluation is improved by PET/MRI by better delineation of nodal morphology other than size, such as a heterogeneous internal signal, irregular borders, or round shape ( Figs. 14.7 and 14.8 ) ( , ). Finally, M staging can be improved with the characterization of liver lesions smaller than 10 mm and a better depiction of peritoneal involvement ( ). This is especially important considering that liver metastasectomy has been shown to increase survival in rectal cancer ( ), although the same cannot be said about resection of lung metastases ( ).
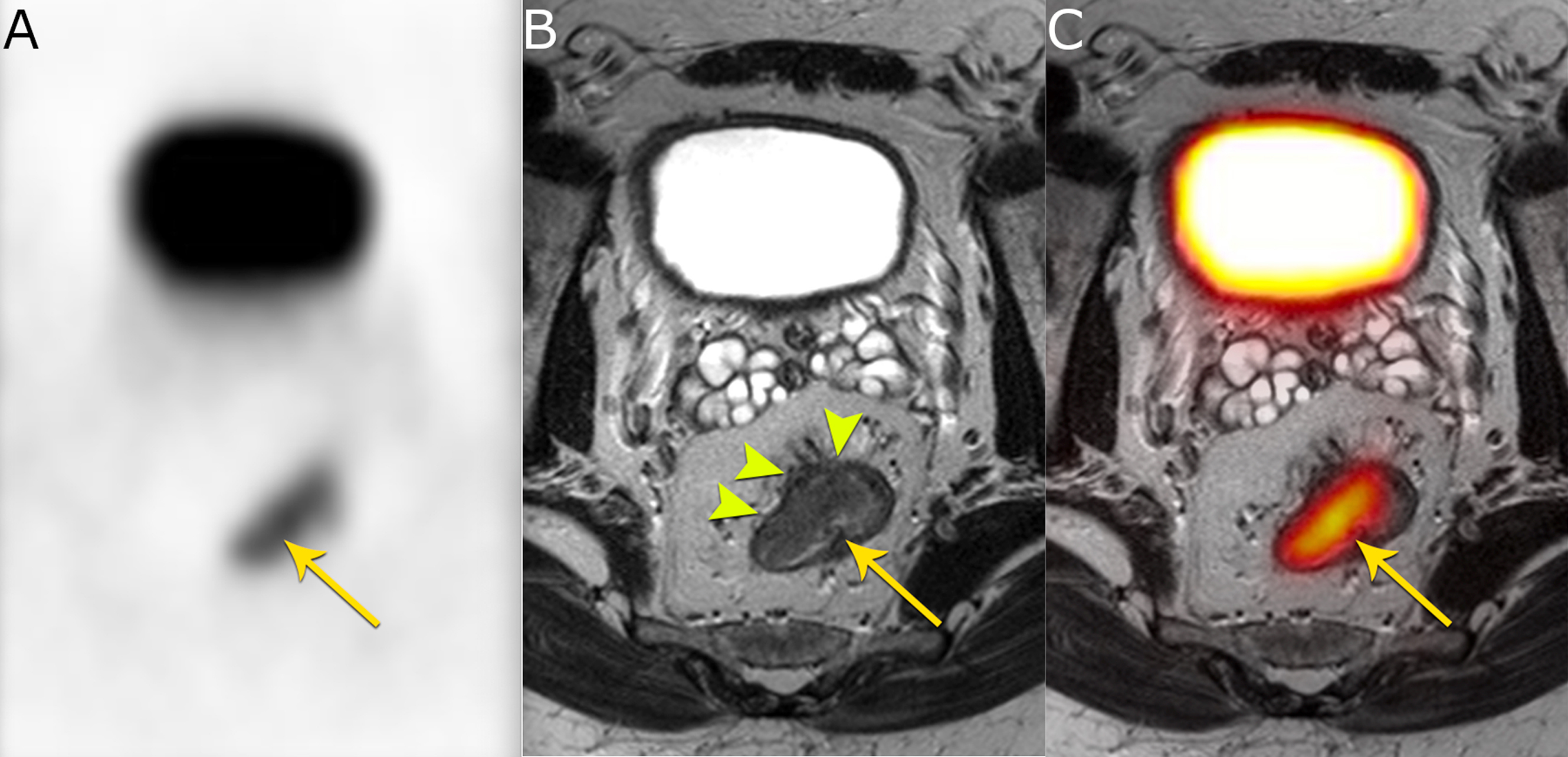
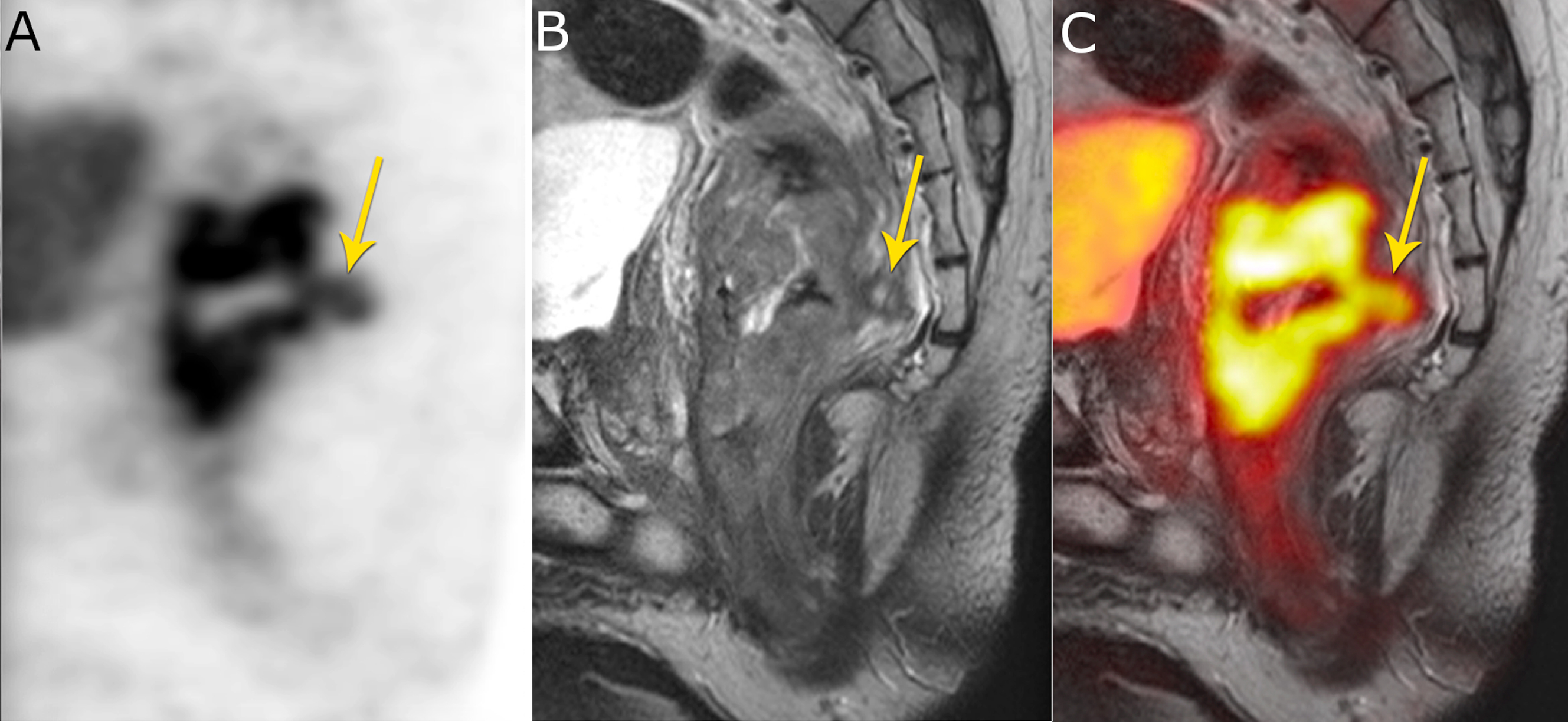
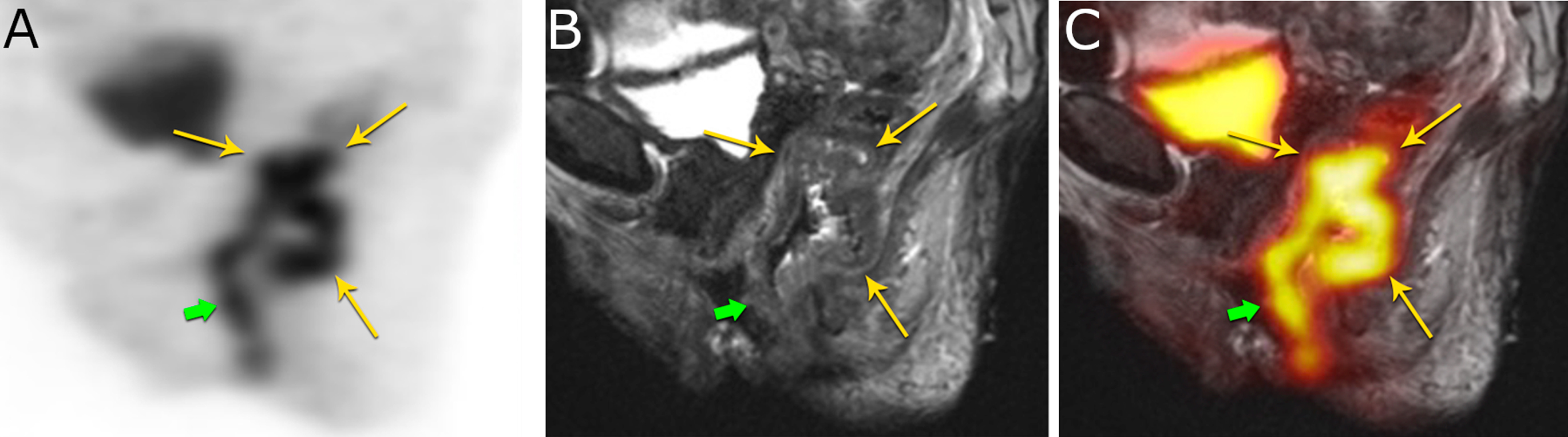
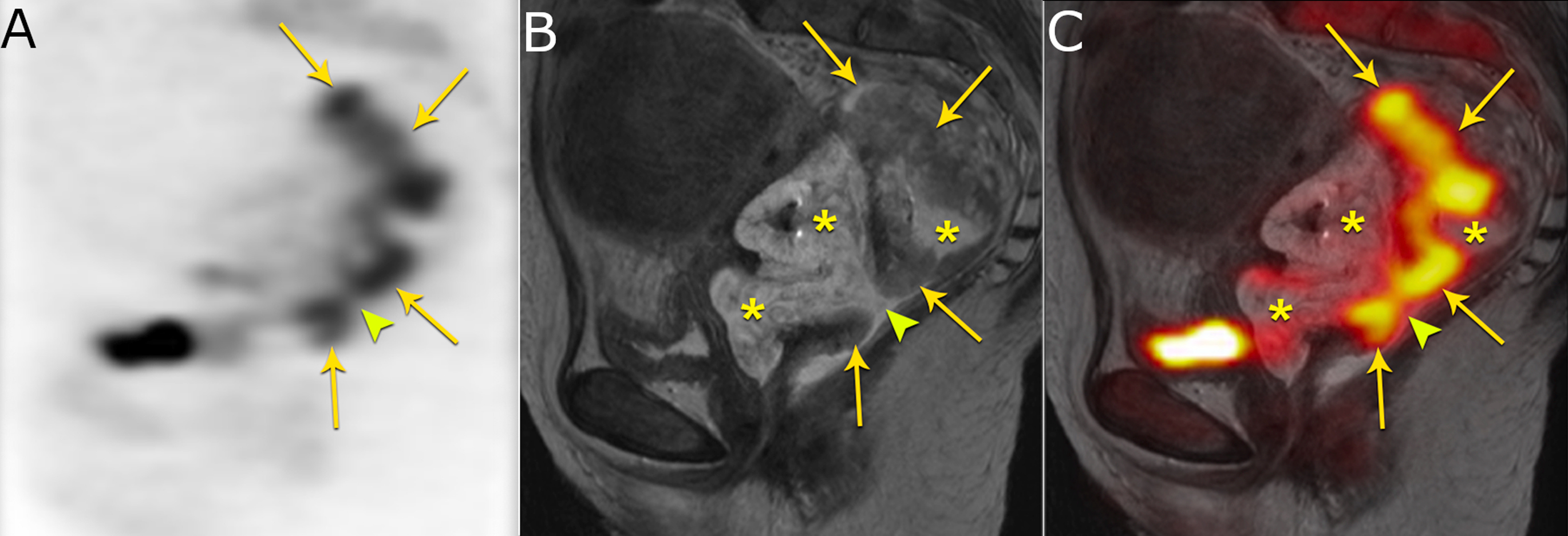
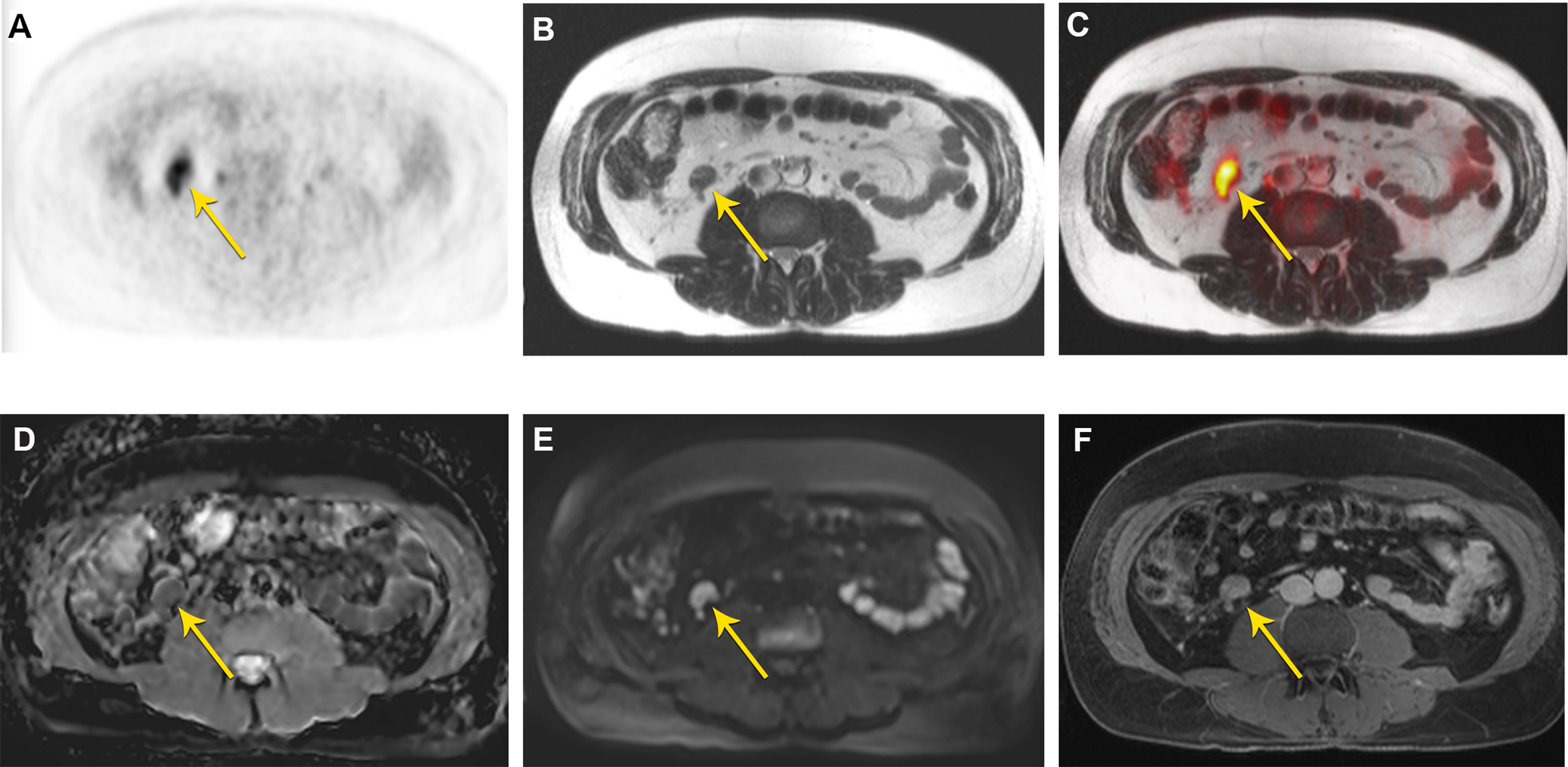
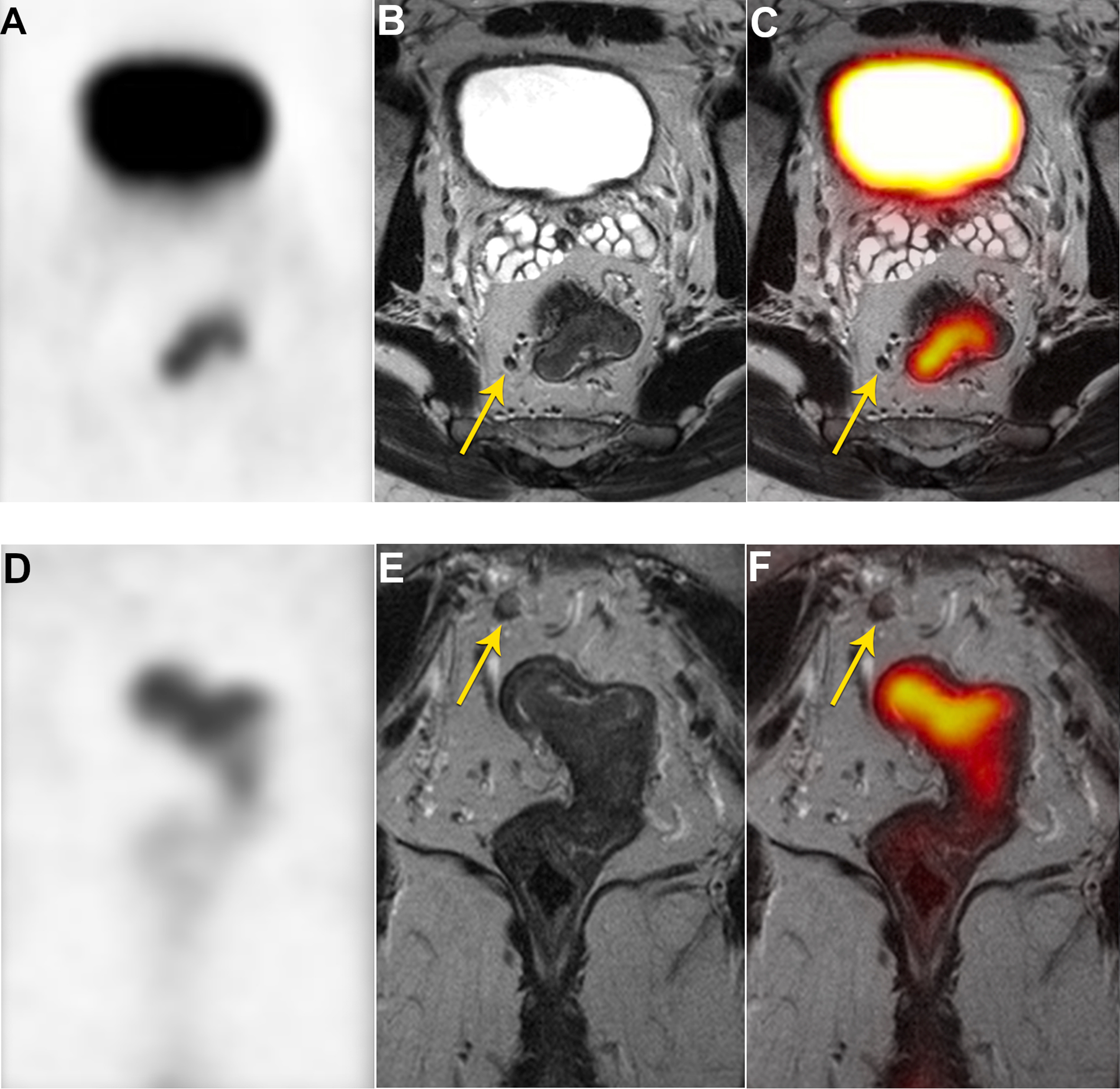
When comparing PET/MRI to CE-CT, impact on patient management has also been shown. A study with 51 patients reported that PET/MRI impacted the management of 22% of the cases ( ). Moreover, PET/MRI was associated with a higher predictive value for malignancy, both on a lesion and on a patient level. These advantages arise mainly from the better depiction of liver lesions and lymph nodes, which in turn is due to better soft tissue contrast and DWI capabilities of MRI.
Even though the increased utility of PET/MRI in the aforementioned examples is determined mainly by MRI advantages over CT, PET/MRI may also improve staging and even facilitate detection when compared to stand-alone MRI ( Fig. 14.9 ). In a retrospective evaluation of 62 cases of rectal cancer, PET/MRI was superior to MRI alone with regards to the evaluation of tumor size, N status, and external sphincter involvement and showed a trend toward better assessment of extramural vascular invasion (EMVI), which has been more clearly demonstrated by a dedicated prospective study ( Figs. 14.10 , 14.11 , and 14.12 ) ( ; ). However, FDG variability uptake of rectal cancer may limit PPV with PET/MRI ( ).
