Introduction
The use of positron emission tomography (PET) in the evaluation of genitourinary malignancies has traditionally been hampered by low tumor uptake and the masquerading effect of excreted radiopharmaceutical activity in the urinary collecting system ( ; ; ; ). However, the advent of positron emission tomography/magnetic resonance imaging (PET/MRI) and new radiopharmaceuticals has mitigated these shortcomings, leading to an expanded utilization of PET in the field of uro-oncology. MRI has several strengths, including exceptional soft tissue contrast as well as the availability of diffusion weighted (DWI) and dynamic contrast-enhanced (DCE) sequences, which allows for more accurate assessment of local genitourinary tumor extent than computed tomography (CT) ( ). MRI also lacks ionizing radiation, which may be beneficial in younger patients with genitourinary cancers who undergo multiple imaging studies for tumor restaging and surveillance ( ). Combining the strengths of MRI with the metabolic information provided by PET offers several advantages as compared to conventional imaging modalities for the assessment of bladder and prostate cancers ( ; ; ). The use of PET/MRI in adrenal malignancies, renal cell carcinoma (RCC), and upper tract urothelial carcinoma (UTUC) is less well-established but the emerging role of PET/CT in these malignancies indicates that PET/MRI will likely play a role in these settings as well ( ; ; ).
Nonetheless, some factors which limit the implementation of uro-oncologic PET/MRI in day-to-day clinical practice include its high cost and complexity as multiple protocols are utilized depending on the type of tumor being evaluated ( ; ; ). Additionally, image quality may be hindered by misregistration or motion artifacts from respiration and bowel peristalsis ( ; ). Furthermore, PET/MRI is limited in the evaluation of lung metastases, which are often encountered in patients with genitourinary malignancies, particularly RCC ( ; ; ).
This chapter discusses the role of PET/MRI in patients with adrenal malignancies, RCC, urothelial carcinoma (UC), and prostate cancer. The utility of PET/MRI in characterizing incidentally detected adrenal and renal lesions is also briefly discussed.
PET/MRI protocol
Several steps should be taken prior to the performance of uro-oncologic PET/MRI. Firstly, patients who undergo FDG or 18 F-fluciclovine PET/MRI should fast for at least 4 hours prior to the examination. On the other hand, patients who undergo PSMA-PET/MRI are not required to fast ( ). The decision to administer a pre-scan diuretic and hydration regimen should be made on a case-by-case basis depending on several patient factors, including hydration status, blood pressure, and the presence of clinically significant bladder outlet obstruction. Utilization of this regimen reduces the accumulation of radiopharmaceuticals in the urinary system, which minimizes scatter artifacts and facilitates the detection of genitourinary lesions ( ). In our institution, 20 milligrams of Furosemide is injected intravenously at least 30 minutes prior to scan initiation. For hydration, oral administration of 1–1.5 liters of water or intravenous injection of 500 milliliters of normal saline is recommended. Following diuretic and fluid administration, patients are instructed to void immediately before proceeding to the PET/MRI scanner. Bladder catheterization is rarely performed given its discomfort and the risk of catheter-associated urinary tract infection. Patients with prostate cancer may undergo additional preparatory steps prior to the start of imaging. These include administration of an intestinal antispasmodic, which reduces motion artifact from intestinal peristalsis, and a rectal enema, which reduces susceptibility artifacts associated with endorectal gas ( ; ). At our institution, the administration of intravenous hyoscine butylbromide and a fleet enema before PET radiopharmaceutical injection is well-tolerated and results in improved image quality.
The PET parameters used for uro-oncologic indications are usually straightforward and fairly standardized. However, the selection of MRI sequences and whether to perform them simultaneously with the PET acquisition or after the PET acquisition tends to vary depending on the type of genitourinary tumor being assessed. In general, a protocol for assessing genitourinary tract malignancies includes whole body T1-weighted Dixon and T2-weighted single-shot fast spin echo (SSFSE) sequences to evaluate for lymph nodal and distant metastases ( ). In selected cases, a whole body short tau inversion recovery (STIR) sequence may be beneficial for the detection of osseous metastases. Additionally, dedicated T2-weighted SSFSE and DWI sequences focused on the liver may be used in genitourinary malignancies that have a propensity to metastasize to the liver. High-resolution T2-weighted and DWI sequences of the pelvis should be performed in patients with prostate and bladder cancer. Lastly, the use of gadolinium should be considered. T1-weighted fat-saturated pre- and dynamic post-contrast sequences of the upper abdomen are obtained in the case of renal, adrenal, and upper tract cancers; meanwhile they are acquired over the pelvis in the case of prostate and bladder cancers. The total scan time should not exceed 60 minutes.
Several radiopharmaceuticals, which are described in more detail in Chapter 2 , are available for uro-oncologic PET/MRI. The most commonly utilized radiopharmaceuticals include FDG, 18 F or 68 Ga-PSMA, 18 F-sodium fluoride (NaF), and 18 F or 11 C-Choline.
Adrenal masses
Clinical overview
Most adrenal masses are adenomas, which are benign tumors that are often diagnosed incidentally on cross-sectional imaging ( ). Nonfunctioning adenomas are considered “do not touch” lesions unless they are >4 centimeters in size or cause compressive symptoms ( ). Functioning adenomas responsible for a clinically significant elevation in hormone levels may be treated with adrenalectomy ( ).
Most malignant adrenal lesions are metastases, often originating from primary carcinomas in the lungs, gastrointestinal tract, breast, and kidneys ( ). Melanoma also frequently metastasizes to the adrenal glands ( ). The diagnosis is usually suspected on the basis of patient history and imaging findings, though biopsy may be pursued in selected cases ( ).
Adrenal cortical carcinoma (ACC) is an aggressive tumor arising from the adrenal cortex. It occurs rarely with an incidence of approximately 1 case per one million person-years ( ). Cross-sectional imaging may help with ACC detection but surgical resection is ultimately required to reach a definitive diagnosis if no metastatic disease is identified ( ). Biopsy of adrenal masses that are suspicious for ACC is generally avoided due to the risk of tumor seeding and the potential inability to differentiate ACC from an adenoma on the basis of a biopsy specimen ( ). FDG-PET/CT plays a role in the staging and restaging of ACC ( ). Aside from surgical resection, other management options include radiofrequency ablation, radiotherapy, and mitotane therapy ( ).
Pheochromocytomas are rare and in most cases benign tumors that originate from the catecholamine-secreting chromaffin cells in the adrenal medulla ( ). Most cases of pheochromocytomas are sporadic but some may be attributed to hereditary syndromes, such as multiple endocrine neoplasia type 2 (MEN2), von Hippel-Lindau disease (VHL), and Neurofibromatosis type 1 (NF1) ( ). Patients may present with refractory hypertension, flushing, palpitations, and headaches. The diagnostic work-up of patients with suspected pheochromoyctoma generally entails acquisition of plasma and 24-hour urine free metanephrine levels followed by anatomic or functional imaging for tumor localization ( ; ; ). Surgical resection is the preferred treatment approach ( ; ). Preoperative alpha and beta blockade is necessary to prevent an adrenergic crisis during surgery.
Utility of PET/MRI
Adrenal nodules are commonly encountered on PET imaging performed for oncologic staging ( ; ). Most incidentally discovered adrenal nodules in patients with no known malignancy represent benign adenomas ( ). While the degree of FDG uptake can help distinguish these benign lesions from malignancies, smaller adrenal nodules are more difficult to characterize with PET ( ). Therefore, adrenal nodule characterization on PET/CT and PET/MRI is also dependent on the detection of intranodular fat. Nodules with an attenuation value of ≤10 Hounsfield units (HU) on CT can be diagnosed confidently as lipid-rich adenomas ( ). However, patients with adrenal nodules measuring >10 HU on CT often have to undergo a dedicated adrenal washout CT study to reach a definitive diagnosis, which results in a less efficient diagnostic work-up( ). With PET/MRI, all lipid-rich and most lipid-poor adenomas demonstrate signal dropout on chemical shift MRI images, which are generally included as part of a standard PET/MRI protocol ( ; ). This potentially obviates the need for patients to return for further imaging work-up. However, metastases from some specific primary malignancies, such as hepatocellular carcinoma and clear cell RCC, may contain microscopic fat and therefore demonstrate signal dropout on out-of-phase sequences Fig. 12.1 ( ). Therefore, correlation of the imaging features of the adrenal lesion with those of the primary malignancy is fundamental.
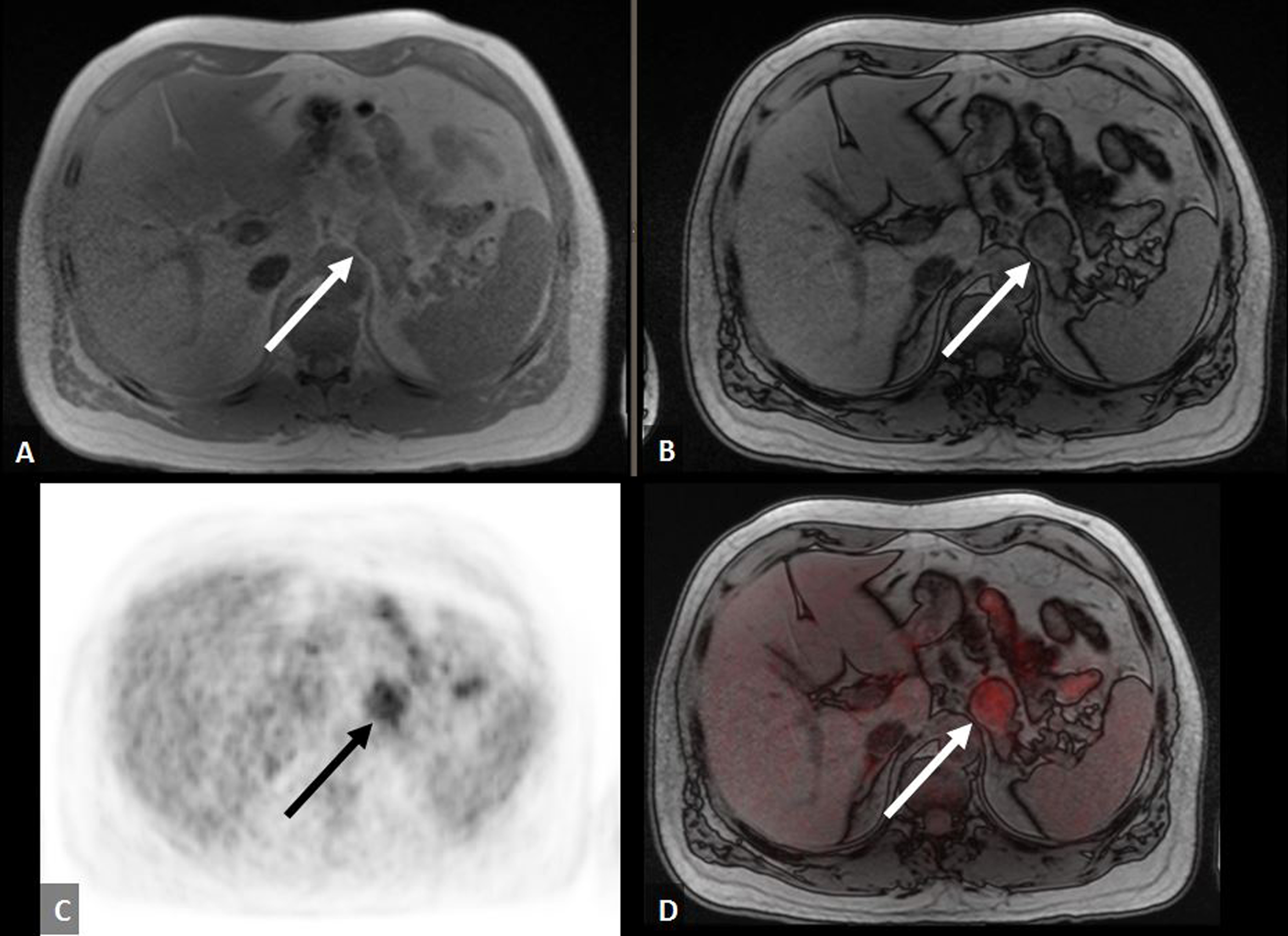
Although adrenal washout CT is considered superior to chemical shift MRI images for the characterization of adrenal nodules, it is not feasible to perform this routinely as part of a standard PET/CT protocol due to time and resource constraints. In a study of 173 patients with 16 adrenal incidentalomas, PET/MRI correctly classified 13 out of 16 adrenal incidentalomas, whereas PET/CT correctly classified only 3 of them ( ). Adrenal metastases can often be distinguished from adrenal adenomas on the basis of heterogeneous signal and similar uptake to other metastatic lesions as in Fig. 12.2 .
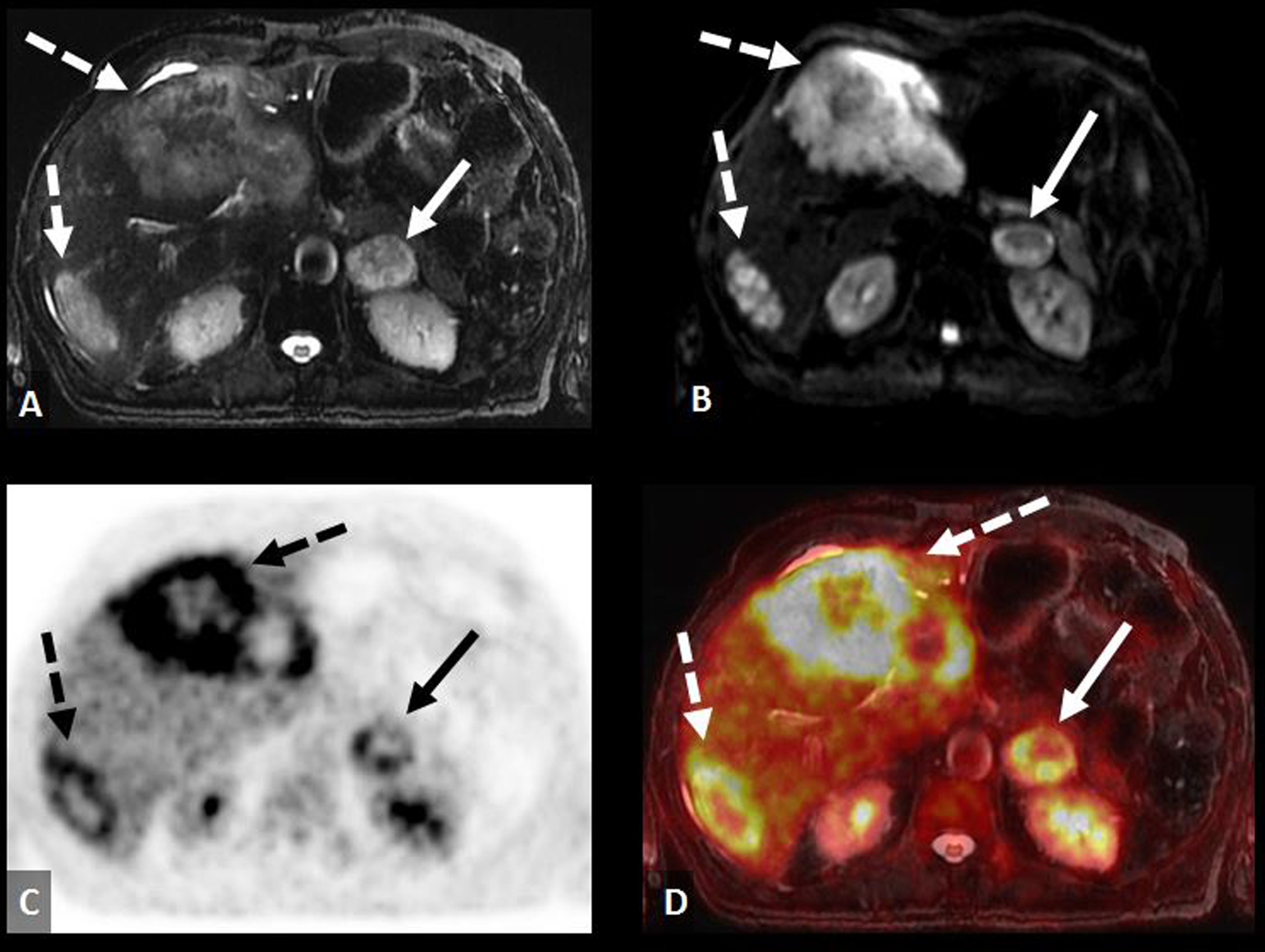
PET has been shown to play a complementary role to diagnostic CT for the initial staging of ACC and detection of locally recurrent disease. In a study of 28 patients with ACC, PET in combination with diagnostic CT detected 89% of lung metastases, 75% of peritoneal and abdominal lymph node metastases, 70% of bone metastases, and 60% of liver metastases ( ). Additionally, PET in combination with diagnostic CT identified 54% of locally recurrent tumors. PET/CT has also demonstrated the potential to identify recurrent disease following radiofrequency ablation (RFA) of ACC lung metastases. In a study consisting of 68 patients with either primary lung cancer or lung metastases, three of whom had lung metastases from ACC, focal or rim uptake on post-RFA FDG-PET/CT was associated with disease recurrence ( ). Although there are no studies that evaluate the use of PET/MRI for ACC staging, it may be advantageous over PET/CT for the detection of liver and peritoneal metastases as well as locally recurrent disease due to its superior soft tissue contrast.
Somatostatin receptor (SSTR) PET/CT performs well for pheochromocytoma detection ( ; ). In a study of patients with pheochomocytomas and paragangliomas, Gallium-68 ( 68 Ga) DOTATATE-PET/CT and FDG-PET/CT identified sites of disease with 96.2% and 91.4% accuracy, respectively ( ). However, the degree of radiopharmaceutical uptake corresponding to the sites of disease was significantly higher with 68 Ga-DOTATATE as compared to FDG-PET/CT with median maximum standardized uptake values (SUVmax) of 21 and 12.5, respectively. Therefore, 68 Ga-DOTATATE is considered the preferred imaging modality for the detection of pheochomocytoma. The excellent performance of PET/CT for pheochromocytoma detection suggests that PET/MRI might be used effectively for this purpose.
Renal cell carcinoma
Clinical overview
Kidney cancer accounts for 2.2% of newly diagnosed cancers and 1.8% of cancer-related deaths annually worldwide ( ). In the United States (U.S.), approximately 76,000 new cases of kidney cancer are expected in 2021 ( ). Over 90% of kidney cancers are diagnosed as RCC ( ). Risk factors for RCC include obesity, hypertension, cigarette smoking, acquired cystic kidney disease, and hereditary syndromes, such as VHL and Tuberous Sclerosis (TS) ( ). The most common histologic subtype of RCC is clear cell (∼75%) followed distantly by papillary (∼15%) and chromophobe (∼5%) ( ). The clinical course of RCC is variable with some tumors progressing slowly and others rapidly with early-onset metastatic disease ( ).
RCC is typically detected incidentally on imaging. A minority of patients with RCC present with symptoms, such as hematuria and flank pain, that prompt a diagnostic work-up ( ). Patients who are good surgical candidates and have imaging findings strongly suggestive of a resectable RCC often undergo surgery without a preoperative biopsy for diagnostic confirmation ( ). However, there are several scenarios in which image-guided percutaneous biopsy of renal masses is performed, particularly in patients who are poor surgical candidates and prior to percutaneous local ablative therapies ( ). Staging is typically performed with contrast-enhanced CT ( ). MRI may also be helpful, particularly for the detection of local vascular invasion and liver metastases.
The treatment of choice for localized surgically resectable RCC is radical or partial nephrectomy with curative intent ( ; ). In patients who are poor surgical candidates or have small tumors, percutaneous ablative treatments, such as cryoablation or RFA, or active surveillance may be considered ( ). Patients with unresectable RCC or metastatic disease are usually managed medically with targeted therapies and immune checkpoint inhibitors ( ).
Utility of PET/MRI
As with adrenal nodules, kidney lesions are often encountered incidentally on PET imaging performed for oncologic staging ( ). Low-grade RCCs may demonstrate minimal to no FDG uptake and can be mistaken for benign lesions ( ). Additionally, physiologic urinary excretion of the radiopharmaceutical may obscure solid renal masses ( ). Therefore, the detection and characterization of incidental renal lesions on PET/CT and PET/MRI is mostly reliant upon diagnostic CT and MRI features, respectively. PET/MRI is superior to PET/CT for the characterization of incidental renal lesions. In a study of 78 patients with 154 incidentally detected renal lesions, only 13 renal lesions remained indeterminate at PET/MRI as opposed to 25 at PET/CT ( ). This is explained by the availability of T2-weighted sequences, which aid in the identification of small cysts that would otherwise be difficult to characterize on CT, and the availability of fat suppression sequences, which facilitate the detection of small foci of intralesional fat that would otherwise also be difficult to visualize on CT ( ).
Although FDG-PET/CT is not currently recommended for the diagnosis and initial staging of RCC, it can be used effectively for postoperative surveillance and restaging ( ). In a meta-analysis consisting of 14 studies, FDG-PET/CT had a pooled sensitivity of 86% and specificity of 88% for the detection of recurrent and metastatic RCC lesions following treatment ( ). When using PET/CT for RCC restaging, false-negative cases are largely due to the small size of lesions and limited spatial resolution of the scanner ( ). On the other hand, false-positive cases generally occur due to concomitant infection, postoperative scarring, or radiation-induced inflammation.
FDG-PET may help predict the histologic subtype of RCCs and determine prognosis. In a study of 93 patients with renal lesions, high-grade clear cell RCC and papillary RCC demonstrated significantly higher mean SUVs than normal renal parenchyma ( ). In contrast, the mean SUVs of low-grade clear cell RCC and chromophobe RCC did not significantly differ from normal renal parenchyma Fig. 12.3 . Furthermore, Lee et al. demonstrated that the median SUVmax of primary RCC lesions was significantly higher in patients with metastatic disease as opposed to patients without metastatic disease (5.0 vs. 2.6) ( ).
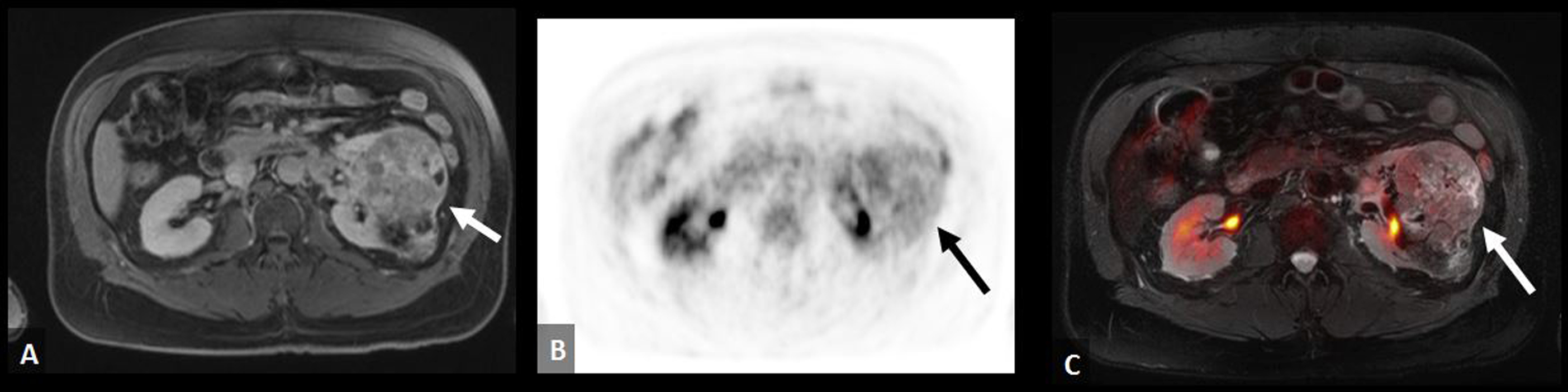
PET/CT has also been shown to be effective for discriminating RCC tumor thrombus from bland thrombus. This distinction is important as it has implications on surgical approach, prognosis, and staging ( ). In a study of 60 patients with RCC, PET/CT correctly identified all seven cases of tumor thrombus in the inferior vena cava ( ). Another study demonstrated that the mean SUVmax for bland thrombus was 3.2 as opposed to 6.0 for tumor thrombus ( ).
Another application of PET/CT in patients with RCC is evaluating the therapeutic response to targeted therapies, such as tyrosine kinase inhibitors (TKIs). Counterintuitively, lesions that respond well to TKIs generally show minimal to no decrease in size following treatment ( ). Therefore, measuring change in tumor size on anatomic cross-sectional imaging is an unreliable method for monitoring the response to TKI treatment. In a meta-analysis of patients with metastatic RCC, those with lesions that demonstrated a reduction in SUVmax following TKI treatment were found to have a higher survival rate ( ).
To date, there are no studies that investigate the use of PET/MRI in patients with RCC. However, the emerging role for PET/CT in the restaging of RCC following treatment, RCC prognosis determination, differentiation of RCC tumor thrombus from bland thrombus, and assessment of response to targeted therapy, suggests that PET/MRI might play an essential role in these areas as well.
Urothelial cancer
Clinical overview
There were approximately 573,000 new bladder cancer cases and 213,000 deaths related to bladder cancer worldwide in 2020 ( ; ). Risk factors for UC include cigarette smoking, advanced age, male sex, and occupational exposure to carcinogens ( ). UC is the most common histologic subtype of bladder cancer ( ). Approximately 90% of UC cases arise from the bladder and can be classified on the basis of detrusor muscle invasion as muscle-invasive bladder cancer (MIBC) or non-muscle invasive bladder cancer (NMIBC) ( ). The remaining 5%–10% of UCs arise from the renal pelvis or ureter and are referred to as UTUC ( ).
UC is most commonly discovered during a diagnostic work-up for hematuria, which generally consists of urine cytology, cystoscopy, and CT urography for assessment of the upper tract ( ; ; ). Less commonly patients present with irritative voiding symptoms or flank pain ( ). The diagnosis of bladder UC and UTUC is established on the basis of tissue sampling, which can be obtained at the time of cystoscopy or ureteroscopy, respectively ( ; ). Cross-sectional imaging is generally performed in patients with MIBC or UTUC to assess for metastatic disease prior to treatment initiation (S. J. ; ). Traditionally, PET/CT has been considered a suboptimal imaging modality for the staging of UC. However, in recent years it has been used more frequently and has shown the ability to alter the treatment approach of these cancers (S. J. ; ).
The treatment of UC varies according to its location (bladder or upper tract), depth of infiltration (muscle-invasive or non-muscle invasive), and stage (localized or metastatic). NMIBC is usually treated with transurethral resection of bladder tumor (TURBT) only or TURBT followed by intravesical Bacillus Calmette–Guérin therapy ( ). For localized MIBC, neoadjuvant chemotherapy followed by cystectomy is the preferred treatment approach ( ). The treatment approach for localized UTUC varies but generally entails radical nephroureterectomy with or without neoadjuvant chemotherapy ( ). Metastatic disease is generally treated with systemic chemotherapy ( ).
Utility of PET/MRI
The detection of UC in the bladder or upper urinary tract with FDG-PET is often limited by the obscuring effect of excreted FDG activity ( ). This effect can be mitigated by adequate hydration and forced diuresis prior to scan initiation Fig. 12.4 . Despite this shortcoming, FDG-PET/CT had a sensitivity of 83% for primary lesion detection in a study of 49 patients with UTUC ( ). This indicates that UC detection with FDG-PET imaging can be performed, albeit with close correlation to CT or MRI images.
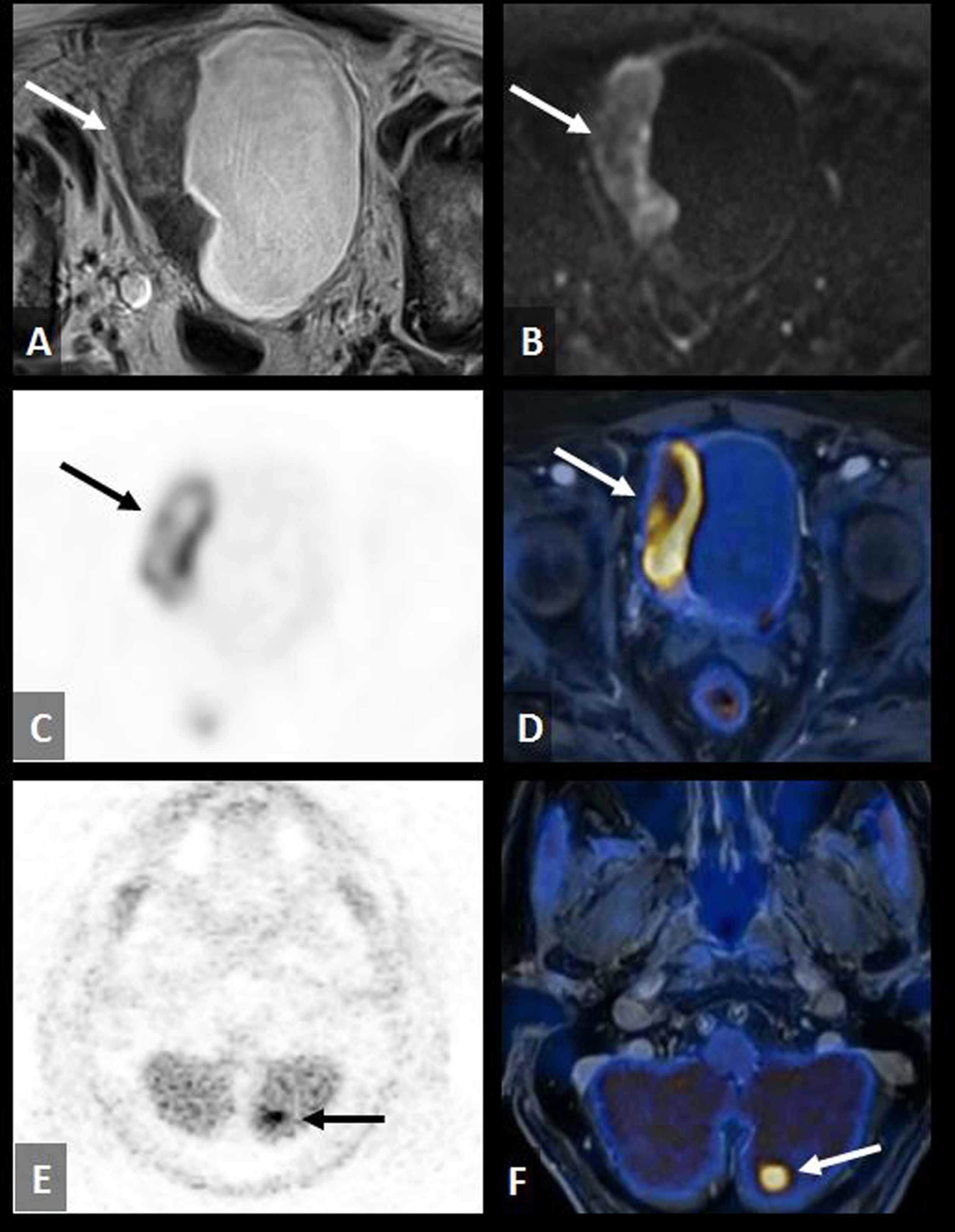
PET/MRI holds promise for assessing the local extent of bladder cancer and detecting local bladder cancer recurrence due to its excellent soft tissue contrast Fig. 12.5 . Pelvic MRI utilizing DCE and DWI sequences has been shown to accurately distinguish MIBC from NIMBC using a five-point Vesical Imaging-Reporting and Data System (VI-RADS) score ( ). Higher VI-RADS scores indicate a higher probability of detrusor muscle invasion. In a study of 340 patients with bladder cancer, a VI-RADS score of three or greater had a sensitivity of 87.1% and specificity of 96.5% for the detection of detrusor muscle invasion ( ). FDG activity may also help identify extravesical tumor extension into adjacent organs, such as the prostate, vagina, rectum, and uterus ( ).
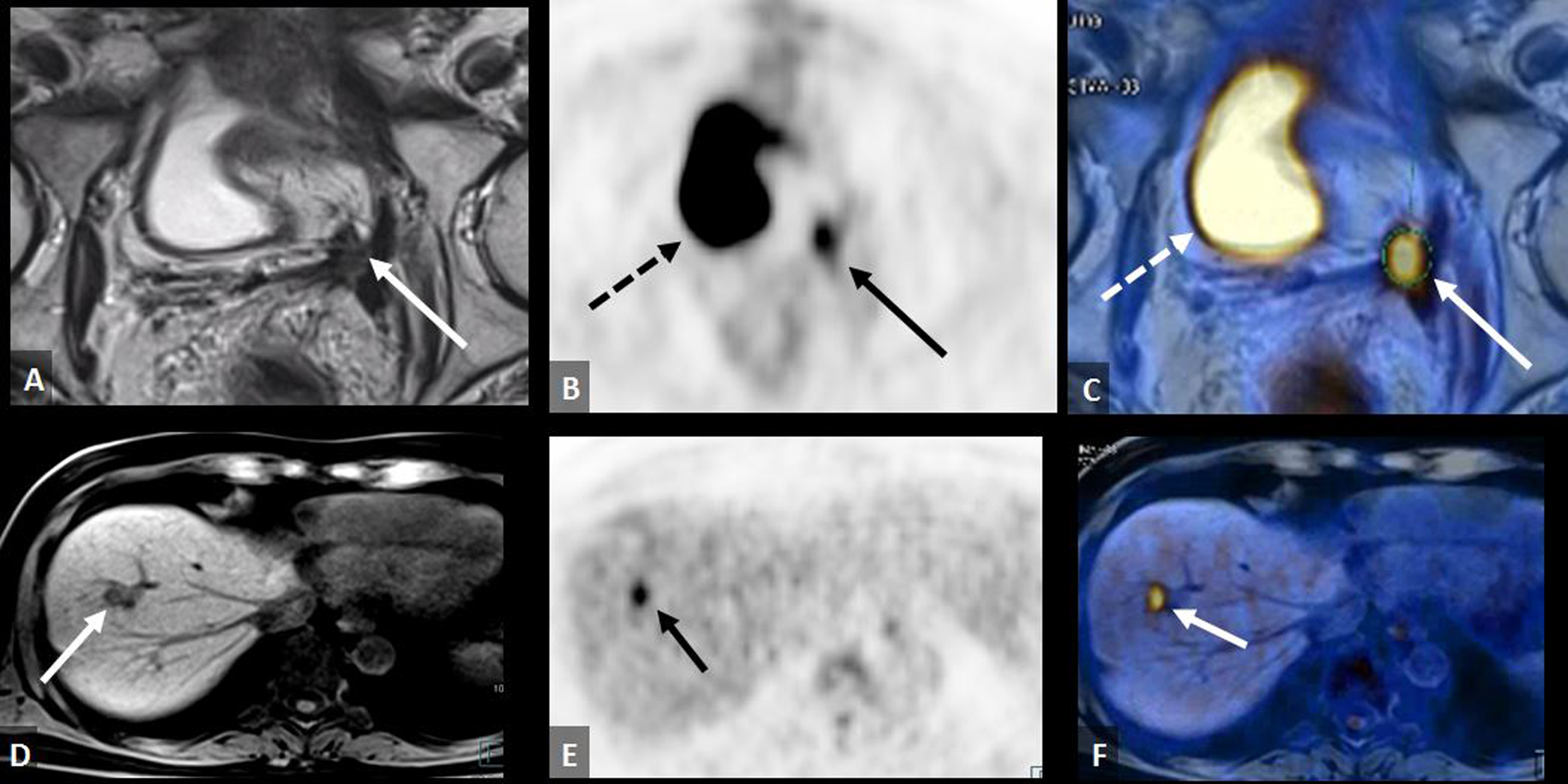
FDG-PET/MRI outperforms conventional morphologic imaging for the detection of bladder cancer metastases. In a study of 15 patients with bladder cancer, PET/MRI identified 36% more metastatic lesions than stand-alone CT ( ). Rosenkrantz et al. demonstrated that PET/MRI improved the characterization of findings that were otherwise equivocal for bladder cancer metastases on stand-alone MRI ( ). However, to date, there are no studies that compare the performance of PET/MRI to PET/CT for the identification of bladder cancer metastases. In a meta-analysis, PET/CT had a pooled sensitivity of 82% and specificity of 89% for the detection of bladder cancer metastases ( ).
PET/CT has also been used to monitor response to neoadjuvant chemotherapy and distinguish responders from non-responders ( ; ). PET/MRI could play the same role as PET/CT in this regard. In a study of 31 patients with metastatic UC receiving chemotherapy, PET responders were observed to have a better progression free survival ( ). Patients with tumors that demonstrated a >20% reduction in FDG activity without the development of any new sites of abnormal uptake following chemotherapy were classified as PET responders in this study.
PET/MRI is an effective imaging modality for the detection of bladder cancer metastases and has the potential to accurately assess the local extent of bladder cancer, which may obviate the need for patients to undergo multiple imaging studies for initial staging.
Prostate cancer
Clinical overview
Prostate cancer has the second highest incidence among all cancers in males worldwide with approximately 1.4 million newly diagnosed cases annually ( ). In the U.S., it is the most common cancer type and second highest cause of cancer-related mortality in males ( ). In 2021, prostate cancer is expected to account for approximately 249,000 new cases and 34,000 deaths ( ). 46 The risk factors for prostate cancer include age, African American descent, family history, and hereditary syndromes, such as Lynch Syndrome and breast cancer predisposition gene (BRCA) mutations ( ).
Prostate cancer is usually detected in the setting of an elevated prostate-specific antigen (PSA) level and palpable prostatic mass on digital rectal exam ( ). A minority of patients with prostate cancer present with symptoms, such as difficulty voiding or pelvic pain ( ). Patients with elevated PSA levels are usually referred for transrectal ultrasound-guided prostate biopsy ( ). However, over the last several years multiparametric MRI (mpMRI) of the prostate has increasingly been utilized to identify clinically significant prostate cancer (csPCa) in patients with elevated PSA levels prior to making a decision of whether or not to proceed with biopsy ( ; ; ). This change in diagnostic approach has been shown to reduce the number of unnecessary prostate biopsies and improve the detection of csPCa ( ). The Gleason score is used to assign a prostate cancer grade ( ; ). Functional and anatomic imaging also plays a critical role in prostate cancer staging and the localization of biochemically recurrent disease after radiotherapy or prostatectomy. There is an emerging role for PSMA-PET for these indications ( ).
The treatment of localized disease varies and may include active surveillance, radiation therapy with or without androgen deprivation therapy (ADT), and radical prostatectomy ( ). The choice of therapy depends on several factors, such as tumor histology and patient life expectancy ( ). Local recurrence after prostatectomy can be treated with salvage radiotherapy with or without ADT ( ). If there is local recurrence following radiation therapy, a salvage prostatectomy may be discussed in selected cases, but ADT is also an option ( ). The treatment of metastatic disease has evolved over the last several years. ADT remains the mainstay of treatment, but in the castration-sensitive scenario, the use of additional drugs, such as second-generation antiandrogens or chemotherapy, has become an option ( ). In the castration-resistant scenario, other treatment modalities, such as radiopharmaceuticals (radium-223 and Lutetium-177-PSMA), Poly (ADP-ribose) polymerase (PARP) inhibitors, and immunotherapy, may be employed ( ).
Utility of PET/MRI
PSMA-PET/MRI may be used for csPCa detection but it is unclear if it offers any benefit as compared to mpMRI. A study of 32 patients demonstrated that PSMA-PET/MRI and mpMRI had similar specificities of 88% and 90% for csPCa detection, respectively ( ). However, PSMA-PET/MRI had a higher sensitivity than mpMRI for this same purpose (73% vs. 69%). In contrast, Margel et al. reported that PSMA-PET/MRI improved the specificity but not the sensitivity of csPCa detection as compared to mpMRI ( ). Ferraro et al. demonstrated that PSMA-PET/MRI had a high sensitivity (96%) and negative predictive value (93%) for the detection csPCa in patients with elevated PSA levels and lesions assigned a Prostate Imaging-Reporting and Data System (PI-RADS) score of ≥3 on mpMRI ( ).
PSMA-PET/MRI may play an essential role in the local staging of prostate cancer. In a study of 74 patients with prostate cancer, mpMRI detected extracapsular extension of tumor ( Fig. 12.6 ) and seminal vesical invasion ( Fig. 12.7 ) more accurately than PSMA-PET/CT ( ). As compared to mpMRI, PSMA-PET/MRI was shown to have a higher sensitivity (69% vs. 46%) but decreased specificity (90% vs. 94%) for the detection of extracapsular extension ( ).
