Fig. 10.1 First drawing of a human ventricular system. Drawing by Leonardo da Vinci from the Schlossmuseum in Weimar, shown here in modified form. This is the first drawing of a human ventricular system based on an actual dissection. Note the accurate depiction of the aqueduct and interventricular foramen. The fourth ventricle terminates blindly, however, apparently because Leonardo did not question the prevailing Galenic doctrine, which held that the CSF space communicates with the cribriform plate via the infundibulum and “drains” through the plate.
This brief historical review is based on a detailed account by Aschoff et al (1999) and includes portions of that article.
10.2 Fundamentals of Anatomy and Physiology
10.2.1 Functions of the CSF
The primary function of CSF is to protect the brain, which “floats” in the fluid medium. This buoyancy effect reduces the relative weight of the brain by more than 90%. Additionally, the flow of CSF provides an alternative route for the transport of ions, molecules, and proteins to and from the brain cells. Finally, CSF functions as a mediator for intracranial compliance. The intracranial compartments consist basically of brain tissue, blood, and CSF. If one of these compartments increases in volume, due for example to a tumor or parenchymal hemorrhage, the resulting mass effect can be offset to some degree by a decrease in the CSF volume, thus preventing an immediate rise in intracranial pressure. The principle that the sum of the three compartments (blood, brain, CSF) is constant, so that a volume change in one compartment evokes compensatory volume changes in the others, is known as the Monroe–Kellie doctrine.
Note
Monroe–Kellie doctrine: Changes in the volume of intracranial blood, CSF, or brain evoke a compensatory volume change in the other compartments.
10.2.2 Anatomy of the CSF Spaces
The intracranial CSF spaces are formed by the four ventricles, the basal cisterns, and the subarachnoid spaces over the convexity of the brain. The two lateral ventricles are located in the cerebral hemispheres, each consisting of a frontal horn, cella media, occipital horn, and temporal horn. The junction between the temporal and occipital horns is called the antrum or trigone. Each of the lateral ventricles communicates with the third ventricle through the intraventricular foramen (foramen of Monro). The third ventricle is a midline structure that communicates with the fourth ventricle via the cerebral aqueduct (Sylvian aqueduct). Finally, the fourth ventricle communicates with the outer CSF spaces through the lateral apertures (foramina of Luschka) and the posteromedial median apertures (foramen of Magendie; ▶ Fig. 10.2).
Fig. 10.2 Anatomy of the ventricular system. Diagrammatic representation.
1–4 = Lateral ventricle
1 = Frontal (anterior) horn
2 = Cella media
3 = Trigone and occipital horn
4 = Temporal horn
5 = Interventricular foramen (of Monro)
6 = Third ventricle
7 = Cerebral (Sylvian) aqueduct
8 = Fourth ventricle
9 = Median apertures (foramina of Luschka)
10 = Lateral aperture (foramen of Magendie)
10.2.3 Production and Transport of CSF
Most CSF is produced by the choroid plexus of the fourth ventricle. Although this is the “classic” site of CSF formation, it has been suggested that CSF is also produced outside the choroid plexus by the parenchymal capillaries or ependyma.
The volume of circulating CSF in an adult is approximately 150 mL, and one-third of that volume is intracranial. Approximately 300–500 mL of CSF is produced daily, with the result that the total CSF volume is “exchanged” about 2–3 times each day. The reabsorption of CSF occurs mainly at the arachnoid (Pacchionian) granulations. These are sites where the arachnoid membrane evaginates into the dural venous sinuses, allowing CSF to exit the subarachnoid space and enter the venous circulation. The partial absorption of CSF via the choroid plexus and via capillaries or small lymphatics has also been postulated or described. It is also believed that some CSF is reabsorbed in spinal root sleeves. Thus, the classic “life cycle” of CSF from its production to clearance is as follows:
production in the choroid plexus (here: in the lateral ventricle) → intraventricular foramen → third ventricle → cerebral aqueduct → fourth ventricle → lateral and median apertures → arachnoid granulations → venous system.
The driving force behind CSF circulation is probably the pulsations of the choroid plexus and brain parenchyma that occur during the alternation between systole and diastole. During systole, blood is pumped into the cranial interior through arterial vessels. Because the venous volume remains almost constant, CSF flow is initially directed through the foramen magnum toward the spinal canal to compensate for the volume influx. Approximately 50 to 100 ms later, CSF flow is observed within the ventricular system, particularly in the aqueduct. ▶ Fig. 10.3 illustrates CSF movements at different points in the cardiac cycle.
Fig. 10.3 Dynamic imaging of CSF flow. Phase-contrast sequences acquired at different intervals from the R-wave in the electrocardiogram (ECG) provide a dynamic record of CSF flow during one cardiac cycle. The images document CSF flow registered at various points in the cardiac cycle. Note the variable direction of CSF flow at different times and locations (with kind permission of Prof. C. Groden, Department of Neuroradiology, Mannheim University Hospital). (a) Time 1. (b) Time 2. (c) Time 3. (d) Time 4.
A pressure gradient between the sites of CSF production and absorption has also been discussed as a possible driving force for CSF flow. This is unlikely, however, based on theoretical considerations and the results of dynamic MR imaging of CSF movements.
10.2.4 CSF Equilibrium and Hydrocephalus
Hydrocephalus results from an imbalance between the production, transport, and reabsorption of CSF. This imbalance leads to an increase in the CSF volume, a rise of CSF pressure, and enlargement of the CSF spaces. The rise in CSF pressure leads to increased intracranial pressure.
Clinical manifestations Hydrocephalus in infants and small children is manifested by an increase in head circumference with bulging, enlarged fontanelles and engorged superficial veins. Adults present mainly with the signs and symptoms of increased intracranial pressure such as restlessness, headaches, nausea and vomiting, decreased vigilance, concentration difficulties, fatigue, and forgetfulness. Later symptoms may include diplopia, gait disturbance, and bladder dysfunction.
Note
Symptoms of hydrocephalus are:
Restlessness
Headaches
Nausea
Vomiting
Impaired concentration
Fatigue
Diplopia
Gait disturbance
Bladder dysfunction
Pathology Types of hydrocephalus:
Compensated hydrocephalus: Because a rise of CSF pressure due to an increased pressure gradient between the CSF and venous system is associated with increased absorption, a new equilibrium between CSF production and absorption can be established at a higher pressure level. This condition is called “compensated” hydrocephalus.
Hypersecretory hydrocephalus: Hydrocephalus due to the excessive production of CSF is a very rare condition found in patients with a choroid plexus papilloma or carcinoma. It is likely that hypersecretory (overproduction) hydrocephalus may also be caused by deep cerebral venous thrombosis.
Malresorptive hydrocephalus: Hydrocephalus due to decreased absorption of CSF may have two main causal mechanisms. First, actual absorption may be decreased while CSF flow is unobstructed. This type may be described as “malresorptive,” “unresorptive,” or “hyporesorptive.” Hydrocephalus due to decreased CSF absorption is also commonly referred to as communicating hydrocephalus. Possible causes include subarachnoid hemorrhage, meningitis, and increased intracranial venous pressure.
Obstructive hydrocephalus: In a second mechanism, CSF flow may become obstructed between its sites of production and absorption. The obstruction may be anywhere in the CSF pathway but most commonly occurs at sites of physiologic narrowing such as the intraventricular foramina and cerebral aqueduct. This type is also known as noncommunicating hydrocephalus.
Hydrocephalus ex vacuo: True hydrocephalus caused by an imbalance between CSF production, transport, and absorption requires differentiation from ventricular enlargement due to brain atrophy or other events causing loss of brain tissue such as trauma, infarction, or tumor resection. Of course, a diffuse or circumscribed loss of brain tissue does not evoke a corresponding shrinkage of the intracranial volume. Instead, the CSF flows into and occupies the vacated space. This is not accompanied by a rise in CSF pressure. This type of CSF space enlargement, called “hydrocephalus ex vacuo,” is not hydrocephalus in the true sense as described in this chapter. Various dementing illnesses such as Alzheimer’s disease cause a focal or predominantly focal reduction of brain volume with corresponding ventricular enlargement.
Congenital hydrocephalus: Congenital forms of hydrocephalus, like that occurring in ▶ holoprosencephaly, are addressed only peripherally in this chapter.
The different types of hydrocephalus listed above are all described in relation to the CSF, from its production to its clearance. The causes and pathophysiology of hypersecretory hydrocephalus, obstructive hydrocephalus, and malresorptive hydrocephalus are reviewed in ▶ Table 10.1. An alternative classification simply distinguishes between communicating hydrocephalus, in which CSF flow is not restricted, and obstructive hydrocephalus. Note that hypersecretory hydrocephalus and malresorptive hydrocephalus are both referred to as “communicating hydrocephalus” in this simplified classification.
Type | Cause | Site of pathophysiologic change | Type of change |
Hypersecretory hydrocephalus | Overproduction of CSF | Choroid plexus | Choroid plexus papilloma or carcinoma |
Internal cerebral veins | Venous congestion of choroid plexus with increased CSF production | ||
Obstructive hydrocephalus | Obstruction of CSF flow | Intraventricular Physiologic sites of narrowing in the ventricular system:
Extraventricular (e.g., basal CSF spaces) | Masses such as:
Adhesions:
|
Malresorptive (hyporesorptive) hydrocephalus | Decreased absorption of CSF | Over the cerebral hemispheres (including arachnoid granulations) Tentorial notch | Adhesions:
Increased venous pressure:
Precise etiology still unknown:
|
10.2.5 CSF and Intracranial Hypotension
Intracranial hypotension is characterized by a decrease in CSF pressure (usually <6 cm H2O at lumbar puncture) and postural headaches that are triggered or exacerbated by standing. This symptom is often called “hypotension headache.” In rare cases, intracranial hypotension may be accompanied by cranial nerve deficits.
10.3 Epidemiology
Very few reliable data have been published on the epidemiology of hydrocephalus. The incidence of congenital hydrocephalus is reported at 2 to 3 per 1000 live births. In the United States, 8,000 to 10,000 children are born with hydrocephalus each year.
Even fewer data have been published on adult hydrocephalus. For the United States, data from the National Center for Health Statistics indicate that approximately 150,000 patients were diagnosed with hydrocephalus in the early 1990s. This would represent a prevalence of 58:100,000 or 1:1733 population per year. It is unclear, however, whether these figures include many cases of ex-vacuo ventricular enlargement due to brain atrophy, for example.
Another approach to estimating the incidence of hydrocephalus in developed countries is to look at the number of hydrocephalus operations. For example, approximately 11,000 hydrocephalus operations were performed in Germany in 1997, based on data collected from about 80% of neurosurgical departments in the country. When we consider this figure and assume that approximately 50% of those operations were revisions, the result is 7000 “new procedures” in Germany each year. The incidence of hydrocephalus based on these figures would be approximately 10:10,000 population per year. With an assumed average survival time of 2 to 3 decades, we can calculate a hydrocephalus prevalence figure that, while higher than the figure of 58:100,000 population/year reported for the United States, is still on the same order of magnitude. A Swedish study reports an incidence of first hydrocephalus operations of 3.36:100,000 population/year. The hydrocephalus prevalence estimated from this figure is close to the figure reported for the United States.
The Swedish study also supplies data on the relative frequency of the different types of hydrocephalus that required surgery. Of 891 cases that were treated surgically within a 3-year period, 231 (26%) were posthemorrhagic, 102 (11%) were tumor-associated, and 77 (9%) were posttraumatic. Ninety-eight of the surgical patients (11%) had aqueductal stenosis. Postinfectious and postoperative hydrocephalus were each diagnosed in 13 patients (1.5%). The largest group consisted of 243 patients (27.3%) treated for idiopathic normal-pressure hydrocephalus.
A German epidemiologic study found that normal-pressure hydrocephalus had a prevalence of 0.41% (4:982 individuals) in the over-65 age group. The number of patients with normal-pressure hydrocephalus will increase considerably in the next few decades as a result of demographic trends.
10.4 Imaging
10.4.1 Modalities
10.4.1.1 Computed Tomography
CT has the ability to detect or exclude hydrocephalus. It is a faster and more cost-effective modality than MRI for the follow-up of known hydrocephalus and for assessing ventricular size after shunting.
Tips and Tricks
In the CT follow-up of children with hydrocephalus, the patient’s eyes should be protected from direct exposure to reduce the risk of radiation-induced cataracts.
10.4.1.2 Magnetic Resonance Imaging
MRI is the imaging modality of choice for disclosing the underlying cause of hydrocephalus. Owing to the significantly higher soft-tissue contrast of MRI and its capability for arbitrary plane selection, the etiology of hydrocephalus, such as lesions at strategic sites in the ventricular system (e.g., colloid cyst at the interventricular foramen or aqueductal stenosis), can be diagnosed better and more easily with MRI. It is also the preferred modality for detecting changes secondary to hydrocephalus such as “pressure caps” or increased optic nerve sheath diameter ( ▶ Fig. 10.4).
Fig. 10.4 Obstructive hydrocephalus. FLAIR sequence. This case combines several of the more definite signs for differentiating true hydrocephalus from ex-vacuo ventricular enlargement. (a) Note the bilateral enlargement of the temporal horns. (b) The occipital horns are rounded and enlarged. The frontal and occipital horns are rimmed by hyperintense “pressure caps” caused by transependymal CSF flow. (c) The outer CSF spaces (basal cisterns and sulci) are small or obliterated.
MRI also has the ability to image CSF flow. T1w and T2w sequences demonstrate signal voids in areas of rapid CSF flow, comparable to the signal void seen in arteries. CSF signal voids are particularly common at sites of physiologic narrowing in the ventricular system such as the interventricular foramina or cerebral aqueduct.
Beyond the simple detection of flow voids, the direction of CSF flow can be studied with pulse- or electrocardiographically (ECG)-triggered phase-contrast sequences. When phase-contrast sequences are acquired at different intervals from the R-wave of the ECG, they permit the dynamic visualization of CSF flow at different points in the cardiac cycle ( ▶ Fig. 10.3). Acquisitions in cine mode provide a particularly vivid record of CSF flow.
Finally, the protocol should include venous magnetic resonance angiography (MRA) in cases where a venous outflow obstruction is the presumed cause of hydrocephalus. The intracranial arteries should be imaged by time-of-flight or contrast-enhanced MRA in patients with suspected posthemorrhagic hydrocephalus (e.g., after subarachnoid hemorrhage).
▶ Table 10.2 shows a recommended MRI protocol for the etiologic investigation of hydrocephalus. Of course, the protocol can and must be modified based on the cause of the hydrocephalus.
Step | Sequence | Orientation | Slice thickness (mm) | Comments |
1 | T2w or FLAIR | Axial | 4–6 | Whole brain:
|
2 | T1w | Axial | 4–6 | Whole brain:
|
3 | T2w | Sagittal | 2–3 | Midline structures: aqueduct, tectum, interventricular foramina |
4 | T1w with contrast | Axial | 4–6 | Whole brain (see above) |
5 | T2w or T1w with contrast | Coronal or sagittal | 2–4 | Through pathologic change (e.g., tumor, interventricular foramen) |
6 (if required) | Phase-contrast sequences | Usually sagittal | Dynamic imaging of CSF flow | |
7 (if required) | Venous MRA (phase-contrast sequences) | Usually sagittal | In patients with suspected venous outflow obstruction | |
8 (if required) | Arterial MRA (time-of-flight or contrast-enhanced) | Usually axial | In patients with suspected posthemorrhagic hydrocephalus (e.g., after subarachnoid hemorrhage) |
10.4.2 Imaging Findings
The following descriptions of different forms of hydrocephalus follow the classification shown in ▶ Table 10.1:
Hypersecretory hydrocephalus, due to overproduction of CSF.
Obstructive hydrocephalus, due to restriction of CSF flow.
Malresorptive hydrocephalus, due to decreased absorption of CSF.
Congenital hydrocephalus is addressed only briefly in this chapter.
10.4.2.1 General Findings
As the CSF pressure rises and the ventricles begin to enlarge, dilatation of the temporal horns is usually the first and most sensitive sign of hydrocephalus. Apparently this is because the ventricular system has the highest compliance of the intracranial structures and is therefore the first system that shows visible volume changes in response to pressure changes.
Note
Dilatation of the temporal horns is the most sensitive radiologic sign of hydrocephalus.
Over time, all ventricular segments affected by the pressure elevation become dilated. The occipital horns of the lateral ventricles lose their sharply tapered shape, becoming rounded and enlarged ( ▶ Fig. 10.4). The increased CSF pressure cause “pressure caps” to appear around the ventricles. Usually they are most pronounced around the frontal horns of the lateral ventricles and are best demonstrated in FLAIR sequences. Pressure caps are believed to result from the transependymal seepage of CSF under pressure ( ▶ Fig. 10.4).
It is not always easy to distinguish hydrocephalus from ventricular enlargement secondary to the generalized or focal atrophy of brain tissue (hydrocephalus ex vacuo). Various signs, angles, and indices have been proposed to aid this differentiation, some more useful than others. They include the following:
Bilateral enlargement of the temporal horns: See above.
Narrowing or effacement of cortical sulci: See ▶ Fig. 10.4.
Transependymal CSF flow (“pressure caps,” see above and ▶ Fig. 10.4): The raised CSF pressure in the ventricles causes CSF to seep through the ependymal lining of the ventricles into adjacent brain tissue. This increases the local water content of the tissue, which appears hyperintense in T2w sequences and hypointense in T1w sequences. There is a potential for confusion with microangiopathic lesions in the periventricular white matter and with confluent inflammatory periventricular lesions like those seen in multiple sclerosis.
Rounding and enlargement of the normally tapered occipital horns of the lateral ventricles: See ▶ Fig. 10.4.
Enlargement of the anterior and posterior recesses of the third ventricle: The mamillopontine distance, also described as a criterion for the presence of hydrocephalus, probably reflects enlargement of the anterior recess of the third ventricle. This displaces the floor of the third ventricle down toward the pons and, with it, the mammillary bodies ( ▶ Fig. 10.5).
Accentuated CSF flow void: A sign of hyperdynamic CSF flow, especially in the third ventricle and aqueduct.
Decrease in the “ventricular angle”: This angle measures the divergence of the frontal horns ( ▶ Fig. 10.6).
Increase in the frontal horn radius: See ▶ Fig. 10.6.
Ventricular index: This is defined as the ratio of the maximum distance of the lateral margin of the frontal horn from the midline to the distance of the inner table surface from the midline at the same location (see ▶ Fig. 10.6). This index is of little help in differentiating hydrocephalus from ex-vacuo ventricular enlargement, as it is increased in both cases.
Increased diameter of the optic nerve sheath.
Compression of both transverse sinuses on venous MRA.
Fig. 10.5 Enlargement of the anterior and posterior recesses of the third ventricle (arrows).
Stay updated, free articles. Join our Telegram channel

Full access? Get Clinical Tree
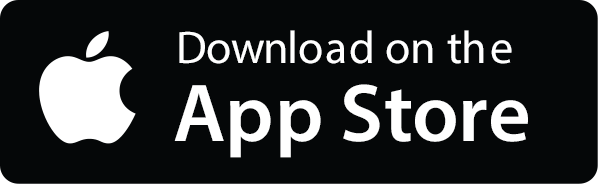
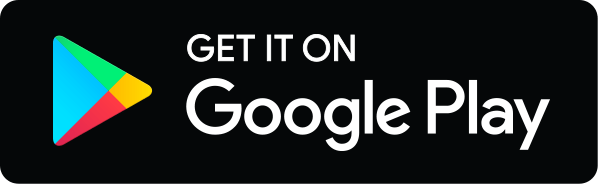