Fig. 48.1
(a) Axial CT image of a 78-year-old man shows lytic lesions of the left ilium (white arrow) and right sacrum. (b) Axial CT image shows percutaneous core needle biopsy (not shown) and fine needle aspiration (black arrow) of the lytic iliac lesions with sheath locked into the cortex (white arrow). Pathologic examination diagnosed a plasma cell myeloma
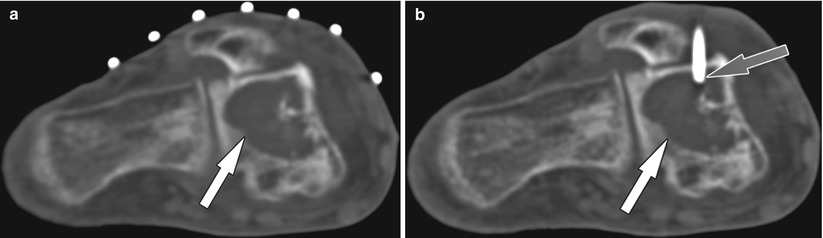
Fig. 48.2
(a) Axial CT image of a 73-year-old man shows a lytic lesion in the talus (white arrow). (b) Axial CT image shows the access sheath (gray arrow) for core needle biopsy in the lesion (white arrow). Pathologic examination diagnosed a lung cancer metastasis
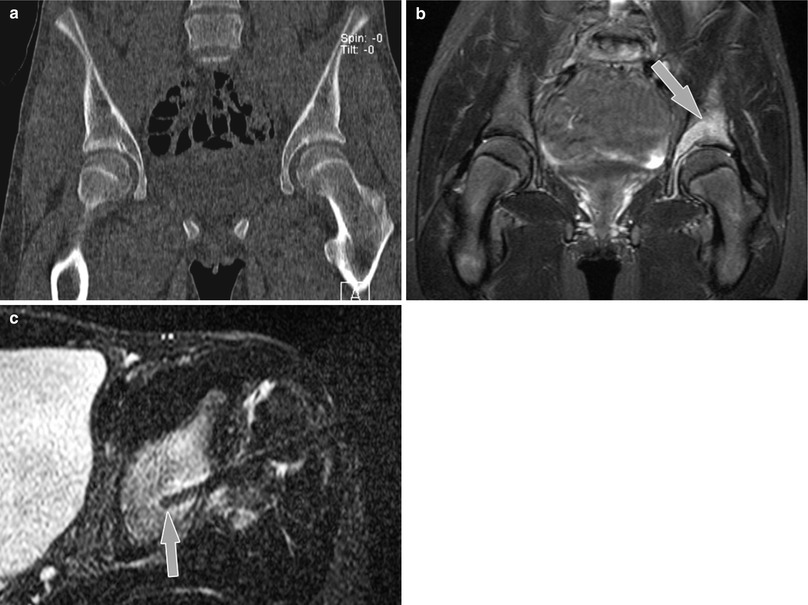
Fig. 48.3
MR-guided osseous biopsy of a lesion of the left acetabular region in a 13-year-old girl using a wide-bore 1.5 T MR system (Magnetom Espree, Siemens Medical Solutions). (a) Coronal CT image at level of the acetabulum shows no definite abnormality. (b) Diagnostic coronal 1.5 T STIR MR image (TR/TE, 7000/75; slice thickness, 4 mm; field of view [FOV], 22 cm; FOV phase, 100 %; base resolution, 256; phase resolution, 70 %; acquisition time, 6 mi 32 s) shows abnormal hyperintense signal in the left periacetabular region (arrow). (c) Interventional axial STIR MR image used for intermittent monitoring during drill placement demonstrates drill tip in the left supra-acetabular region of the signal abnormality (arrow). Pathologic examination showed diagnosed chronic recurrent multifocal osteomyelitis (With permission from Springer)
Percutaneous Vertebral Augmentation Techniques
Percutaneous vertebral augmentation techniques comprise minimally invasive, imaging-guided procedures that aim for the stabilization or restoration of the damaged vertebral body. Treatment goals of percutaneous vertebral augmentation techniques are pain relief, kyphosis reduction and prevention of progression of vertebral collapse, and decreased morbidity and mortality. Rationale for vertebral augmentation includes failure of conservative management and conditions that are inappropriate for open surgery. The general underlying principle of percutaneous vertebral augmentation techniques is the percutaneous placement of vertebral implants with or without prior manipulation of the vertebral body. An implant material that is commonly used is the polymeric bone substitute polymethylmethacrylate (PMMA). The following text is intended to provide an overview of the two most common techniques, vertebroplasty and kyphoplasty, and the more recently described technique of vertebral body stenting.
Vertebroplasty
Vertebroplasty was initially described as the image-guided, transoral puncture of the C2 vertebral body and subsequent injection of polymethylmethacrylate (PMMA) cement for the percutaneous management of a painful, aggressive hemangioma of a vertebral body [35]. While access routes and target lesions have evolved, the principle of pain relief following the injection of acrylic bone cement into the vertebral body became fundamental to vertebroplasty.
Indications for vertebroplasty are painful primary and secondary osteoporotic vertebral body compression fractures refractory to medical therapy, painful vertebral bodies with extensive osteolysis or invasion secondary to benign or malignant tumor, and painful vertebral body fracture associated with osteonecrosis [36].
Contraindications of vertebroplasty include unmanageable bleeding disorder, improvement of symptoms with conservative management, asymptomatic vertebral body fracture, local or generalized infection, allergy to bone cement, and tumor mass with involvement of the spinal canal [37].
Vertebroplasty may be performed under fluoroscopy or CT guidance [38, 39] (Fig. 48.4). Monitoring of needle placement and cement injection are critical elements of vertebroplasty in order to detect and minimize complications [40]. Reported complications rates range between 1 and 2 % for osteoporotic fractures and 5–10 % for neoplastic fractures and include injury to adjacent structures due to aberrant needle passage; cement migration into the disc space, prevertebral space, intervertebral foramen, or epidural space; cement embolization; and pyogenic infections [41].
The procedure can be performed under local anesthesia or in combination with conscious sedation. Needle access of vertebral bodies depends on the level, size, and shape of the targeted vertebral body [38, 41]. An intercostovertebral needle path is commonly used in the thoracic spine, and para- or transpedicular needle paths are commonly used in the lumbar spine. Normally, 2.5–4 ml of cement provides adequate filling of the vertebral body and pain relief in patients with osteoporotic fractures. The desired cement location is the anterior two thirds of the vertebral body [42].
Kyphoplasty
Kyphoplasty can be considered as a modification of vertebroplasty with similar indications. In addition to improvement of the structural integrity of the damaged vertebral body, morphologic restoration of osteoporotic vertebral compression fractures and kyphosis correction are additional goals [43, 44]. In kyphoplasty, device-mediated expansion of the vertebral body and creation of a cavity inside the targeted vertebral body precedes the deployment of bone cement (Fig. 48.5). The restoration of vertebral height is generally performed by a balloon tamp, which is introduced into the vertebral body through the bone needles. The inflation of the balloon is monitored under imaging control and is stopped when a pressure above 250 psi is obtained, when the balloons contact the cortical surface of the vertebral body or if the balloons expand beyond the border of the vertebral body and if the height of the vertebra is restored [37].
Although vertebral height restoration can be achieved, this effect may actually be subtle [45]. From a technical point of view, kyphoplasty is associated with reduce pressure of cement injection secondary to the created cavity and lower rates of cement leakage, which can be advantageous in cases with tumor infiltration and a compromised posterior vertebral margin [41, 46, 47]. Although, it should be noted that the increased rates of cement migration in vertebroplasty do not result in increased morbidity [41, 48]. On the other hand, balloon expansion and vertebral expansion of kyphoplasty can be unpredictable, and balloon herniation through the endplate tumor displacement can occur [41]. Unlike vertebroplasty, kyphoplasty requires general anesthesia, is associated with considerably higher cost, and requires the use of larger needles [49].
A position paper by multiple societies in Radiology and Neurosurgery states that there is no proved advantage of kyphoplasty relative to vertebroplasty with regard to pain relief, vertebral height restoration, or complication rate [50].
Vertebral Body Stenting
Vertebral body stenting was developed to improve the restoration of the height of a damaged vertebral body [51, 52]. Vertebral body stenting utilizes catheter-mounted stent, which are introduced into the vertebral body through a bone needle (Fig. 48.6). The stents surround an inflatable balloon and expand along the balloon as it is inflated. The potential advantage is the prevention of the collapse of the created cavity after deflation of the balloon by the deployed stents. The created cavity is finally with bone cement.
Controversies
Currently there is considerable amount of confusion and controversy regarding the efficacy of percutaneous vertebral augmentation therapies for the treatment of vertebral body compression fractures, when compared to medical management. There is good evidence that vertebroplasty results in superior pain control within the first 2 weeks of intervention compared with optimal medical management for osteoporotic vertebral body compression fractures [53–55], while randomized controlled trials found no benefit of percutaneous vertebroplasty over a sham placebo procedure involving the injection of local anesthetic into the area adjacent to the fracture [56, 57]. Further randomized controlled trial comparing percutaneous vertebroplasty with a sham intervention to assess pain relief in patients with an acute osteoporotic vertebral compression fracture is anticipated to better understand the benefit of percutaneous vertebroplasty [58].
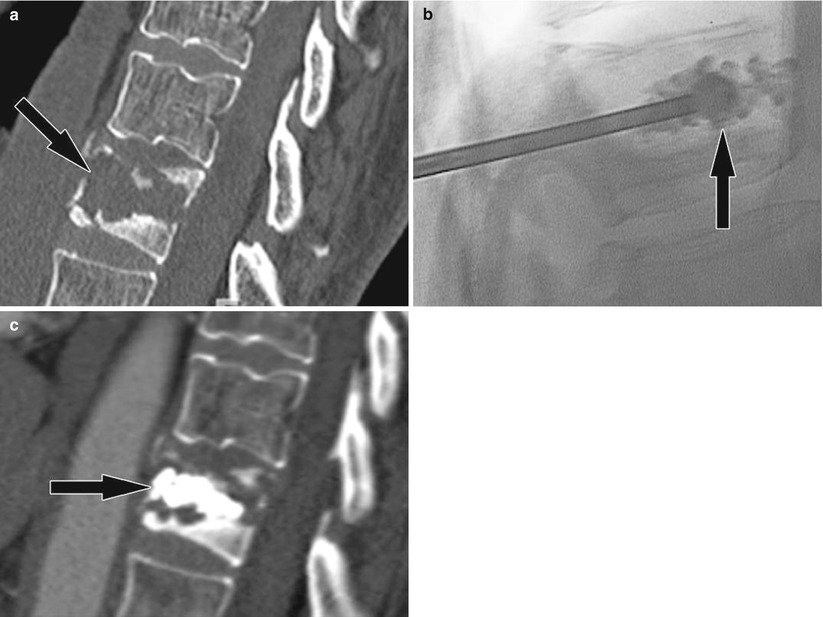
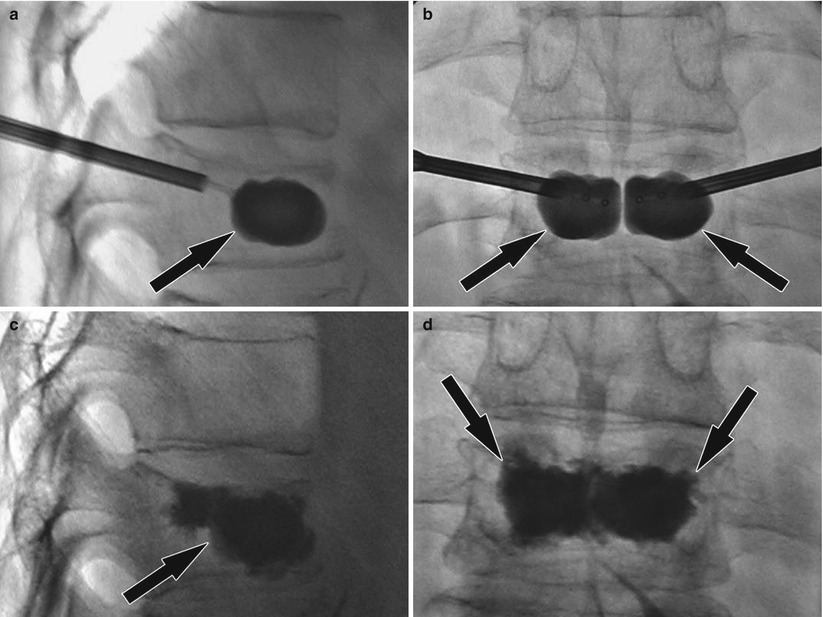
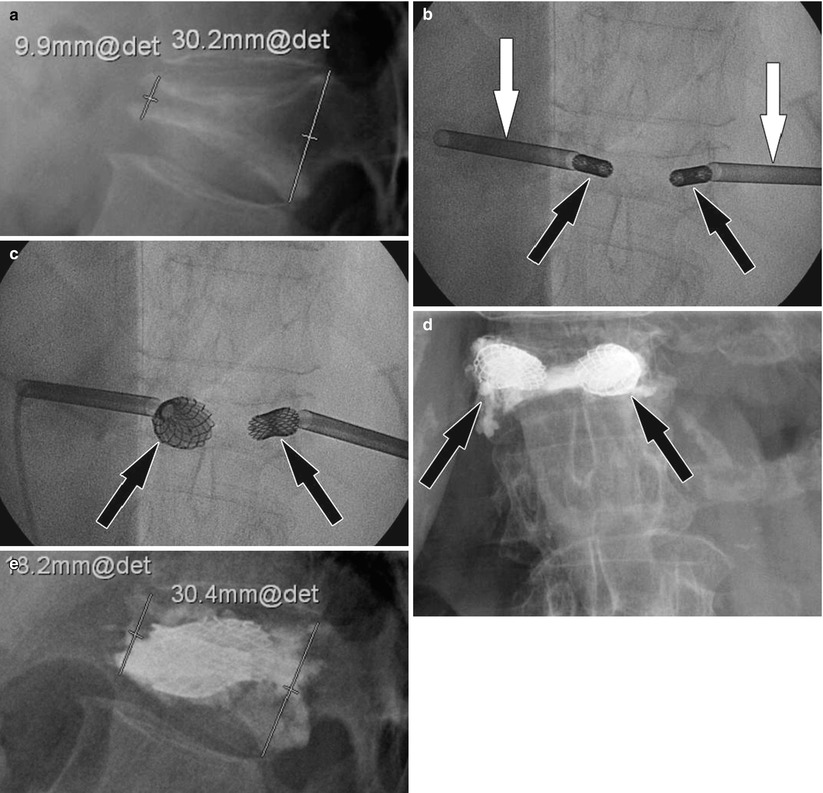
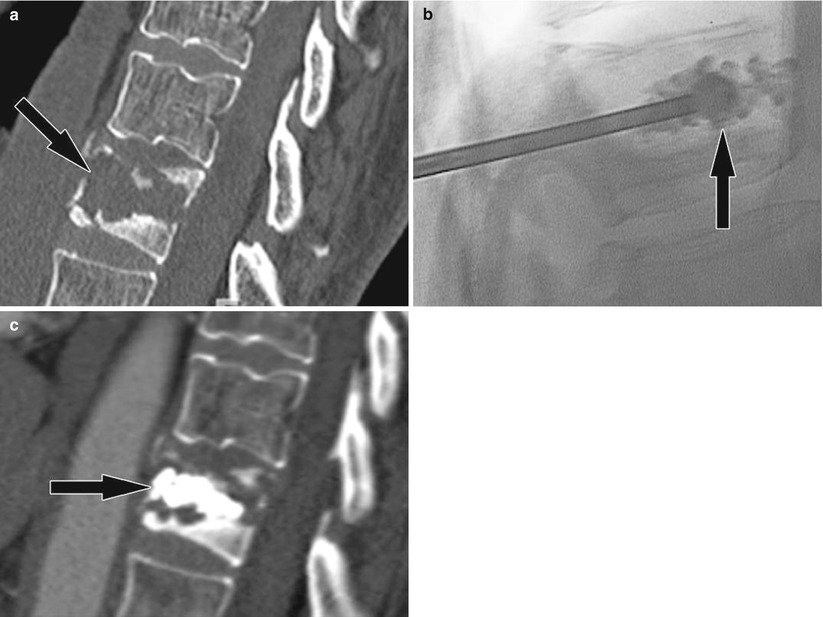
Fig. 48.4
Vertebroplasty for painful vertebral body metastasis. (a) Sagittal CT image shows a lytic vertebral body lesion (arrow) with pathologic fractures of the cortex and mild loss of height. (b) Lateral fluoroscopy image show injection of bone cement (arrow) into the lytic cavity. (c) Sagittal CT image following successful and uncomplicated vertebroplasty shows bone cement (arrow) within the lytic lesions
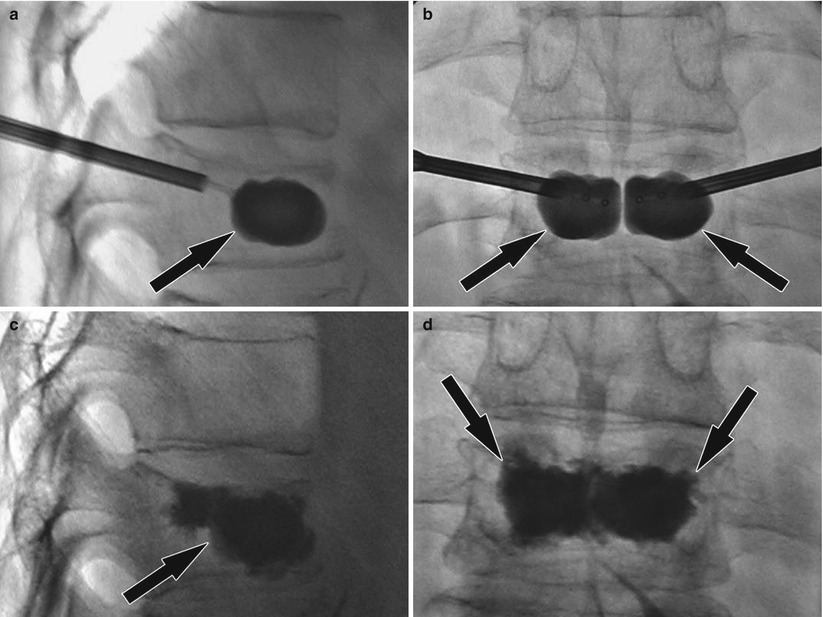
Fig. 48.5
Kyphoplasty for painful vertebral body compression fracture. (a) Lateral fluoroscopy image shows the inflated balloon (arrow) locating in the anterior two thirds of the vertebral body for height restoration. (b) Frontal fluoroscopy image shows bipedicular access and inflated balloons (arrows). (c) Lateral fluoroscopy image following injection of radiopaque bone cement (arrow) locating to the anterior two thirds of the vertebral body. (d) Frontal fluoroscopy image shows good bone cement filing (arrows) of the vertebral body
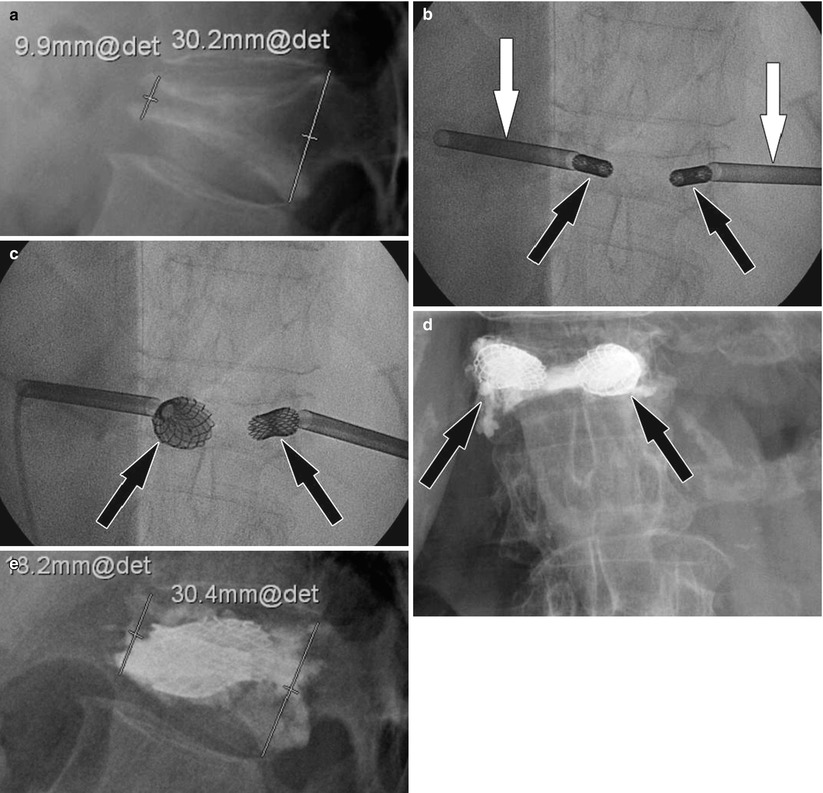
Fig. 48.6
Vertebral body stenting of an osteoporotic vertebral body compression fracture. (a) Pre-interventional lateral radiograph shows an anterior wedge compression fracture of a thoracic vertebral body with an anterior height of approximately 9.9 mm. (b) Frontal fluoroscopy image shows two bone needles (white arrows) and inserted stents in the vertebral body (black arrows). (c) Frontal fluoroscopy image shows expanded stent (arrows) after inflation of balloons and vertebral height restoration. (d) Frontal radiograph shows bone cement (arrows) surrounding the stents filling the created cavity. (e) Lateral radiograph 4 months following the procedure shows improved vertebral body height of approximately 18.2 mm
Percutaneous Management of Bone Tumors
Metastatic lesions are the most common bone tumors but seldom they are candidates for surgery due to advanced disease and comorbidities. Palliative therapy consists mainly of radiation therapy, and various percutaneous methods are available as well. Traditionally the endovascular embolization has been the most commonly used percutaneous method when applicable.
Recently, cementoplasty [59], ethanol injection [60], laser ablation (LITT) [61], cryotherapy [62], and radiofrequency ablation (RFA) [63] have become a part of a treatment paradigm towards better control and effect in palliative therapy. Microwave therapy [64], focused ultrasound (FUS) [65], and irreversible electroporation (IRE) [66] are emerging techniques.
When malignant primary bone tumors are considered, surgery is the primary treatment of choice. However, benign bone tumors are often small, and curative treatment result is possible using percutaneous techniques. The most common entity treated this way is osteoid osteoma. Palliative treatment of primary bone tumors using percutaneous techniques is also possible.
Cementoplasty refers to percutaneous injection of cement into the bone. The procedure is called also vertebroplasty or osteoplasty when performed in spine. Cementoplasty is used increasingly to treat bone metastases. The primary indication is pain control but preservation of structural integrity of bone is equally important. Polymethylmethacrylate (PMMA) is the most common cement used as it has best mechanical properties and possible direct effect on tumor tissue and nociceptors [67]. Treatment is usually mediated under fluoroscopy (also 3D fluoroscopy) or CT guidance. Careful monitoring during cement injection is mandatory in order to avoid cement leakage into the vascular structures and neural spaces. Typical targets are acetabular and sacral lesions and generally areas subject to compression forces (Fig. 48.7). Bone biopsy or vertebroplasty sets are used to gain lesion access and to inject cement. Cementoplasty can be used in combination as a stabilizing procedure before or with other therapies such as radiation therapy and radiofrequency ablation [68]. Initial results with the combination therapy of cementoplasty and RFA have been promising [69].
Ethanol injection is a simple and cost-effective method to achieve tumor necrosis [60]. The necrotizing effect is due to direct toxic effect, dehydration, and thrombosis. A thin (18–25 g) needle is advanced to the tumor, and 95 % ethanol is carefully administered according to the tumor volume but not exceeding 25 ml. Prior to alcohol injection, fluoroscopy control of contrast spread at the injection site is recommended in order to minimize the effects of extravasation. The problem with ethanol injection is the inability to monitor the ethanol spread directly under fluoroscopy; therefore, CT is the preferred method of guidance.
Laser ablation is an ablation technique frequently used to treat malignant soft tissue tumors [70]. LITT uses optical fibers to mediate light energy to tumor tissue thus ensuing coagulation necrosis. Powerful Nd:YAG or infrared lasers can be used. Along with FUS, LITT is ideally suited for MRI guidance and thermal monitoring due to lack of MRI interference. Using cooled laser catheters, larger tumors can be successfully treated. Bone access is through drill or trocar, and treatment is conducted in coaxial manner while making sure that the active part of the laser probe is freely situated in the lesion.
The advent of new-generation thin cryoprobes has increased the use of cryoablation in the treatment of malignant tumors. The cellular death occurs due to freezing of cytoplasm, and the freezing cycle is based on Joule-Thompson effect. The advantage of cryotherapy is the visualization of the ice ball in MRI or in CT that makes the local therapy control straightforward. The treatment is also better tolerated than heat-distributing methods. The downside of cryoablation is the lengthy treatment time due to the fact that typically several freeze-thaw cycles are needed to obtain desired effect. Prolonged treatment may also initialize transient hemolysis. Initial results of the use of cryotherapy in treating bone tumors are encouraging [62, 71].
Radiofrequency ablation is the most commonly used ablation method and is widely accepted as part of clinical paradigm in treating hepatic metastases. Similarly to LITT, RFA effect is based on ensuing tissue coagulation. The mechanism here is alternating electrical current that oscillates in high frequency. Most applicators are based on monopolar technology although bipolar technique is also used [72]. In bone, the electrode insertion to tumor tissue is through trocar or drill, if the cortical bone remains intact. CT and fluoroscopy are the most common guidance methods, but also MRI with certain restrictions may be used. In skeletal metastatic disease, the pain palliation results of RFA therapy are good to excellent [73]. The problem with RFA is that the monitoring of treatment effect during ablation is often not possible, but instead, the treatment effect relies primarily to accurate probe placement and pre-procedural planning. To generalize, this is true with all methods. Radio-frequency ionization is a low-temperature bipolar method that is more local in its effect and is used to achieve tumor decompression by vaporizing tumor tissue with the touch of the probe. It is particularly well suited to be used as combination therapy with cementoplasty.
Of benign tumors, the osteoid osteoma (OO) or lesions mimicking OO are best suited for curative percutaneous therapy. The tumors are typically small, well delineated, and readily diagnosed with imaging. Both laser and RFA can be used to obtain similar results. CT/3D fluoroscopy and MRI are used to guide therapy [61, 74–76] (Fig. 48.8).
Both OO and metastatic lesions frequently involve spine. Care must be practiced when treating lesions adjacent to critical neural structures with thermal techniques. Thermal protective measures can also be utilized; these include CO2 and saline injection or infusion to insulate critical structures from the heat [77–79].
Percutaneous methods for tumor management provide a minimally invasive means to achieve tumor palliation in malignant invasion of bone. The primary intention is not the complete removal of tumor tissue but that of tumor reduction, pain management, and restoring/maintaining structural integrity. In the case of selected benign entities, such as osteoid osteoma, curative results can be obtained.
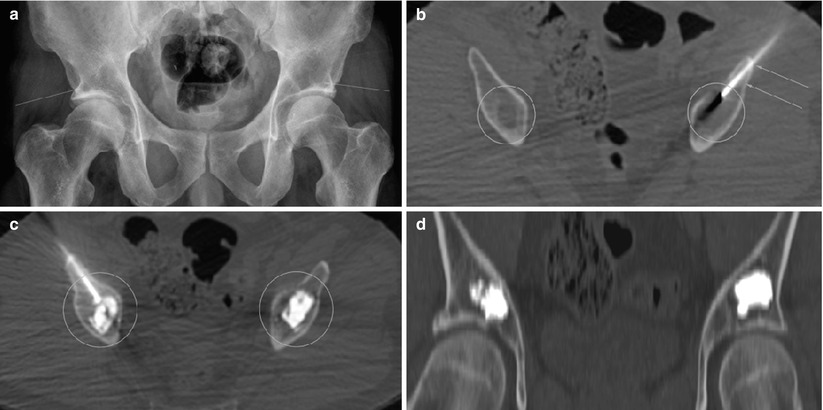
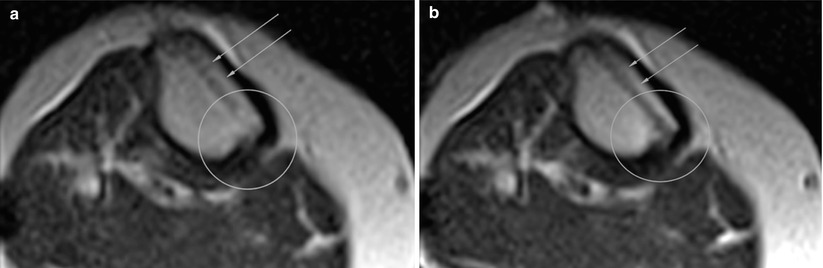
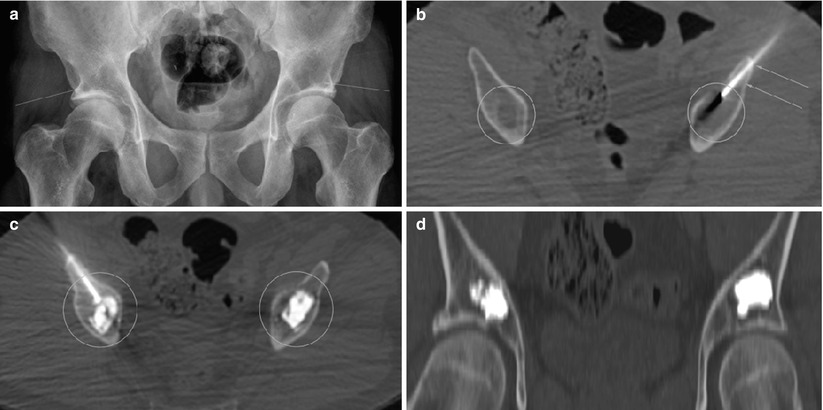
Fig. 48.7
(a) Pelvic radiograph, a patient with plasmacytoma. Bilateral acetabular lytic lesions. Arrows mark the lesions. (b) CT-guided puncture of lytic lesions (ovals). Arrows mark the needle. (c) CT-guided cement injection (ovals). (d) Coronal reconstruction image, cement in lesions
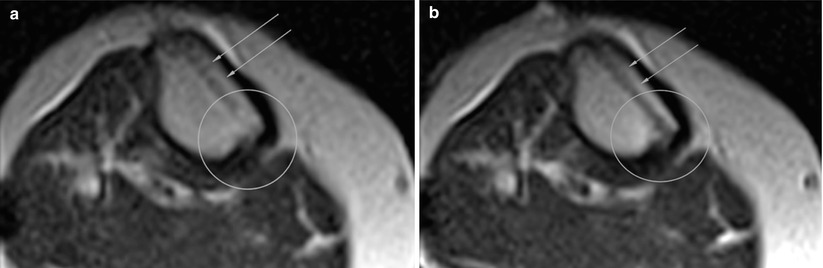
Fig. 48.8
(a) MRI-guided ablation of osteoid osteoma mimicking lesion at 1.5 T. A trans-tibial puncture with MRI-compatible needle (arrows). Lesion is seen as marked irregularity of inner cortex (oval). T2 HASTE sequence. (b) MRI-guided ablation of osteoid osteoma mimicking lesion at 1.5 T. A trans-tibial puncture with MRI-compatible needle (arrows). Thermal effect of laser light is seen as marked signal decrease. T2 HASTE sequence
Percutaneous Management and Therapy of Cystic and Reactive Bone Lesions
Cystic and reactive bone lesions are a heterogeneous entity with few common particulars but perhaps the increased osteoclastic activity resulting in bone resorption leading to cyst and cyst-like as well as other reactive changes, such as edema, in bone. These lesions include tumor-like, vascular lesions of aneurysmal bone cysts (ABC) and simple unicameral bone cysts (UBC) as well as more reactive conditions with cystic elements, such as osteochondritis dissecans (OCD), post-traumatic bone cysts, herniation pits, and osseous ganglia.
Aneurysmal bone cyst is an expanding bone lesion with vascular components [80]. It can arise as de novo lesion (70 %) or as a secondary lesion (30 %). The exact etiology is unknown, but ABC may concede with reactive vascular malformation, post-trauma, and genetic alterations ultimately thought to lead to an increase of local intraosseous venous pressure and consequent development of the lesion [81–85]. Active ABCs necessitate therapy and surgical resection, and curettage is the standard approach but not applicable to all lesions [86, 87]; other treatment methods are curettage with or without bone grafting [88] and percutaneous techniques. Radiation therapy is effective but with side effects, and steroid injections are ineffective [89, 90]. The most widely used percutaneous techniques are selective embolization, which is mainly used as temporary measure to facilitate surgery and application of direct intralesional sclerotherapy and/or demineralized bone particles [91, 92] (Fig. 48.9). Different sclerosing agents can be used, Ethibloc® is common, but use of polidocanol is reported [93], and authors have used also sodium tetradecyl sulfate (STS, Fibro-vein®); these drugs are not FDA approved for described use. The authors use dual puncture technique in order to minimize possible extravasation of sclerosing agent to surrounding tissue or venous structures. Possible complications include pulmonary embolism via venous drainage and local irritation and subcutaneous fistulae [94]. Spinal lesions should be treated with utmost care. Experience and fluoroscopy control upon sclerosant injection is mandatory. Due to this, the majority of ABC sclerotherapy is done under fluoroscopy guidance, although CT or MRI can be used for initial cyst puncture to facilitate better accuracy and selective therapy (Fig. 48.9). Results of sclerotherapy in treating ABCs are promising with high success rate [95, 96].
Simple unicameral bone cyst is a benign, usually self-limiting entity [97] mostly encountered among pediatric patients. Therapy is conservative if the cyst is not active, not symptomatic or does not pose fracture threat. In therapy, minimally invasive surgery or percutaneous techniques are preferred; invariably the therapy is performed under fluoroscopy. Surgery involves percutaneous curettage, which can be mechanical and/or chemical (alcohol, Ethibloc®, corticosteroids,), and consequent filling of the defect with bone substitutes. Combined use of mechanical and chemical curettage (sclerosing agents) and bone substitutes seems to allow best results [98]. As an access method, dual puncture technique is recommended.
Reactive conditions may cause cystic transformation of bone [99, 100]. Traumatic cysts, when present, often affect subchondral bone and may occasionally be treated with percutaneous decompression drilling and bone substitute filling (Fig. 48.10). Similarly to trauma, chronic overuse or mechanical stress may cause degenerative or reactive bone lesions. These include intraosseous ganglion cysts and herniation pits [101–104] that can be treated with percutaneous trepanation when small. Due to the inconspicuous fluoroscopy visualization of these lesions, 3D fluoroscopy, CT, and MRI are optional guidance methods for therapy.
Osteochondritis dissecans is a subchondral lesion of bone associated with microtrauma [105]. Conservative therapy is preferred, but operative treatment is often necessary to treat this condition mostly affecting pediatric patients. Percutaneous retrograde drilling presents as a minimally invasive therapy method [105–107]; with proper navigation guidance, fluoroscopy, CT, or MRI can be used as guidance modalities.
Femoral head osteonecrosis is a devastating reactive condition often leading to destruction of the joint. Femoral head decompression drilling is a procedure that alleviates symptoms and may halt the progression of the condition. Especially lesions that are of Ficat I or II stage and small are more likely to respond to decompression [108, 109]. Here several small drill channels are made to the necrotic lesion through femoral neck, using the greater trochanter as a starting point. The authors use a 3-mm drill and flexible Kirschner wires.
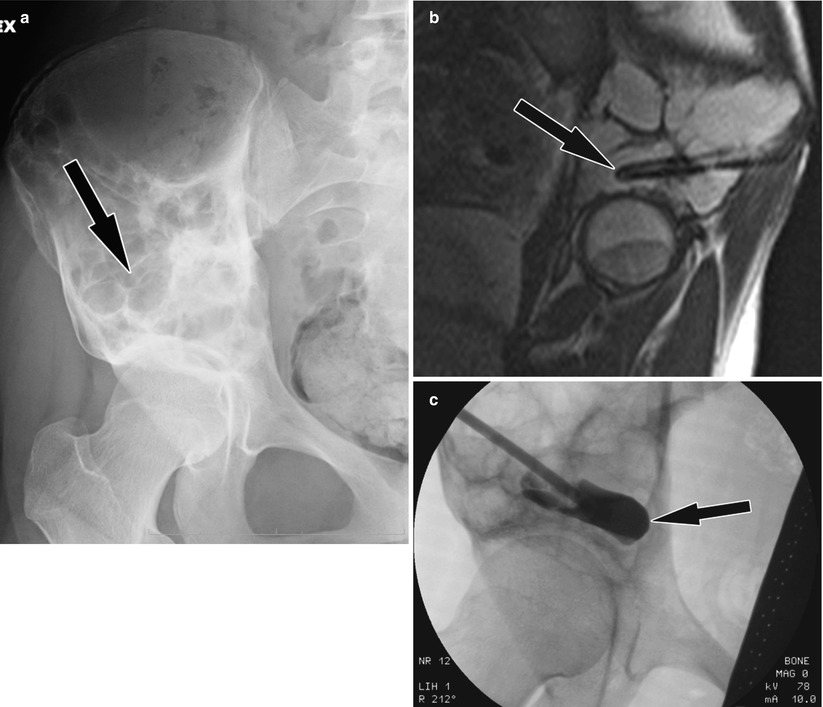
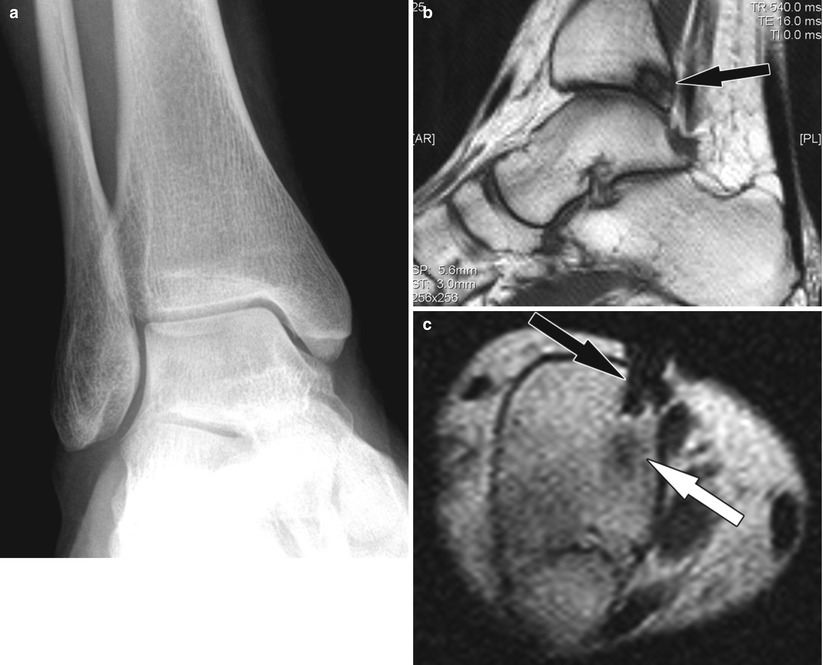
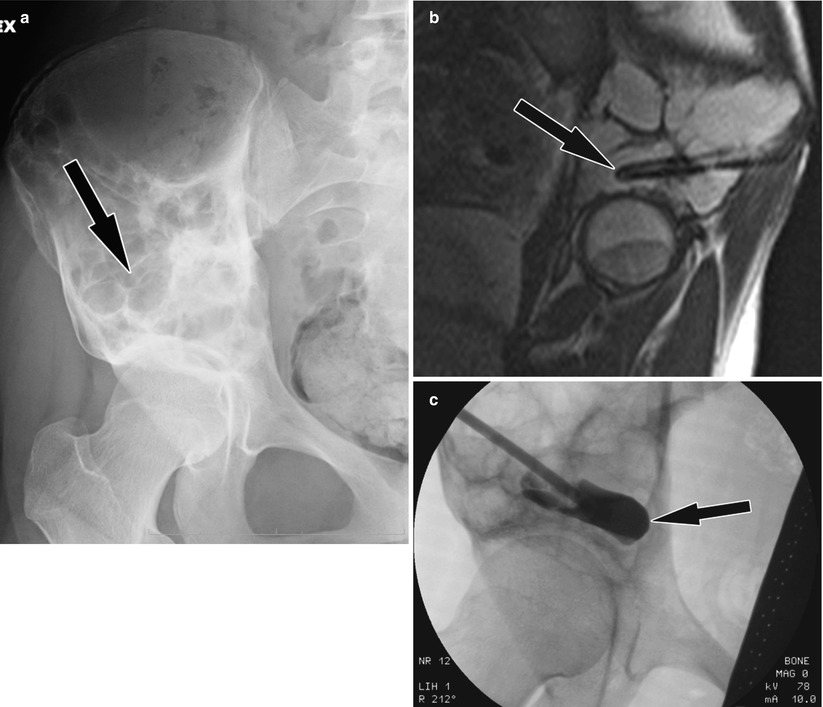
Fig. 48.9
(a) Large ABC of right hemipelvis (arrow). (b) MRI-guided ABC puncture (arrow). (c) Fluoroscopy-monitored ABC sclerotherapy (contrast injection) (arrow), after MRI-guided selective puncture
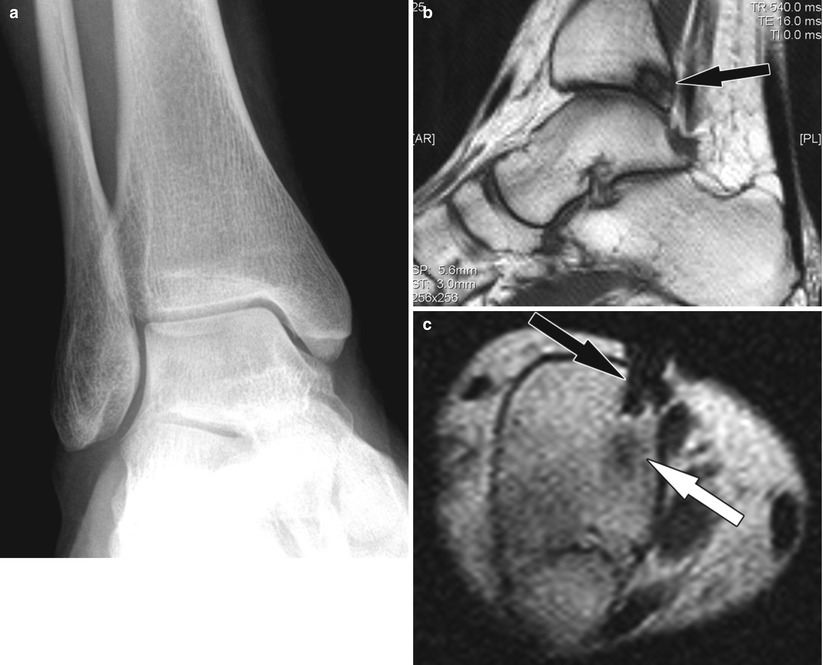
Fig. 48.10
Post-traumatic cyst, percutaneous therapy (a) Normal radiograph of the ankle (left image). (b) MRI reveals cystic lesion (arrow). (c) MRI-guided puncture (black arrow) and bone substitute injection (white arrow)
Image-Guided Reduction and Percutaneous Fixation of Fractures
Percutaneous reduction or/and fixation of fractures is a minimally invasive treatment option in a situation where there is little inherent dislocation or instability associated with trauma. Standard operative approach typically provides superior exposure, allowing access and manipulation of fractures leading to satisfactory positioning and fixation; however, with this traditional regime, there is an increased risk of complications, and some patients simply are not candidates for surgery due to poor physical condition or underlying comorbidity.
Similarly to open techniques, percutaneous fracture reduction and fixation can be achieved via instrumentation and bone cement injection or both. Cement injection is used when there is poor prognosis of healing, especially considering the usage of instrumentation, most often these cases are due to insufficiency fractures or tumors.
Image guidance is frequently used in conjunction with percutaneous fracture fixation. As a rule, a preceding helical thin slice CT and MRI, if necessary, are performed to facilitate procedural planning and possible image fusion for navigation purposes.
Fluoroscopy is by far the most commonly used for procedural guidance but CT is an option as well. The advent of new cone-beam CT devices with inbuilt navigation protocols and large field of view is probably going to widen the indications for percutaneous fixation of fractures [110]. Currently the most frequent targets for instrumented image-guided percutaneous fixation are scaphoid, calcanear, vertebral, and sacroiliac fractures.
Traumatic scaphoid fractures determined as acute B2 type can be treated conservatively with cast fixation or with percutaneous screw fixation [111–113]. Here a reduction is achieved first with an inserted Kirschner wire, and subsequent screw fixation follows. Percutaneous fixation may be associated with better overall outcome [113].
Traumatic calcanear fractures, although rare (2 % of all fractures), are common in young age groups (90 % of patients), and thus, their satisfactory treatment is economically important. Calcanear fractures have been frequently treated with percutaneous fixation, particularly those of type C2 (AO83), and there is evidence that percutaneous technique is associated with considerably less morbidity than traditional lateral approach open surgery [114, 115]; also more complicated fractures of calcaneus can be treated with percutaneous technique with initial overall results being similar to open surgery. Cement injection as a solo treatment method is infrequent but used as a method in treating calcanear joint depression type of fracture. Cement can be applied using open approach or percutaneous technique [116].
Traumatic events involving pelvic fractures are mostly of high impact of nature; this often results to significant injury, frequently extending beyond bony structures [117, 118]. Pelvic fractures consist 0.3–8 % of all fractures [119, 120]. Surgical treatment paradigm remains challenging due to technical difficulty, hemodynamic instability, and associated trauma, which may postpone pelvic surgery. Surgical treatment is performed with unstable pelvic ring fracture types B and C (AO class). Assessment of instability remains a problem, the preferred closed reduction should be performed within 2 days from initial trauma, and later attempt may fail due to ensuing fibrosis and hematoma organization. Percutaneous reduction involves clamps or handles that are attached to the fragments [121]. Open reduction is done with standard surgical technique and exposure.
Percutaneous fixation of pelvic fractures relies completely on image guidance and navigation. The operative technique does not follow standard access routes or dissection planes and due to the atraumatic nature of fixation screw placement, a most straightforward course of approach for the fixation instrumentation is chosen according to the preceding imaging (CT). With this approach, critical structures are avoided. Typically a coaxial technique is used; first, a guide wire is inserted to the intended fixation position followed with over-the-wire screws [122, 123]. External fixation of pelvic fractures is used predominantly as a temporary measure to facilitate later conclusive fixation.
Pelvic anatomy is complex and with risk of neurovascular complications associated with surgical fracture repair; navigation tools are increasingly used to minimize risk of misplacement of fixation material. Navigation can be achieved either with direct real-time (online) systems working in conjunction with imaging devices and/or data (fluoroscopy, CT, cone-beam CT [3D fluoro], MRI) or with offline systems, or both [110, 123–126]. With offline systems, there is no active image registration during the procedure thus exposing the situation to error due to faulty positional registration (external or internal patient movement or reference base movement).
In the management of pelvic fractures, a typical case involves a combination of surgical techniques due to sequential nature of treatment; percutaneous methods and image guidance are part of the established paradigm.
Traumatic thoracolumbar vertebral fractures are common, and invariably the most common fracture type is that of burst fracture (AO class A). These fractures present as potential targets for image-guided open or percutaneous image-guided fixation due to preserved posterior components of the vertebrae facilitating transpedicular introduction of fixation material. The rationale for posterior fixation is functional and procedural. Posterior fixation can make the fixation more stable and prevent the excess loss of reduction; furthermore, percutaneous procedure is minimally invasive with less traumatic consequences to paraspinal musculature, nerves, and vasculature. Most experience from percutaneous fixation is with Sextant system [127] (Fig. 48.11).
The percutaneous fixation appears to be as effective as open fixation with minor inefficiency in restoring anterior height of the vertebra [114, 127]; this could be compensated with simultaneous kyphoplasty or vertebral stenting [52]. Similarly to pelvic region, image guidance and navigation are in the key role in performing vertebral fracture fixation. There is evidence that active, real-time navigation should be preferred over static offline registration due to registration mismatch that generated inaccuracy in thoracic region [128, 129].