Imaging Gene Expression
Uwe Haberkorn
After the identification of new genes, functional information is required to investigate the role of these genes in living organisms. This can be done by analysis of gene expression, protein–protein interaction, or biodistribution of new molecules and may result in new diagnostic and therapeutic procedures. These could include visualization of and interference with gene transcription and the development of new biomolecules to be used for diagnosis and treatment. Recent progress in basic science has delivered a better understanding of the mechanisms of carcinogenesis, tumor progression, and the patients’ immune response toward the tumor. The characterization of tumor cell-specific properties allows the design of new treatment modalities as gene therapy, which circumvents resistance mechanisms toward conventional chemotherapeutic drugs. This chapter focuses on imaging cancer-related gene expression (both artificially introduced and endogenous). Although this chapter focuses on cancer, the methods described have potential applicability to a diverse range of diseases such as neurodegenerative, cardiac, and genetically linked metabolic diseases.
Currently, four approaches are evaluated in experimental and clinical studies related to cancer:
Protection of normal tissues (as the bone marrow), which are normally targets for cytotoxic drugs. The transfer of the gene for the drug efflux pump P-glycoprotein may achieve this. The presence of this pump in bone marrow cells may regulate the intracellular concentration of chemotherapeutic drugs in bone marrow progenitor cells at a lower nontoxic level and thereby protect these cells selectively.
Improvement of the host antitumor response by increasing the antitumor activity of tumor infiltrating immune competent cells or by modifying the tumor cells to enhance their immunogeneity. This approach relies on the introduction of genes that are responsible for the production of foreign surface antigens and elicit a concomitant immune response against the foreign antigen and the otherwise unrecognized tumor antigen. This may result in the elimination of the genetically modified as well as the wild type tumor cells. The expression of cytokines in tumor-infiltrating lymphocytes or in the tumor cells leads to an enhanced intratumoral cytokine concentration, which directs and activates immune-competent cells in the tumor.
Reversion of the malignant phenotype either by suppression of oncogene expression or by introduction of normal tumor suppressor genes. The inactivation of oncoproteins may be performed by introduction of genes for intracellular antibodies (intrabodies) against these oncogenes or by the use of antisense oligonucleotides and ribozymes.
Direct killing of tumor cells by the transfer of cytotoxic or prodrug-activating genes.
Visualization of Gene Transfer
For the clinical application of gene therapy, noninvasive tools are needed to evaluate the efficiency of gene transfer. This includes the evaluation of infection efficiency as well as the verification of successful gene transfer in terms of gene transcription (Table 14.1.1). This information can be obtained by imaging methods and is useful for therapy planning, follow-up studies in treated tumors, and as an indicator of prognosis.
Assessment of Viral Vector Biodistribution
An understanding of the biodistribution of vectors carrying therapeutic genes to their targets would be helpful to develop strategies for target-specific delivery of these therapeutic agents. Schellingerhout et al. (1) used enveloped viral particles labeled with indium-111 ([111In]), allowing the viruses to be traced in vivo by scintigraphic imaging. The labeling procedure did not significantly reduce the infectivity of the herpes simplex virus as evidence by the lack of significant release of the radionuclide within 12 hours after labeling. Sequential imaging of animals after intravenous administration of the [111In]-labeled virus showed a fast accumulation in the liver and a redistribution from the blood pool to liver and spleen. The recombinant adenovirus serotype 5 knob (Ad5K) was also radiolabeled with technetium-99m (99mTc) (2) and retained specific, high-affinity binding to U293 cells, which shows that the radiolabeling process had no effect on receptor binding. In vivo dynamic
scintigraphy revealed extensive liver binding, with a measured 100% extraction efficiency. The liver uptake corresponded to the results of a biodistribution study where tissues were removed and counted.
scintigraphy revealed extensive liver binding, with a measured 100% extraction efficiency. The liver uptake corresponded to the results of a biodistribution study where tissues were removed and counted.
Table 14.1.1 Genes and Radioisotope Imaging Methods Used for Monitoring Successful Gene Transfer | ||||||||||||||||||||||||||||||||||||||||||||||||||||||||||||||||
---|---|---|---|---|---|---|---|---|---|---|---|---|---|---|---|---|---|---|---|---|---|---|---|---|---|---|---|---|---|---|---|---|---|---|---|---|---|---|---|---|---|---|---|---|---|---|---|---|---|---|---|---|---|---|---|---|---|---|---|---|---|---|---|---|
|
Suicide Gene Therapy
The transfer and expression of suicide genes into malignant tumor cells represents an attractive approach for human gene therapy. Suicide genes typically code for nonmammalian enzymes, which convert nontoxic prodrugs into highly toxic metabolites. Therefore, systemic application of the nontoxic prodrug results in the production of the active drug at the tumor site. Although a broad range of suicide principles has been described (Table 14.1.2), two suicide systems are applied in most studies: the cytosine deaminase (CD) and herpes simplex virus thymidine kinase (HSVtk).
Cytosine deaminase, which is expressed in yeasts and bacteria but not in mammalian organisms, converts the antifungal agent 5-fluorocytosine (5-FC) to the highly toxic 5-fluorouracil (5-FU). In mammalian cells no anabolic pathway is known that leads to incorporation of 5-FC into the nucleic acid fraction. Therefore, pharmacologic effects are moderate and allow the application of high therapeutic doses (3,4,5). 5-FU exerts its toxic effect by interfering with DNA and protein synthesis due to substitution of uracil by 5-FU in RNA and inhibition of thymidylate synthetase by 5-fluorodeoxy-uridine monophosphate, resulting in impaired DNA biosynthesis (6).
Nishiyama et al. (7) implanted CD-containing capsules into rat gliomas and subsequently treated the animals by systemic application of 5-FC. They observed significant amounts of 5-FU in the tumors as well as a decrease in the tumor growth rate and systemic cytotoxicity. This approach for local chemotherapy was expanded by Wallace et al. (8) for application in patients with disseminated tumor. They used monoclonal antibody (mAb)-enzyme conjugates to achieve a selective activation of 5-FC, thereby obtaining a sevenfold higher level of 5-FU in the tumor after administration of mAb-CD and 5-FC compared to the systemic application of 5-FU.
Gene therapy with HSVtk as a suicide gene has been performed in a variety of tumor models in vitro as well as in vivo (9,10,11,12,13,14,15,16). In contrast to human thymidine kinase, HSVtk is less specific and it phosphorylates other nucleoside analogues such as acyclovir and ganciclovir (GCV) to their monophosphate metabolites (17). These monophosphates are subsequently phosphorylated by cellular kinases to the di- and triphosphates. After integration of the triphosphate metabolites into DNA, chain termination occurs, followed by cell death. Encouraging results have been obtained in rat gliomas using a retroviral vector system for transfer and expression of the HSVtk gene (15,16).
Recently, in vitro and in vivo studies have further demonstrated the potency of the CD suicide system. Tumor cells that had been infected with a retrovirus carrying the cytosine deaminase gene showed a strict correlation between 5-FC sensitivity and CD enzyme activity (18,19,20). However, although not all of the tumor cells have to be infected to obtain a sufficient therapeutic response, the in vivo infection efficiency of currently used viral vectors is low, and repeated injections of the recombinant retroviruses may be necessary to reach a therapeutic level of enzyme activity in the tumor.
A prerequisite for gene therapy using a suicide system is monitoring of the suicide gene expression in the tumor for two reasons: to decide if repeated gene transductions of the tumor are necessary and to find a therapeutic window of maximum gene expression and consecutive prodrug administration (21). Since 5-FC as well as GCV can be labeled with fluorine-18 ([18F]) with sufficient in vivo stability (22,23), positron emission tomography (PET) may be applied to assess the enzyme activity in vivo (Table 14.1.1). The measurement of therapy effects on the tumor metabolism may also be useful for the prediction of therapy outcome at an early stage of the treatment. PET using tracers of tumor metabolism has been applied for the evaluation of treatment response in a variety of tumors and therapeutic regimens (24,25,26,27), indicating that these tracers deliver useful parameters for the early assessment of therapeutic efficacy.
Table 14.1.2 Suicide Genes Used for Gene Therapy of Cancer | |||||||||||||||||||||||||||||||||||||||||||||||||||||||||||||||
---|---|---|---|---|---|---|---|---|---|---|---|---|---|---|---|---|---|---|---|---|---|---|---|---|---|---|---|---|---|---|---|---|---|---|---|---|---|---|---|---|---|---|---|---|---|---|---|---|---|---|---|---|---|---|---|---|---|---|---|---|---|---|---|
|
Monitoring Gene Therapy by Measurement of Therapeutic Effects
Monitoring the tumor response to gene therapy using imaging procedures for the assessment of morphological changes has been performed with magnetic resonance imaging (MRI) techniques in rats bearing chemically induced hepatocellular carcinoma, C6 rat glioblastomas and in patients with glioblastoma (28,29,30,31,32,33,34,35,36,37,38,39,40,41,42) using replication-deficient viral vectors as well as replicating herpes simplex virus mutants (41). In these studies, marked tumor necrosis or growth retardation and regression after induction of HSVtk expression followed by GCV application were observed.
Maron et al. (30) found an initial response to GCV treatment in 90% of the animals and a complete regression in two thirds of the treated rats. Tumor recurrence could also be observed. In a more clinically relevant experimental protocol consisting of late GCV delivery to large tumor formations, the long-term survival of treated rats was improved by 60% (37). MRI demonstrated a complete regression of tumors in the surviving animals. The effects of cytosine deaminase gene transfer and 5-FC administration were evaluated in different tumor models (43,44,45,46). The 5-FC sensitivity in 9L cells increased 1,700-fold after infection with an adenoviral vector carrying the CD gene (46). These rats demonstrated a remarkable inhibition of tumor growth by MRI with 70% survival for more than 90 days. The mean tumor diffusion increased by 31% within 8 days of initiating 5-FC treatment. These effects were observed prior to tumor growth arrest and tumor regression (45).
Effects of suicide gene therapy on the tumor vascularization may occur since endothelial cells of the tumor blood vessels may integrate retroviral vectors and thus become sensitive towards ganciclovir. This hypothesis was investigated in the subcutaneous 9L gliosarcoma tumor model using measurements of tumor blood flow with Doppler color-flow and ultrasound imaging (47,48). The tumor vasculature decreased after initiation of ganciclovir therapy in the HSVtk-transduced tumors. Furthermore, early necrotic changes were associated with ultrasonographic signs of scattered intratumoral hemorrhage.
Tumor perfusion, as measured in GCV-treated HSVtk-expressing KBALB tumors after intravenous administration of 99mTc-HMPAO (hexamethylpropyleneamine oxime), increased twofold during treatment at day 2 (49). Intratumoral hypoxia was changed: the
accumulation of tritium-misonidazole decreased to 30% to 40% from day 0 until day 3 after the onset of treatment, indicating that tumor tissue had become less hypoxic.
accumulation of tritium-misonidazole decreased to 30% to 40% from day 0 until day 3 after the onset of treatment, indicating that tumor tissue had become less hypoxic.
The measurement of metabolic changes after therapeutic intervention has proven to be superior to morphological procedures for the assessment of early therapy effects. MRI quantitation of changes in intracranial 9L tumor doubling times revealed a significant variation in therapeutic response to gene therapy with HSVtk and GCV. Localized H magnetic resonance spectra of treated 9L tumors showed a dramatic increase in mobile lipids and/or lactate. These changes in intracranial tumor doubling times correlated with changes in H tumor magnetic resonance spectra (50).
Fluorodeoxyglucose (FDG) uptake has been demonstrated to be a useful and very sensitive parameter for the evaluation of glucose metabolism (25,26,51,52). Since the HSVtk/GCV system induces DNA chain termination, additional changes in thymidine incorporation into tumor cell DNA are expected to occur. This may be assessed using carbon-11 ([11C])-thymidine, which has been applied to determine DNA synthesis in vivo (53,54). In a HSVtk-expressing rat hepatoma cell line, uptake measurements using thymidine (TdR)-FDG, 3-O-methylglucose, aminoisobutyric acid (AIB), and methionine were performed in the presence of different concentrations of ganciclovir (55,56). In the HSVtk-expressing cell line an increased (up to 250%) thymidine uptake in the acid-soluble fraction and a decrease to 5.5% in the acid-insoluble fraction was found. The decrease of radioactivity in the nucleic acid fraction occurred early (4 hours) after exposure of the cells to GCV and represents DNA chain termination induced by the HSVtk-ganciclovir system.
The phenomenon of a posttherapeutic accumulation of TdR or its metabolites in the acid-soluble fraction was observed in former studies after chemotherapy (24). This effect may be explained by an increase in the activity of salvage pathway enzymes (e.g., of host thymidine kinase activity during repair of cell damage). Therefore, PET measurements with [11C]-TdR may be used to assess the effects of the HSVtk-GCV system on DNA synthesis if quantitation is based on a modeling approach. In vitro the AIB uptake decreased to 47%, while the methionine uptake in the acid-insoluble fraction decreased to 17%, which is evidence of an inhibition of protein synthesis as well as of the neutral amino acid transport.
During GCV treatment, the uptake of FDG and 3-O-methylglucose increases up to 195% after 24 hours’ incubation with GCV. A high-performance liquid chromatography (HPLC) analysis revealed a decline of the FDG-6-phosphate fraction after 48 hours’ incubation with GCV. Consequently, a normalization of FDG uptake was observed after this incubation period, whereas the 3-O-methylglucose uptake was still increased. Experiments performed with different amounts of HSVtk-expressing cells and control cells showed that these effects are dependent on the percentage of HSVtk-expressing cells (55).
Dynamic PET studies of [18F]-FDG uptake were performed in animals shortly after the onset of therapy with 100 mg GCV/kg body weight as well as after administration of sodium chloride (Fig. 14.1.1).
The arterial FDG plasma concentration was measured dynamically in an extracorporeal loop and the rate constants for FDG transport (K1, k2) and FDG phosphorylation (k3) were calculated using a three-compartment model modified for heterogeneous tissues. An uncoupling of FDG transport and phosphorylation was found with enhanced K1 and k2 values and a normal k3 value after two days of GCV treatment (57).
The arterial FDG plasma concentration was measured dynamically in an extracorporeal loop and the rate constants for FDG transport (K1, k2) and FDG phosphorylation (k3) were calculated using a three-compartment model modified for heterogeneous tissues. An uncoupling of FDG transport and phosphorylation was found with enhanced K1 and k2 values and a normal k3 value after two days of GCV treatment (57).
In clinical and experimental studies an increase of FDG uptake early after treatment of malignant tumors has been described (26,51,58,59). Cell culture experiments with rat adenocarcinoma cells under chemotherapy revealed that this effect is predominantly caused by an enhanced glucose transport (58). The underlying mechanism may be a redistribution of the glucose transport protein from intracellular pools to the plasma membrane, and this is observed in cell culture studies as a general reaction to cellular stress (60,61,62,63). Since prodrug activation by the HSVtk leads to DNA chain termination and cell damage, the same reactions may also occur with this suicide system in tumor cells under gene therapy. Translocation of glucose transport proteins to the plasma membrane as a first reaction to cellular stress may cause enhancement of glucose transport and represents a short-term regulatory mechanism, which acts independently of protein synthesis.
Determination of Suicide Gene Activity by the Uptake of Specific Substrates
In a rat hepatoma model (55,56,64) uptake measurements were performed up to 48 hours in a HSVtk-expressing cell line and in a control cell line bearing the empty vector using 5-iodo-2′-fluoro-2′deoxy-1-b-D-arabinofuranosyluracil (FIAU), fluorodeoxycytidine (FCdR), 5-fluoro-1-(2′-deoxy-2′-fluoro-β-D-ribofuranosyl)-uracil (FFUdR), and ganciclovir. The FCdR uptake was higher in the HSVtk-expressing cells with a maximum after 4 hours (12-fold and threefold higher in the acid-insoluble and acid-soluble fraction, respectively). After longer incubation periods, the FCdR uptake declined. HPLC analysis showed rapid and almost complete metabolization and degradation in both cell lines (56), which might be due to dehalogenation or the action of nucleosidases. The GCV, FIAU, and FFUdR uptake showed a time-dependent increase in HSVtk-expressing cells and a plateau in control cells. The HPLC analysis revealed unmetabolized GCV in control cells and a time-dependent shift of GCV to its phosphorylated metabolite in HSVtk-expressing cells (56).
The ganciclovir, FFUdR, and the FIAU uptake were highly correlated to the percentage of HSVtk-expressing cells and to the growth inhibition as measured in bystander experiments (56,64,65). Similar results were obtained with genetically modified MCF7 human mammary carcinoma cells (66). However, the rat Morris hepatoma cells revealed a much higher difference between HSVtk-expressing cells and control cells in GCV uptake than MCF7 cells (56). MCF7 cells were not as sensitive to the HSVtk/GCV system as Morris hepatoma cells. This difference in the amount of tracer accumulation and sensitivity may be explained by the slower rate of growth of MCF7 cells as compared to Morris hepatoma cells.
Inhibition/competition experiments were performed to further elucidate the transport mechanism of ganciclovir. The nucleoside transport in mammalian cells is known to be heterogeneous with two classes of nucleoside transporters: the equilibrative, facilitated diffusion systems and the concentrative, sodium-dependent systems. In these experiments competition for all concentrative nucleoside transport systems and inhibition of the ganciclovir transport by the equilibrative transport systems was observed, whereas the pyrimidine nucleobase system showed no contribution to the ganciclovir uptake (56,66).
In human erythrocytes acyclovir has been shown to be transported mainly by the purine nucleobase carrier (67). Due to a hydroxymethyl group on its side chain, ganciclovir has a stronger similarity to nucleosides, and, therefore, it may also be transported by a nucleoside transporter. Moreover, the 3′-hydroxyl moiety of nucleosides has been shown to be important for their interaction with the nucleoside transporter (68). In rat hepatoma cells as well as in human mammary carcinoma cells the GCV uptake was shown to be much lower than the thymidine uptake (56,66). In addition to the low infection efficiency of the current viral delivery systems, slow transport of the substrate and its slow conversion into the phosphorylated metabolite have limited the therapeutic success of the HSVtk/GCV system. Cotransfection with nucleoside transporters or the use of other substrates for HSVtk with higher affinities for nucleoside transport and phosphorylation by HSVtk may improve therapy outcome.
The principle of in vivo HSVtk imaging was first demonstrated by Price et al. (69) and Saito et al. (70) for the visualization of HSV encephalitis. Several groups have recently performed in vivo studies using different tracers (71,72,73,74,75,76,77,78,79,80). Gambhir et al. (71) used 8-[18F]-fluoroganciclovir (FGCV) for the imaging of adenovirus-directed hepatic expression of the HSVtk gene in living mice. There was a significant positive correlation between the percentage of injected dose of FGCV retained per gram of liver and the levels of hepatic HSVtk gene expression. Over a similar range of HSVtk expression in vivo, the percentage of injected dose retained per gram of liver was 0% to 23% for ganciclovir and 0% to 3% for FGCV.
Alauddin et al. (72,73) used of 9-(4-[18F]-fluoro-3-hydroxymethylbutyl)-guanine ([18F]-FHBG) and 9-[(3-[18F]-fluoro- 1-hydroxy-2-propoxy)-methyl]-guanine ([18F]-FHPG) for combined in vitro/in vivo studies with HT29 human colon cancer cells, transduced with a retroviral vector, and they also found a significantly higher uptake in HSVtk-expressing cells as compared to the controls. In vivo studies in tumor-bearing nude mice demonstrated that the tumor uptake of the radiotracer is three- and sixfold higher at 2 and 5 hours, respectively, in transduced cells compared with the control cells.
Others used radioiodinated nucleoside analogues such as (E)-5-(2-iodovinyl)-2′-fluoro-2′-deoxyuridine (IVFRU) and FIAU to visualize HSVtk expression (74,75,76,77,82). Autoradiography, single-photon emission computed tomograph (SPECT) and PET images after injection of iodine-131 ([131I]) or [124I]-labeled FIAU revealed highly specific localization of the tracer to areas of HSVtk gene expression in brain and mammary tumors (76,77). The amount of tracer uptake in the tumors was correlated to the in vitro ganciclovir sensitivity of the cell lines that were transplanted in these animals (76,77).
Haubner et al. (82) studied the early kinetics of [123I]-FIAU in the CMS5 fibrosarcoma model. Biodistribution studies a half hour postinjection showed tumor/blood and tumor/muscle ratios of 3.8 and 7.2 in HSVtk-expressing tumors, and 0.6 and 1.2 in wild type tumors. The tracer showed a biexponential clearance with an initial half-life of 0.6 hours followed by a half-life of 4.6 hours. The highest activity accumulation in HSVtk-expressing tumors was observed at
1 hour postinjection. Scintigraphy showed specific tracer accumulation as early as a half hour postinjection, with an increase in contrast over time, suggesting that sufficient tumor/background ratios for in vivo imaging of HSVtk expression with [123I]-FIAU are reached as early as 1 hour postinjection.
1 hour postinjection. Scintigraphy showed specific tracer accumulation as early as a half hour postinjection, with an increase in contrast over time, suggesting that sufficient tumor/background ratios for in vivo imaging of HSVtk expression with [123I]-FIAU are reached as early as 1 hour postinjection.
Similar results were reported for IVFRU by Wiebe et al. (74,75). Due to low nontarget tissue uptake, unambiguous imaging of HSVtk-expressing tumors in mice is possible with labeled IVFRU. The advantage of iodinated tracers like FIAU may be that delayed imaging is possible. Since [18F]-labeled compounds only allow imaging early after administration of the tracer, these iodinated compounds may prove to be more sensitive in vivo. However, quantification with iodine isotopes may be a problem either with [131I], a γ- and β–emitter with a high radiation dose, or with the corresponding positron emitter [124I], which shows only 23% β+ radiation with high energy particles and multiple γ rays of high energy, which leads to a high radiation dose.
To improve the detection of lower levels of expression of PET reporter genes, a mutant herpes simplex virus type 1 thymidine kinase (HSV1-sr39tk) was used as a PET reporter gene (83). After successful transfer of this mutant gene, the accumulation of the specific substrates [8-3H]-penciclovir ([8-3H]PCV), and 8-[18F]-fluoropenciclovir (FPCV) in C6 rat glioma cells was increased twofold when compared with wild type HSVtk-expressing tumor cells and led to increased imaging sensitivity.
CD was evaluated in human glioblastoma cells. A human glioblastoma cell line was stably transfected with the Escherichia coli CD gene (21) and experiments with tritium-FC were performed. Tritium-5-FU was produced in CD-expressing cells, whereas in the control cells only tritium-5-FC was detected (21). Moreover, significant amounts of 5-FU were found in the medium of cultured cells, which may account for the bystander effect observed in previous experiments. However, uptake studies revealed a moderate and nonsaturable accumulation of radioactivity in the tumor cells, suggesting that 5-FC enters the cells only via diffusion (21). Although a significant difference in 5-FC uptake was seen between CD-positive cells and controls after 48 hours’ incubation, no difference was observed after 2 hours’ incubation and a rapid efflux could be demonstrated. Therefore, 5-FC transport may be a limiting factor for this therapeutic procedure and quantitation with PET would have to rely on dynamic studies and modeling, including HPLC analysis of the plasma, rather than on nonmodeling approaches (21).
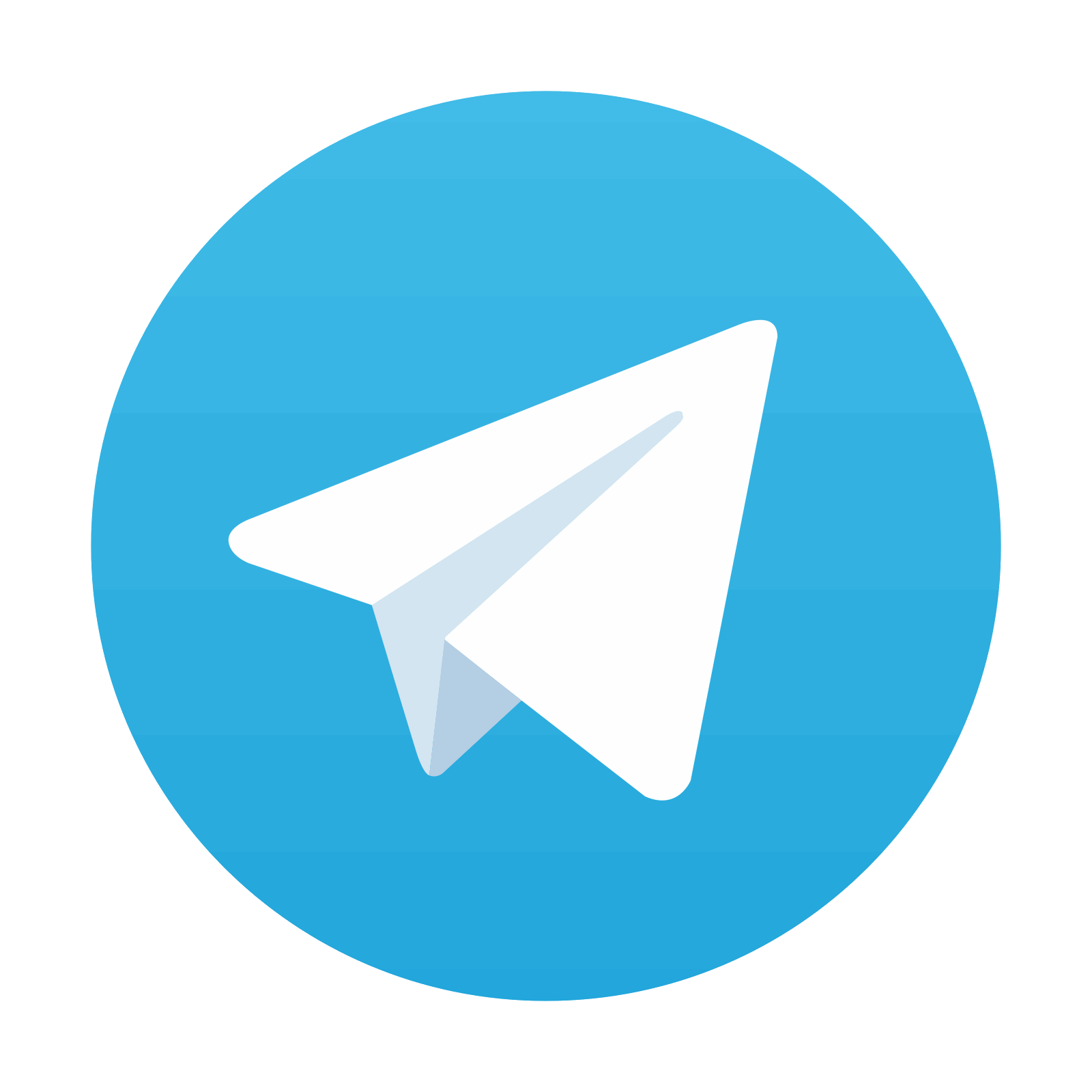
Stay updated, free articles. Join our Telegram channel

Full access? Get Clinical Tree
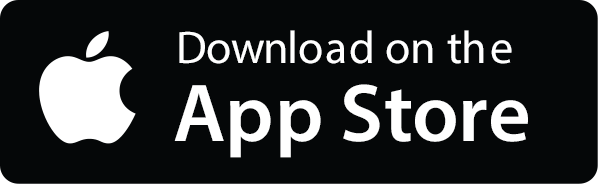
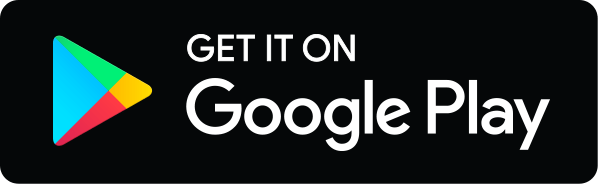