Key Facts
- •
Excessive parathyroid hormone causes increased osteoclastic activity, which leads to bone resorption and replacement with fibrous tissue.
- •
Patients with primary hyperparathyroidism are usually asymptomatic but have laboratory evidence of hypercalcemia and excessive parathyroid hormone.
- •
Tc-99m sestamibi SPECT scan and magnetic resonance imaging of the neck are highly reliable in identifying the cause of hyperparathyroidism but are most often used when a patient has had prior neck surgery.
- •
When minimally invasive parathyroid surgery is to be performed, preoperative tumor localization is indicated.
- •
When a patient younger than 35 years of age presents with hyperparathyroidism or has a known first-degree relative with MEN type I, screening with appropriate laboratory tests for evidence of pituitary gland tumors, parathyroid adenomas, pancreatic islet cell tumors, and neuroendocrine tumors is indicated.
- •
Symptoms of advanced hyperparathyroidism include multiple kidney stones, bone pain with localized swelling, anorexia, weight loss, abdominal pain, peptic ulcer disease, and mental status changes of hypercalcemia.
- •
The earliest radiographic manifestations of hyperparathyroidism are subperiosteal resorption and acro-osteolysis in the fingers.
- •
A normal hand radiograph in a patient suspected of having advanced hyperparathyroidism effectively excludes the diagnosis.
- •
Renal osteodystrophy consists of renal failure-induced rickets or osteomalacia, secondary hyperparathyroidism, and soft tissue calcification.
- •
In renal osteodystrophy, the presence of a rugger jersey spine (osteosclerosis) precludes the diagnosis of aluminum toxicity.
HYPERPARATHYROIDISM
The sine qua non of osseous disease in hyperparathyroidism is resorption of bone with or without formation of brown tumors coupled with replacement of bone by fibrous tissue. Bone resorption occurs due to increased osteoclastic activity caused by excessive parathyroid hormone. In primary hyperparathyroidism, the excessive parathyroid hormone is caused by a solitary parathyroid adenoma in 80% to 85% of cases, hyperplasia in 10% to 15% of cases, multiple adenomas in 3% to 5% of cases, and carcinoma in 1% of cases.
Primary hyperparathyroidism is usually due to a parathyroid adenoma.
Primary hyperparathyroidism occurs classically in middle-aged and elderly females. Secondary hyperparathyroidism is due to calcium imbalance from chronic renal failure resulting in excessive parathyroid hormone. Pseudohyperparathyroidism occurs due to secretion of a parathyroid hormone-like substance by neoplasms.
Imaging of hyperparathyroidism has undergone a paradigm shift in the last few decades. Before the advent of the automated laboratory chemistry panel in the 1970s and its widespread institution by the 1980s, bone imaging played a role in the diagnosis of primary hyperparathyroidism. In this earlier time period, hyperparathyroid patients presented with hypercalcemic symptoms or constitutional symptoms. If the physician suspected hyperparathyroidism, imaging studies of the bones (a radiographic skeletal survey) were ordered to confirm the diagnosis. After parathyroidectomy, patients were followed up with skeletal surveys to confirm successful treatment as evidenced by the resolution of abnormal imaging findings.
In current medical practice, the typical clinical presentation of a patient with primary hyperparathyroidism is an asymptomatic individual with laboratory evidence of hypercalcemia and excessive parathyroid hormone. Given the current asymptomatic clinical presentation of this disease, both conservative and surgical treatments can be used for hyperparathyroidism. Consensus guidelines for when to use conservative versus surgical treatment have been developed.
The role of modern imaging in primary hyperparathyroidism is in preoperative localization of the lesion (i.e., parathyroid adenoma) in patients with any prior neck surgery (failed parathyroid adenoma resection surgery or any other prior neck surgery).
Imaging studies are appropriate for patients with prior neck surgery and primary hyperparathyroidism.
The preferred imaging techniques for preoperative localization of the parathyroid lesion are both a Tc-99m sestamibi SPECT imaging study and a magnetic resonance image (MRI) of the neck. This combination of imaging modalities provides a high success rate of localization (∼95% sensitivity and ∼98% positive predictive value).
There is currently debate over whether preoperative localization of the parathyroid disease is necessary in the previously unoperated neck. Standard exploratory neck dissection (in the unoperated neck) has a higher success rate for identification of a parathyroid adenoma than imaging localization exams. If the surgeon plans to perform a standard neck dissection for parathyroid disease, then preoperative imaging localization is not recommended. However, some surgeons prefer to use minimally invasive neck surgery with a small skin incision over the site of the lesion; in these cases, preoperative imaging localization is mandatory with Tc-99m sestamibi SPECT imaging (with or without computed tomography [CT] image fusion) being the imaging exam of choice.
In patients without prior neck surgery in whom minimally invasive surgery for primary hyperparathyroidism is planned, Technetium-99m sestamibi SPECT scanning (with or without CT image fusion) is necessary for localization of the lesion. In addition, some surgeons will use ultrasound (US) imaging to mark the skin surface entry site immediately prior to the minimally invasive surgery.
Osteoarticular Imaging Features
One should still be familiar with the radiographic osteoarticular findings of hyperparathyroidism, as patients do occasionally present with advanced disease. Radiographically visible osseous resorption can occur at multiple locations such as subperiosteal, endosteal, subchondral, subligamentous, subtendinous, and trabecular ( Table 33-1 ).
The earliest occurrence of subperiosteal resorption occurs along the radial aspect of the middle phalanges of the index and middle fingers. This is seen as bone resorption under the periosteum with cortical tunneling. Grossly, this resembles a long curved erosion along the length of the phalanx with the osseous surface taking on the appearance of toothbrush bristles ( Figure 33-1 ). Acro-osteolysis is also an early finding of bone resorption due to hyperparathyroidism ( Figure 33-1 ). Other common locations for subperiosteal resorption are the ribs, lamina dura of the teeth, and the medial aspect of the long bone metaphyses. Subchondral bone resorption occurs classically at the sacroiliac joints, acromioclavicular joints, sternoclavicular joints, temporomandibular joints, symphysis pubis, and the patella ( Figure 33-2 ). Subligamentous and subtendinous resorption occurs most commonly at the femoral trochanters, ischial tuberosity, calcaneus, clavicle, and humeral tuberosities ( Figure 33-3 ).
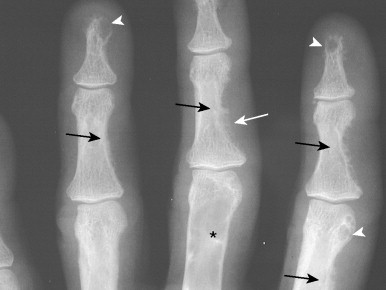
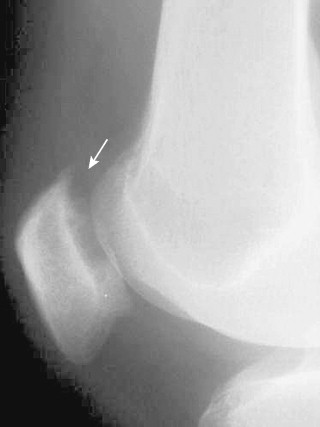
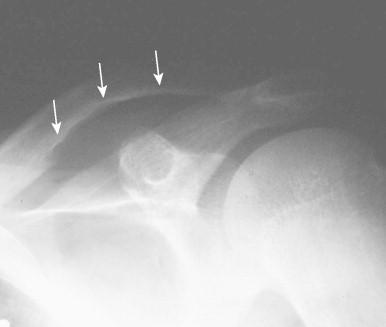
Bone resorption may be responsible for nontraumatic tendon avulsions, particularly at the patellar tendon and quadriceps tendon attachments.
Trabecular resorption is seen classically as the salt-and-pepper appearance to the skull ( Figure 33-4 ).
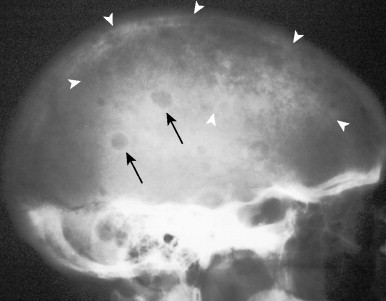
CT imaging shows the findings seen by radiography but with better detail. There are case reports that describe nonspecific increased signal on T2-weighted magnetic resonance images within the marrow of involved bones. However, MRI is not routinely used to evaluate the osseous changes in hyperparathyroidism.
Brown tumors are so named because of their brown color; this results from blood products.
Brown tumors are essentially osteoclastomas with areas of necrosis and hemorrhage. On radiography, they are geographic lytic lesions. Alone, they are nonspecific in appearance. However, when subperiosteal resorption is present, then the presence of polyostotic, geographic, lytic lesions is nearly pathognomonic for brown tumors ( Figures 33-1 , 33-4 , and 33-5 ). After resection of the offending parathyroid adenoma, brown tumors can become sclerotic as they heal. When they become sclerotic, they may mimic blastic metastases ( Figure 33-6 ). Rarely, a brown tumor can be the initial skeletal finding in hyperparathyroidism ( Figure 33-7 ). Because brown tumors usually contain hemosiderin, their classic MRI appearance is hypointense (dark) signal on both T2-weighted and T1-weighted sequences with blooming artifact on gradient echo sequences. However, if not much hemosiderin is present, brown tumors can be hyperintense on the T2-weighted images.
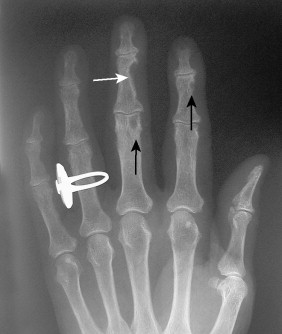
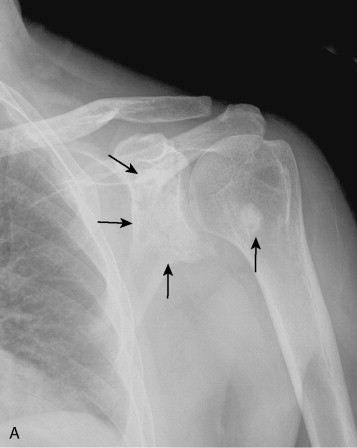
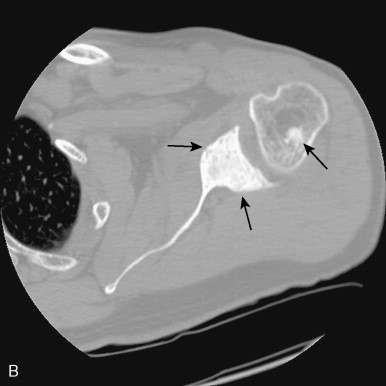
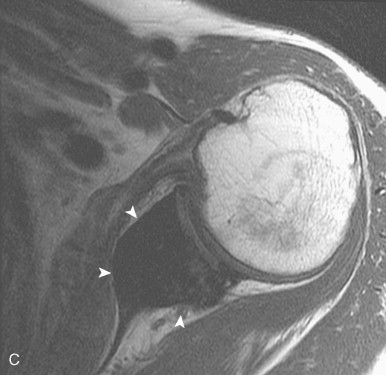
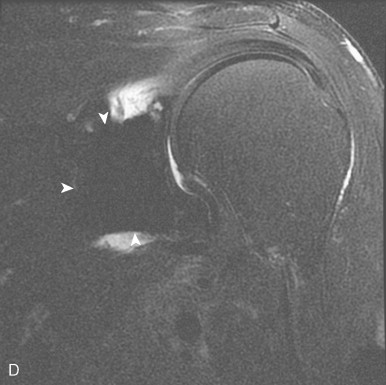
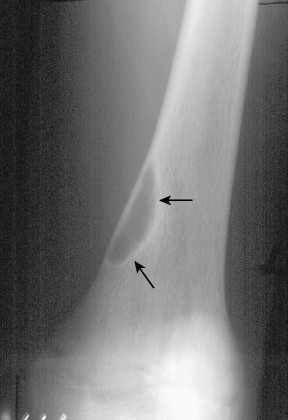
Finally, chondrocalcinosis (cartilage deposition of calcium pyrophosphate dihydrate crystals) can also be seen with hyperparathyroidism. This crystal deposition is most commonly seen on radiographs within the fibrocartilaginous menisci of the knee, the triangular fibrocartilage complex of the wrist, and the fibrocartilaginous disk of the symphysis pubis ( Figure 33-8 ).
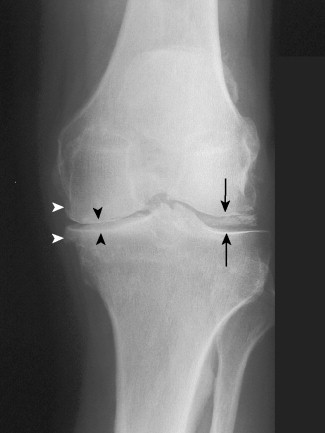
Extraarticular Imaging Findings
Extraskeletal imaging of hyperparathyroidism is confined primarily to preoperative localization of the causal parathyroid adenoma. ByUS, adenomas are classically well-defined, hypoechoic nodules separated from the thyroid gland ( Figure 33-9 ). On CT with intravenous contrast, adenomas are isodense to hypodense relative to the markedly enhancing thyroid gland and hyperdense relative to adjacent fat. The MRI appearance of adenomas is typically that of a mass that is isointense on T1-weighted images, hyperintense on T2-weighted images, and demonstrates enhancement on fat-saturated T1-weighted post-contrast (gadolinium) images. On Tc-99m sestamibi studies, there is retention of activity relative to the thyroid gland ( Figure 33-10 ).
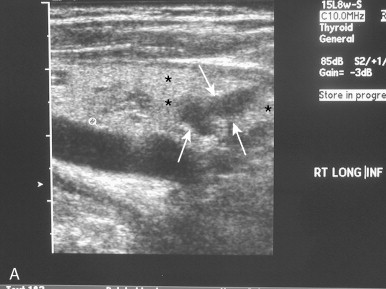
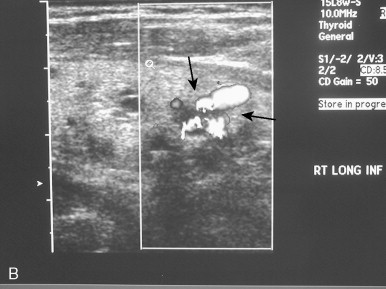
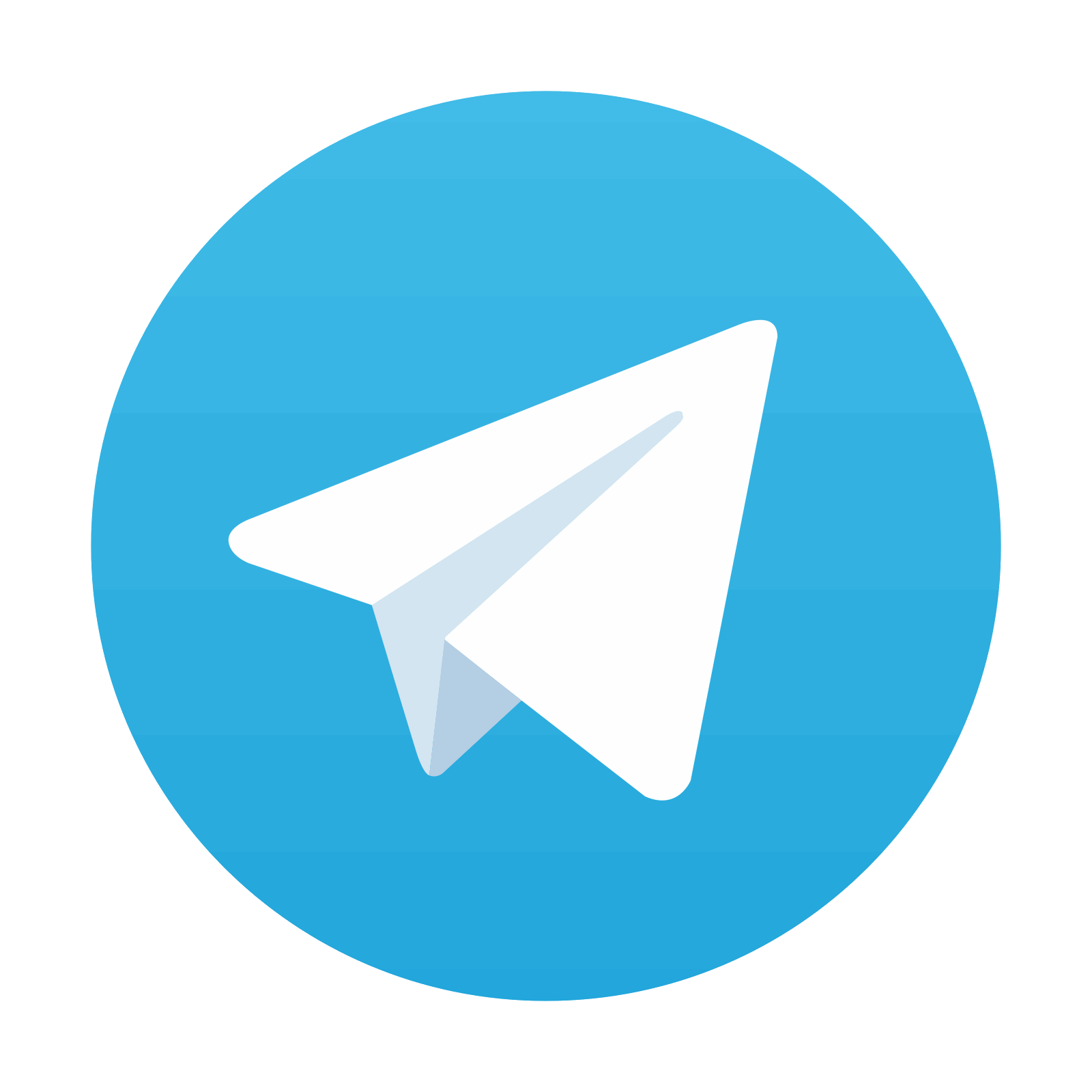
Stay updated, free articles. Join our Telegram channel

Full access? Get Clinical Tree
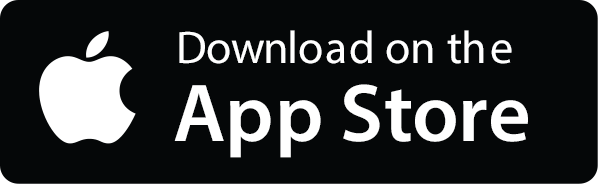
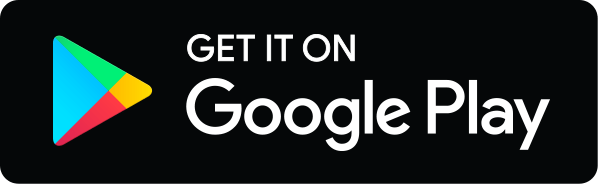
