Fig. 1
View through the microscope, the left image shows the native surgical site of a small glioblastoma. After exogenous 5-ALA application, tumor cells will become fluorescent under ultraviolet light (red tumor on the right image). This feature identifies the tumor cells intraoperatively and facilitates complete resection
On the other hand, it is widely accepted that major neurological deficits will have an adverse influence on prognosis (McGirt et al. 2009a, b; Stummer et al. 2012). The reasons for this have not been clarified. Possibly, patients with prolonged and marked postoperative neurological impairments are less likely to undergo intensive second-line therapies in the case of tumor progression; additionally, they might be prone to immobility-related complications.
Altogether, neurosurgeons are faced with conflicting goals, i.e., to achieve maximal resection during removal of diffuse neoplasms of the brain while maintaining neurological integrity, both for low- and high-grade gliomas. In this context, modern pre- and intraoperative imaging modalities are indispensable for state-of-the-art surgical planning and should be directly integrated into surgical decision making. Imaging, be it preoperative or intraoperative, has greatly helped the surgeon in achieving these opposing aims. Postoperative imaging is equally essential. Not only will it allow the surgeon to assess the quality of his work and whether his predetermined resection aims were achieved, but it will also allow for more differentiated decisions on adjuvant therapies. Residual tumor on postoperative imaging is closely related to prognosis in patients with low- and high-grade gliomas alike.
This chapter gives an overview on how imaging strategies currently assist in surgical decision making and how they can be used intraoperatively for improving surgical management of gliomas.
2 Preoperative Imaging Strategies
The decision of the surgeon on whether to perform debulking surgery, maximal cytoreduction, or only a biopsy, and the appropriate counseling of patients and families, depends on preoperative risk assessment. This assessment in turn relies on preoperative imaging, which gives information on the anatomical extent of tumor deemed appropriate for resection, on its potential dignity, and on the limits of resection, the latter being defined by functionally important brain structures, i.e., the cortex or deep white matter tracts that border on the tumor. Thus, preoperative imaging may be subdivided into imaging of surgical tumor morphology and imaging of functional brain anatomy.
2.1 What Is the Surgical Target in Low-Grade Gliomas?
LGGs are diffuse lesions which extend further than to be assumed from the usual MRI sequences. Nevertheless, for the sake of defining resectability1 before, the estimated extent of resection during, and the surgical result after an operative procedure, standard MRI sequences will have to be relied upon as the modality giving the best morphological information. Among the available sequences, fluid attenuated inversion recovery (FLAIR) is the one most often used. To this end, three large studies which are frequently cited in conjunction with the value of resection in low-grade gliomas (Smith et al. 2008; McGirt et al. 2008; De Witt Hamer et al. 2012) rely on FLAIR images to define the extent of tumor and resection (Fig. 2 ). Apart from FLAIR images, additional sequences are considered necessary (see Conventional MR imaging), especially T2-weighted images (possibly in two orientations) and T1-weighted images before and after intravenous application of Gd-containing contrast agent, the latter intended to identify areas with possible malignant transformation. Identification of such hot spots is crucial since the surgeon has to ensure that these areas are specifically included into the histopathological analysis to avoid undergrading of tumors. Hot spots may also be found in LGG presenting as gliomatosis (Fig. 3 ). It has not yet been addressed in specific studies whether focal resection and treatment of such hot spots in an otherwise unresectable diffuse LGG influence prognosis.
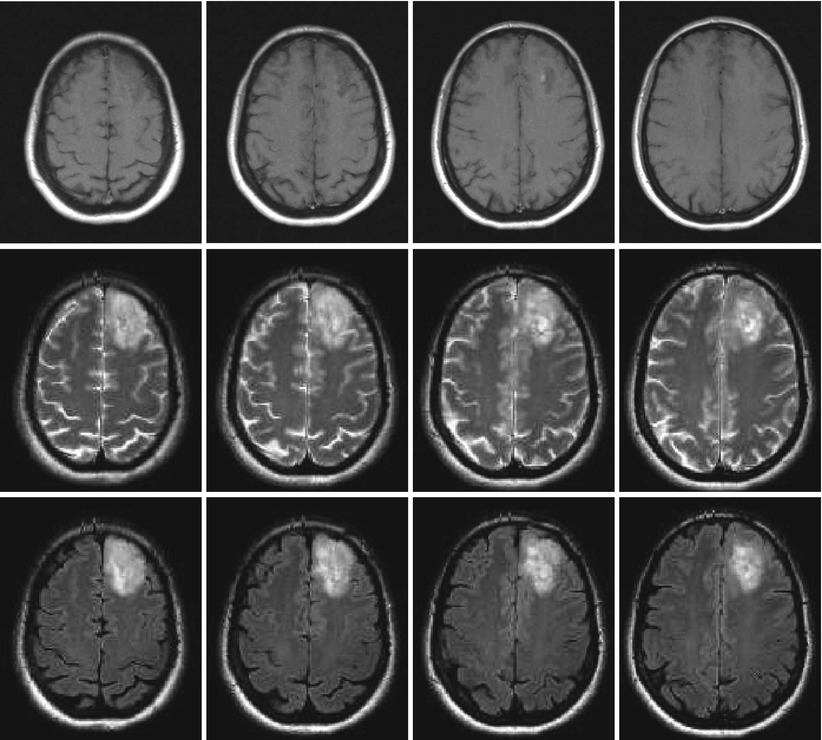
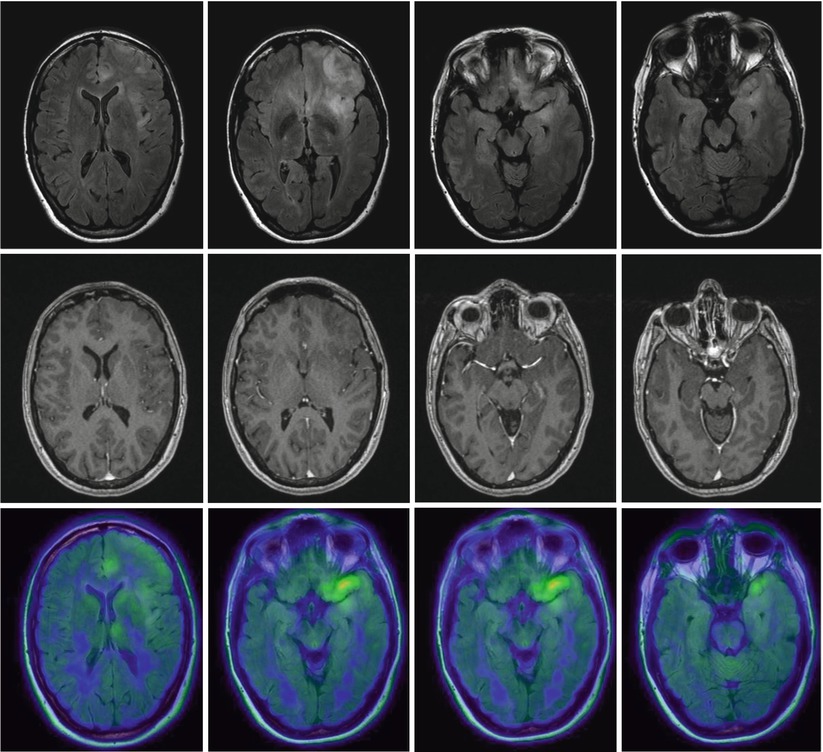
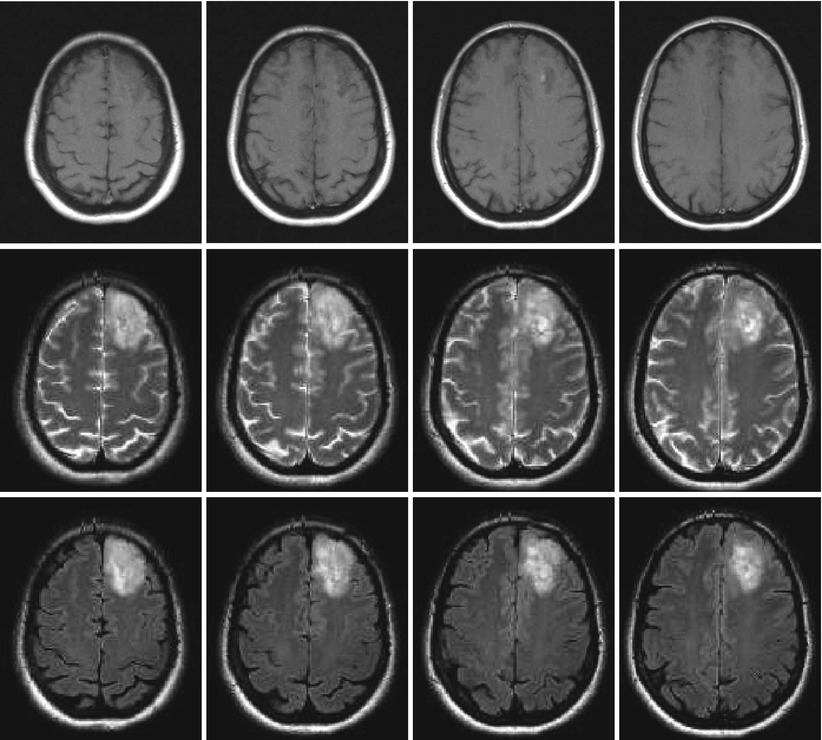
Fig. 2
Left frontal low-grade glioma in different MR sequences; female, 51 years. – native T1 (top), T2 (middle), and FLAIR (bottom) sequences (Courtesy of the Institute of Clinical Radiology, Münster)
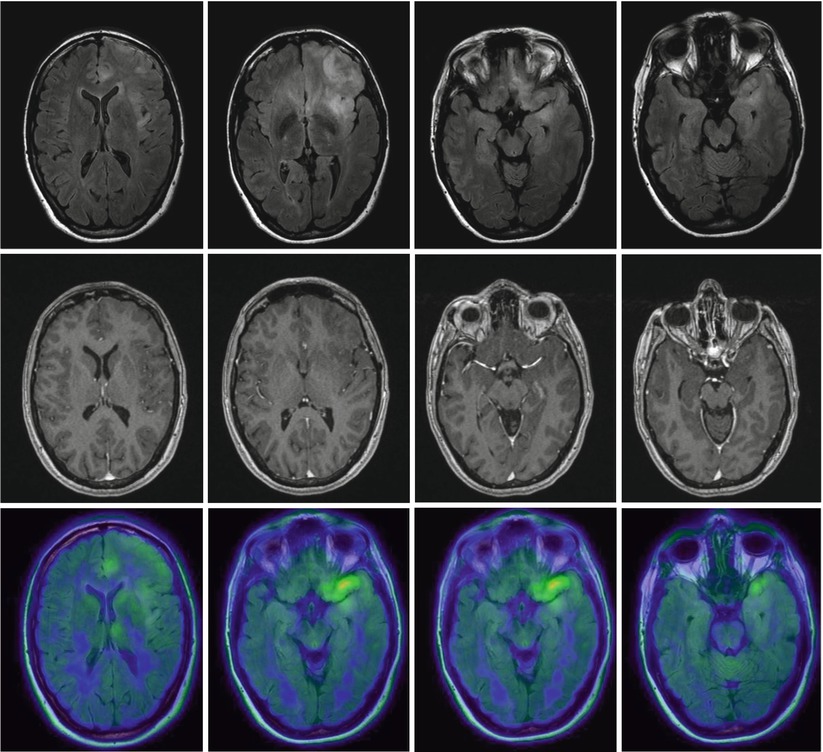
Fig. 3
Low-grade gliomatosis with metabolic hot spots demonstrated by PET; female, 45 years. – FLAIR (top), contrast-enhanced T1 (middle), and 18FET-PET (bottom) (Courtesy of the Institute of Clinical Radiology and Clinic for Nuclear Medicine, Münster)
Due to the diffuse nature of LGG with cells extending beyond what can generally be imaged by standard MRI (Sahm et al. 2012), “supratotal” resection strategies have been proposed (Duffau 2013; Yordanova et al. 2011), which consider functional rather than morphological borders of surgery. In such surgery, resection would not just be limited to the extent of the tumor as defined by the FLAIR image, but extend further up to cortex areas or deep white matter tracts which are considered functionally relevant. Such approaches are justified according to the value of maximal cytoreduction, provided they are safe. However, it remains to be established whether such surgery offers additional benefit.
2.2 The Role of Modern Imaging in Indicating Surgery in Low-Grade Gliomas
The EORTC study (Pignatti et al. 2002) has helped to define LGG patients with poor prognosis as opposed to patients with a longer survival, based on morphological criteria, histology, patient age, and neurological deficits. Higher age (>40 years), diffuse astrocytic pathology rather than oligoastrocytoma and oligodendroglioma, and neurological deficits contribute to the risk. Large tumors (>6 cm) and tumors crossing the midline were independent risk factors as well. Due to the restricted prognosis of high-risk patients, it might appear justified to treat these more aggressively, including surgical debulking or cytoreduction. Others have observed that the speed of growth, as determined from MR imaging, will also help to discern those tumors which carry a bad prognosis and possibly require a more aggressive surgical approach. Pallud et al. (2006) have demonstrated that if LGGs grow more than 8 mm per year, their prognosis is considerably worse than that of slower-growing tumors.
Amino acid positron emission tomography (PET) is a modern tool with increasing availability and acceptance for guiding the decision whether to perform surgery in apparently low-grade gliomas and in which areas to specifically collect biopsies. Hot spots in diffusely infiltrating tumors do not only delineate malignant tumor regions (Kunz et al. 2012; Ewelt et al. 2011) but they will also help to avoid undergrading if areas of malignant degeneration are missed (Fig. 3 ). It is estimated that high-grade astrocytomas are frequently undergraded if they are only biopsied instead of being resected after craniotomy, the latter with a higher likelihood of finding the anaplastic focus in up to 30 % of cases (Glantz et al. 1991; Woodworth et al. 2005; Jackson et al. 2001; Muragaki et al. 2008). Even in histologically proven LGG, increased amino acid uptake appears to signify a worse prognosis than in LGG without enhanced uptake (Floeth et al. 2007). Consequently, if a presumed LGG shows enhanced amino acid uptake, cytoreductive therapy should be considered more strongly, which can be based on the available evidence from the surgical treatment of HGG and the probability that such tumors might actually represent malignant gliomas which require adjuvant therapies.
PET (Chap. 7) and MR modalities (Chaps. 3, 4, 5, and 6) are currently being introduced into routine use, supplementing the information to be derived from standard sequences and possibly helping to identify low-grade tumors at risk for rapid progression (Guillevin et al. 2012; Geer et al. 2012; Sahin et al. 2013).
2.3 What Is the Surgical Target in High-Grade Gliomas?
In HGG a prognostic value of resection has always been associated with the removal of the contrast-enhancing parts of the tumor, as visualized by the contrast-enhanced T1-weighted sequence, although it is well known that malignant glioma cells infiltrate many centimeters beyond the contrast-enhancing tumor margins or even beyond the zone of edema (Sahm et al. 2012). This has been observed for both the anaplastic astrocytomas (Keles et al. 2006) and, with several large cohort studies and one randomized study, in glioblastoma (McGirt et al. 2009a, b; LaCroix et al. 2001; Stummer et al. 2008; Stummer et al. 2012; Kreth et al. 2013). This observation may be related to the biological characteristics of contrast-enhancing tumor. Contrast-enhancing areas are highly replete with dysfunctional vessels from uncontrolled angiogenesis, which are inefficient in supplying the tumor with oxygen, resulting in tumor hypoxia. This hypoxia, among other factors, renders the tumor resistant to radiotherapy (Stummer et al. 2011a, b).
Recent evidence, however, suggests that resections extending beyond the area of contrast enhancement might further improve prognosis in patients with glioblastomas as demonstrated by Aldave et al. (2013). This group operated glioblastoma patients guided by 5-ALA fluorescence. The zone of fluorescing tissue, which the surgeon can observe intraoperatively, is now known to extend well beyond the area of contrast enhancement as visualized by the MRI or even the 18FET-PET (Fig. 4 ) (Schucht et al. 2014; Roessler et al. 2012). Aldave et al. (2013) analyzed all their patients without contrast-enhancing residual tumor on early postoperative MRI and stratified by whether all fluorescing tissue had been resected or not. They demonstrated that patients in whom all fluorescing tissue had been removed survived significantly longer. The MRI equivalent to this region of fluorescence extending beyond contrast enhancement is not known. Whether MR spectroscopy (see Chap. 3), diffusion-weighted imaging (Chap. 5), or MR perfusion methods (Chap. 4) will play a future role in helping to predict the extension of fluorescence beyond the region of contrast enhancement cannot yet be foreseen. In a malignant glioma, contrast enhancement will typically be encased by the zone of edema (Fig. 4 ).
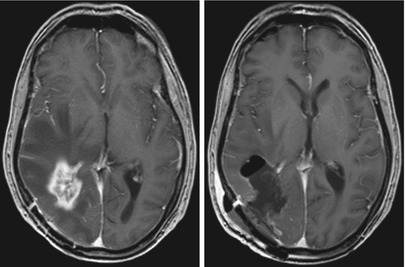
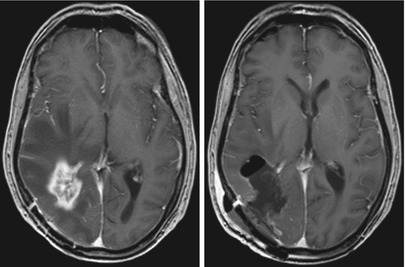
Fig. 4
Glioblastoma; male, 61 years. – contrast-enhanced T1 sequence. Left: Edema encasing the region of contrast enhancement. Right: Postoperative image after “supramarginal” resection guided by 5-ALA fluorescence (Courtesy of the Institute of Clinical Radiology, Münster)
The CT scan may also play a role in defining surgical strategies for oligodendrogliomas or oligoastrocytomas. These tumors frequently show calcifications on CT scans which are sometimes difficult to confidently diagnose on the MRI. Recognizing a possible “oligo” component on preoperative imaging will influence the surgeon’s strategy regarding cytoreduction when neurological function is at stake (Fig. 5 ). High-grade oligodendrogliomas and oligoastrocytomas have a better prognosis in the face of cytotoxic therapies than anaplastic astrocytomas (Wick et al. 2009), and thus for such patients jeopardizing neurological function for the sake of radical tumor removal need not be an option.
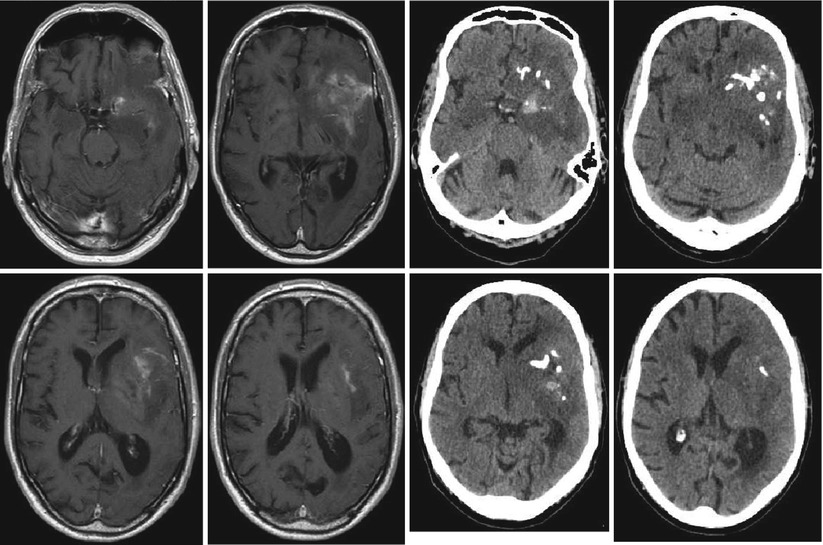
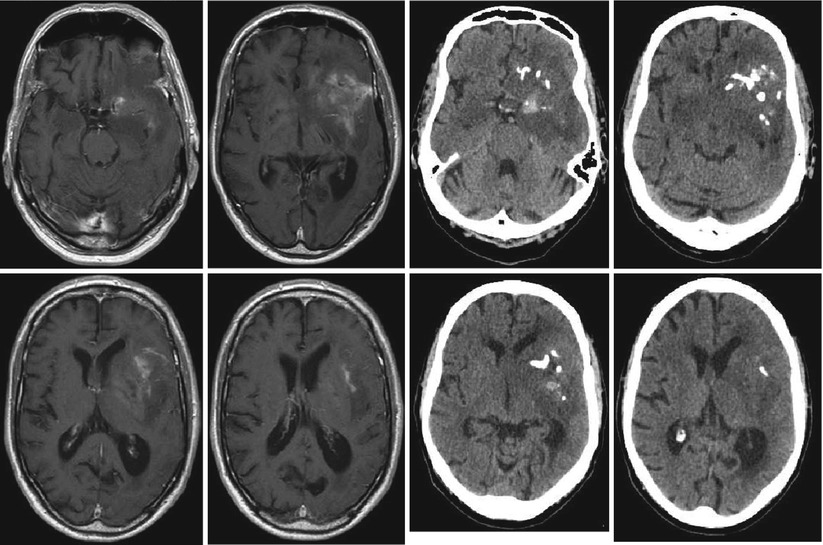
Fig. 5
Anaplastic oligoastrocytoma with calcifications; male, 72 years. – contrast-enhanced T1 (left), and native CT (right; courtesy of the Institute of Clinical Radiology, Münster)
2.4 Preoperative Imaging of Function and Functional Anatomy
During glioma surgery preservation of neurological function is paramount compared to radicalness, but safely achievable utmost radicalness must always remain the goal of surgery, or else the risks of surgery would not be justified. Intraoperatively, low-grade or infiltrating high-grade tumor tissue outside the region of gross necrosis is not easily distinguishable using microscopic visualization techniques, nor is function. Thus, preoperative knowledge of the anatomical localization of non-dispensable functions relative to tumor tissue aimed for resection is crucial. This knowledge will influence the surgeon’s judgment of resectability in the individual case and will guide his decision on the surgical approach, the selection of technological aid for surgery (neuronavigation, fluorescence, intraoperative mapping or monitoring), and his counseling of patients and families regarding the risks and gains of surgery.
2.4.1 Imaging of Functional Cortex
Intraoperatively, apart from gross anatomical landmarks for indication of functionally important cortex and tracts, the brain offers few clues to individual functional representation on the one hand, or to the extent of resectable tumor on the other (see Conventional MR imaging).
With the exception of the primary motor cortex (Farrel et al. 2007; Shinoura et al. 2009), the classical anatomical representation of functions on the cortical surface may show considerable interindividual variability, especially with regard to the areas of language representation. The so-called “Broca” area in the inferior precentral frontal gyrus and the so-called “Wernicke” region located in the posterior third of the superior temporal gyrus are more or less statistical concentrations of cortical locations relevant for speech phonology, syntax, and semantics (Vigneau et al. 2006). Cortical regions with significant language functions may be found outside the classical regions so that simply respecting anatomical topography will not give the necessary safety for resecting tumors close to the so-called eloquent brain regions (McGirt et al. 2009a, b; Ojemann et al. 2008). Such knowledge has been derived from mapping during brain surgery under local anesthesia. Furthermore, brain tumors sometimes lead to considerable distortion of normal topography, a factor in itself obscuring efforts to maintain safety during resection close to eloquent brain regions. Further, functional rearrangement over time as an indicator of brain plasticity has been demonstrated as a response to tumor invading eloquent brain (Desmurget et al. 2007), which further confounds attempts to localize individual functions based on anatomy alone. Thus, preoperative imaging of the individual representation of function would be of utmost benefit. To this end functional MRI (fMRI) is the most commonly employed modality (see Conventional MR imaging) (Belliveau et al. 1991). Appropriate computer algorithms serve to convert this information into overlays on conventional MR imagery for the representation of “eloquent” brain regions (Fig. 6 ).
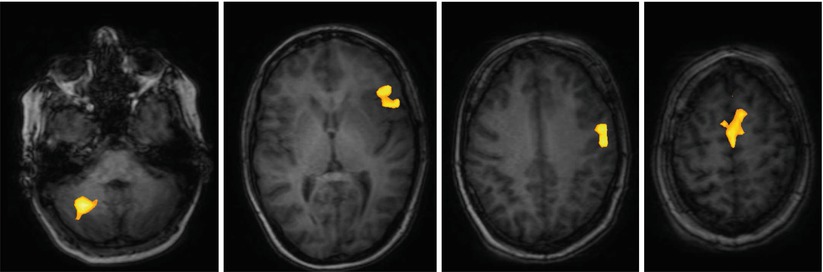
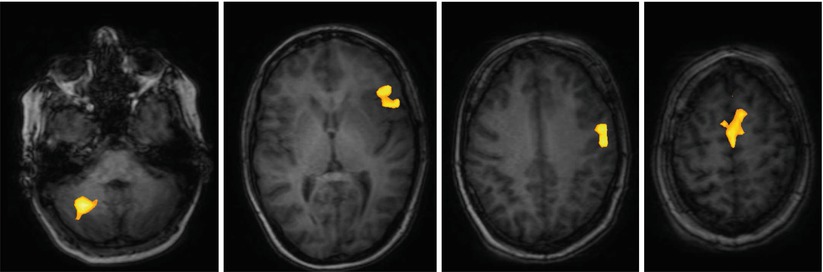
Fig. 6
Language mapping by functional MRI; female, 45 years. – BOLD data superimposed on native T1 images (Courtesy of Wolfram Schwindt, Institute of Clinical Radiology, Münster)
This information can be imported into the image base used for neuronavigation, which can then be used for locating functionally important cortex intraoperatively (Nimsky et al. 2011). However, the reliability of fMRI in precisely predicting the location of cortical locations critical for language functions is not unanimously accepted (Giussani et al. 2010). Furthermore, fMRI information suffers from brain shift, as does neuronavigation, further losing reliability during the course of surgery. Nevertheless, fMRI gives sufficient preoperative information to determine which hemisphere is dominant for language, thus allowing to determine preoperatively whether advanced neurophysiological monitoring or mapping techniques are necessary and helping to approximately predict at which locations the surgeon should test for function during surgery.
A newer development for the expansion of the potential of MRI and neuronavigation is transcranial magnetic stimulation (TMS), a technique which promises greater reliability and spatial resolution than fMRI (Weiss et al. 2012). With this method a magnetic coil is used to generate highly defined electrical fields for the transcranial induction of cortical potentials which in turn elicit motor responses. These responses can be detected, quantified, and incorporated into the MRI images used for neuronavigation, a procedure referred to as navigated brain stimulation (NBS). So far, reliable data are available for the noninvasive detection of motor functions of the hands and feet (Weiss et al. 2012). The detection of the cortical representation of perioral muscles and the muscles of the tongue and the detection of regions essential for language production (by inhibitory stimulation) are presently being validated in clinical studies.
2.4.2 Imaging of Subcortical Tracts
Although fMRI and NBS have proven useful for preoperative localization of functionally relevant cortex, these methods do not give information on subcortical white matter tracts that connect functionally relevant cortex areas and are equally important for maintenance of function. Diffusor tensor imaging, which is based on the preferential diffusion of water along fiber structures in the brain (Basser et al. 1994), has provided the technological basis for imaging of deep white matter tracts (Fig. 7). This information can be integrated into the MR imaging set used for neuronavigation, providing an instrument for localizing these tracts intraoperatively and reducing surgical risk (Wu et al. 2007). However, surgeons must bear in mind that these data rely on preoperative imaging and are distorted during the course of surgery by tissue shifts due to the loss of CSF and tumor resection (“brain shift”). Thus, this technique only gives an estimate of the true location of functional tracts (Zolal et al. 2012; Maesawa et al. 2010; Prabhu et al. 2011).
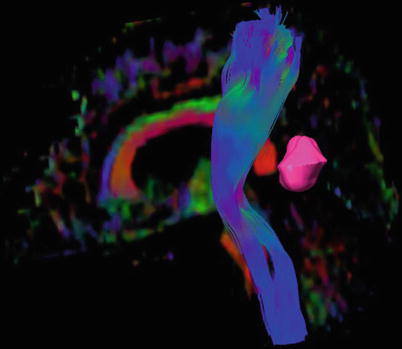
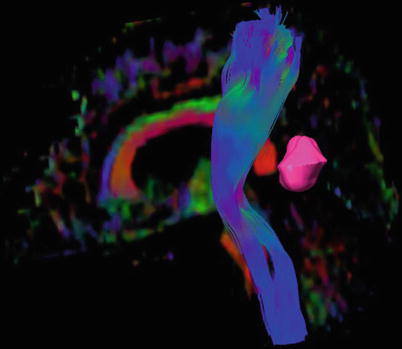
Fig. 7
3D reconstruction of the pyramid tract from a diffusion tensor data set, tumor marked lilac; male, 62 years (DTI data courtesy of the Institute of Clinical Radiology, Münster)
Recently, NBS has been combined with tractography, providing seeding points for reconstructing functionally relevant white matter tracts using DTI and carrying the potential for maps of fiber tracts for individual brain function, for instance, within the pyramidal tract (Frey et al. 2012).
3 Intraoperative Allocation of Relevant Anatomy
The main confounder involved in neuronavigation is a phenomenon called brain shift, i.e., the distortion of anatomy as a result of mass resection, puncture of cysts, or loss of CSF during surgery (Hartkens et al. 2003; Spetzger et al. 2002), compared to the image data set obtained preoperatively on which neuronavigation is usually based. This markedly reduces the accuracy of neuronavigation as surgery progresses, especially in the late stages of surgery where accuracy is crucial for identifying eloquent cortex, critical tracts, or residual tumor. For this reason intraoperative MRI or CT is frequently used where such devices are available, with the possibility of obtaining a new image data set for updating neuronavigation in an iterative fashion (Nabavi et al. 2001; Ferrant et al. 2002; Uhl et al. 2009). Intraoperative MRI by itself or in conjunction with navigation has been demonstrated to be a useful tool for identifying residual tumor in glioma surgery. Studies indicate efficacy of intraoperative MRI in increasing the radicality of glioma surgery (Senft et al. 2011; Kubben et al. 2011) or in the localization of relevant tracts or cortex. Navigated 3D ultrasound, which is far less expensive and logistically simpler in its use, fulfills a similar purpose (Rygh et al. 2008) but may be less beneficial for HGG due to the confounding influence of edema on ultrasound images (Solheim et al. 2010).
In principle, however, identification of eloquent brain or cortex based on imaging modalities will rely on the aptitude of these modalities to truthfully detect structures that are surgically relevant. At the end of the day, these methods provide two-dimensional indirect pictures derived from tissue biology which are susceptible to artifacts and require mental reconstruction and interpretation by the surgeon regarding the tissue he is confronted with in a three-dimensional space. Navigation as an aid for orientation in this space is helpful but can only be as good as the underlying imaging. Due to these limitations, many dedicated neuro-oncological surgeons resort to additional, direct, and biologically oriented methods to define function and tumor margins during surgery.
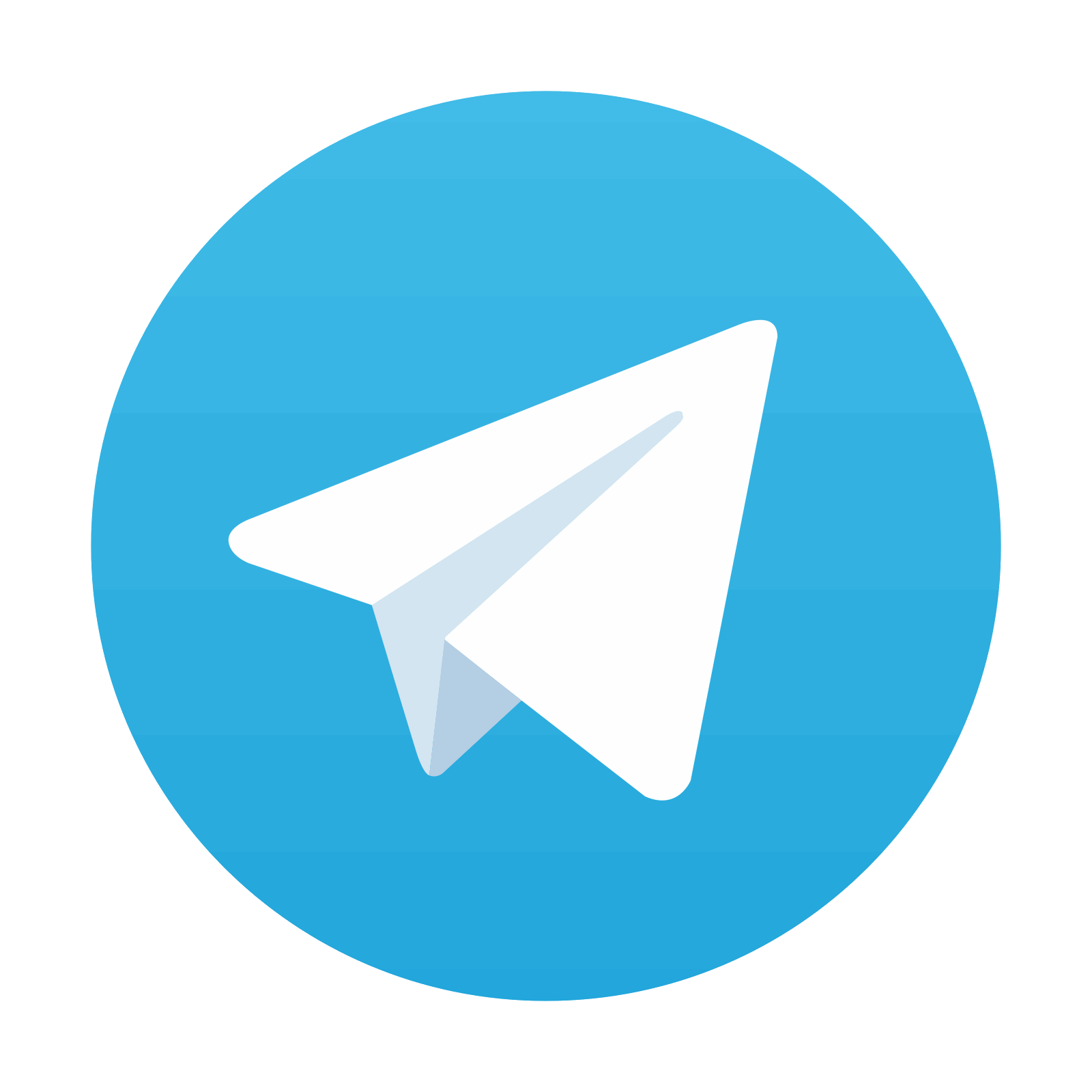
Stay updated, free articles. Join our Telegram channel

Full access? Get Clinical Tree
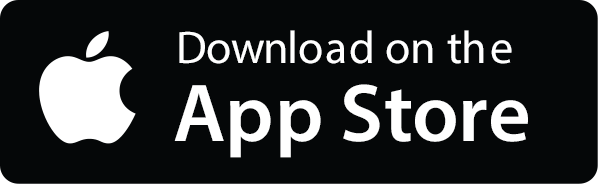
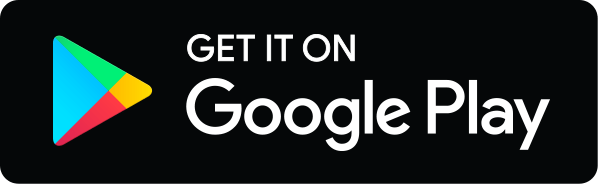