Tracer
Molecular target
18F-2-fluoro-2-deoxy-d-glucose (FDG)
Glucose metabolism
11C-methyl-l-methionine (MET)
Amino acid transport
O-(2-18F-fluoroethyl)-l-tyrosine (FET)
Amino acid transport
3,4-Dihydroxy-6-18F-fluoro-l-phenylalanine (FDOPA)
Amino acid transport
18F-3′-deoxy-3′-fluorothymidine (FLT)
Proliferation
18F-fluoromisonidazole (FMISO)
Hypoxia
18F-fluoroethyl-choline
Phospholipid synthesis
68Ga-DOTATOC
Somatostatin receptors
3 Delineation of Tumor Extent, Biopsy Guidance, and Treatment Planning
One of the most important aspects in the initial diagnosis of gliomas is the identification of tumor extension and the metabolically most active areas of the tumor. Representative tissue samples are vital for histological tumor diagnosis, prognostication, and treatment planning. The ability of contrast-enhanced MRI to show the most rapidly proliferating portions of the usually inhomogeneous gliomas is limited, particularly when the tumor does not show contrast enhancement on MRI. Multiple studies comparing the radiological findings with the histological findings in tissue samples from biopsy or open surgery have provided evidence that PET using radiolabeled amino acids detects the solid mass of gliomas and metabolically active tumor areas more reliably than either CT or MRI (Mosskin et al. 1989; Goldman et al. 1997; Kracht et al. 2004; Pauleit et al. 2005, 2009; Pirotte et al. 2007) (Fig. 1). This helps to prevent the problem of nondiagnostic biopsies from nonspecifically altered tissue and to plan invasive procedures (e.g., tumor resection, stereotactic biopsy) (Fig. 2). Local maxima of FDG uptake in heterogeneous gliomas are usually colocalized with amino acid uptake, but MET and FET PET have been shown to be considerably more sensitive than FDG PET for biopsy guidance (Pirotte et al. 2004; Pauleit et al. 2009; Plotkin et al. 2010). (Fig. 3).
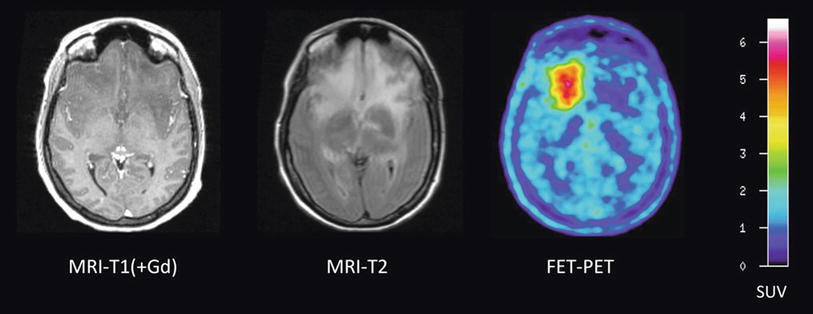
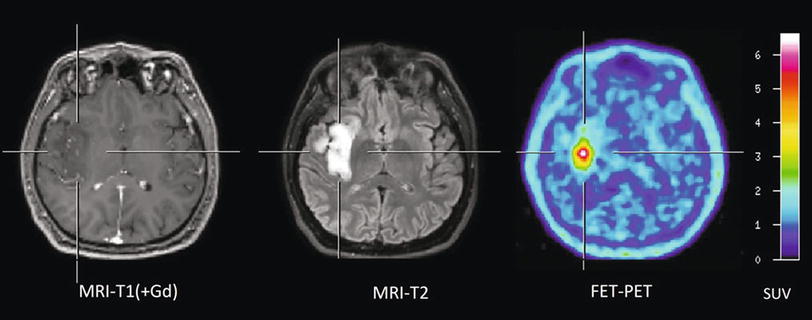
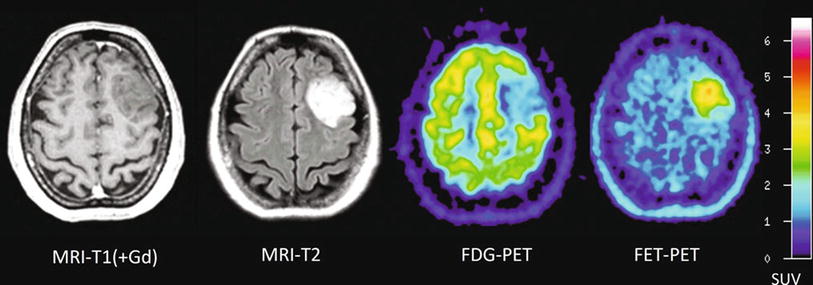
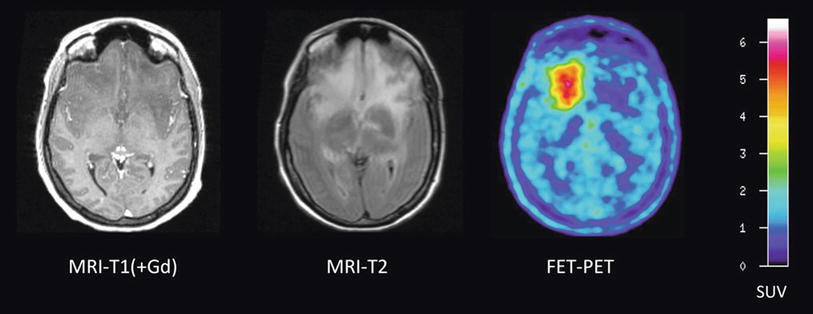
Fig. 1
Patient with an astrocytoma of WHO grade II in the frontal lobe. T1-weighted MRI after application of Gd-DTPA shows no pathological contrast enhancement and the tumor cannot be delineated. T2-weighted MRI shows widespread abnormalities in the frontal lobe and is not helpful to depict the tumor. FET PET identifies a tumor with high tracer uptake in the lower frontal lobe
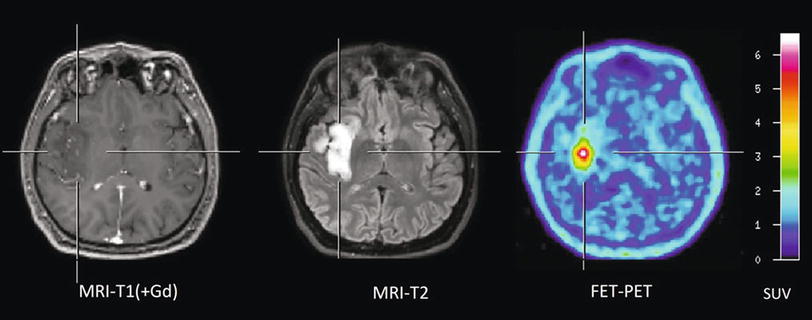
Fig. 2
Patient with a diffused astrocytoma of WHO grade II. T1-weighted MRI on the left shows no contrast enhancement and in the T2-weighted MRI (middle) diffused signal abnormalities. FET PET identifies a local maximum in the tumor for biopsy guidance
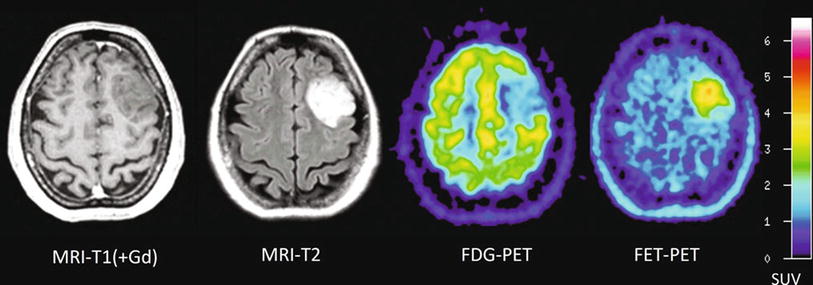
Fig. 3
Patient with a diffused astrocytoma of WHO grade II. T1-weighted MRI on the left shows no contrast enhancement in the tumor and FDG uptake is low. Again, FET PET exhibits increased tracer uptake and identifies a local maximum for biopsy guidance
Furthermore, it has been demonstrated that integrating MET PET for resection guidance of high-grade gliomas provided a final target contour different from that obtained with MRI alone in about 80 % of the procedures (Pirotte et al. 2009). Complete resection of the tumor area with increased amino acid uptake resulted in significantly longer survival of patients, while the degree of contrast enhancement on the postoperative MRI scan did not have an impact on survival. Similarly, the amount of residual tracer uptake in FET PET had a strong prognostic influence (Piroth et al. 2011a). These data indicate that resection of malignant gliomas guided by amino acid PET may increase the amount of anaplastic tissue removal and thus patients’ survival.
The improved imaging of glioma tissue using amino acid PET has also attracted interest for radiation treatment planning (Grosu and Weber 2010; Matsuo et al. 2012). A number of centers have started to integrate amino acid imaging into CT- and MRI-based radiotherapy planning, particularly when high-precision radiotherapy is performed or in the setting of dose escalation studies or for the reirradiation of recurrent tumors (Levivier et al. 2004; Grosu et al. 2005; Rickhey et al. 2008; Weber et al. 2008; Piroth et al. 2009). Improved outcome of the patients with radiotherapy planning using amino acid imaging compared with conventional radiation therapy planning, however, has not yet been proven. A recent prospective study indicated that an integrated boost intensity-modulated radiation dose escalation concept, which was based on FET PET-guided target volume delineation, showed no survival benefit for the patients (Piroth et al. 2012). The failure of that prospective study to improve survival time of the patients, however, does not mean that amino acid PET is an ineffective tool to plan radiotherapy in cerebral gliomas. Local relapses are usually detected within the 95 % dose-volume indicating that the local dose escalation is not able to improve local tumor control (Lee et al. 2009; Weber et al. 2009).
In meningiomas, promising results concerning imaging of tumor extent have been reported using the somatostatin receptor ligand 68Ga-DOTATOC (Gehler et al. 2009; Nyuyki et al. 2010; Thorwarth et al. 2011; Afshar-Oromieh et al. 2012). All studies consistently reported that 68Ga-DOTATOC PET/CT information may strongly complement anatomical data from MRI and CT in cases with complex meningioma and is thus helpful for improved target volume delineation especially for skull base manifestations and recurrent disease after surgery (Fig. 4).
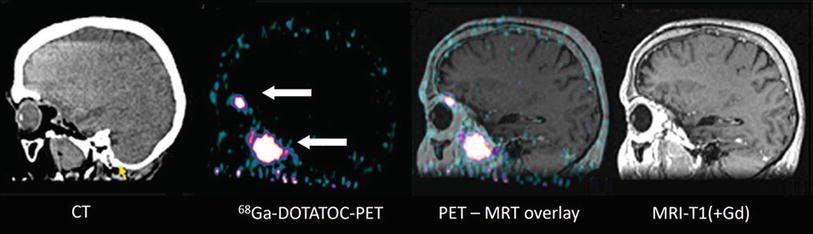
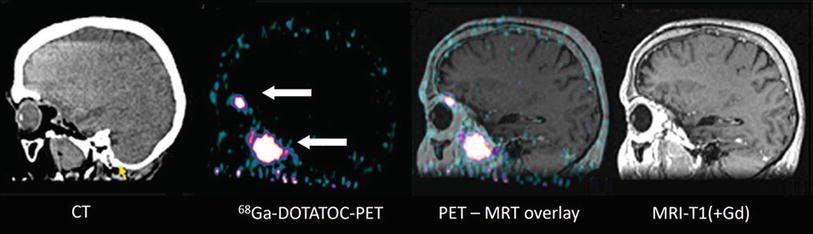
Fig. 4
Patient with a recurrent skull base meningioma 2 years after resection. Both MRI and PET/CT indicate a tumor, but 68Ga-DOTATOC PET shows an additional intraorbital lesion, which is not detectable by MRI (Courtesy of Michail Plotkin, Vivantes Clinics Berlin, Germany)
4 Tumor Grading and Prognosis
FDG PET is considered as a relative accurate predictor of the World Health Organization (WHO) grading and prognosis of cerebral gliomas since the early days of PET (Di Chiro et al. 1982; Delbeke et al. 1995; Padma et al. 2003; Chen 2007). In many studies, high-grade gliomas (HGG) could be differentiated from low-grade gliomas (LGG) (Kaschten et al. 1998; Borbely et al. 2006; Singhal et al. 2012). However, in these studies, the rate of correct identification of an HGG varies considerably. Furthermore, some studies observed no significant differences of FDG uptake in LGG and HGG (Kim et al. 2005; Miyake et al. 2012).
Some authors reported that the proliferation marker FLT may be a more powerful predictor of tumor progression and survival (Chen et al. 2005; Choi et al. 2005; Miyake et al. 2012). A relationship between in vivo derived kinetic parameters of FLT uptake and proliferation rate could be demonstrated in patients with HGG (Ullrich et al. 2008). The rate constant indicating phosphorylation k3 had a significant correlation with proliferation index Ki-67, whereas the transport rate K1 did not. No significant correlation was found between SUV of FLT and Ki-67 by immunostaining indicating that kinetic analysis was helpful for in vivo assessment of tumor proliferation in high-grade gliomas. Grade of malignancy and proliferation activity of primary brain tumors can be evaluated by FLT, but a limitation is high uptake in benign lesions that disrupt the blood-brain barrier and in necrotic areas (Saga et al. 2006; Miyake et al. 2012). Furthermore, some studies reported on a relationship between tumor grade and uptake of choline derivatives, but the number of studies is still too small to draw final conclusions (Kato et al. 2008; Gulyas and Halldin 2012).
Most studies employing amino acid PET have shown that gliomas of different WHO grades substantially overlap in their degree of amino acid uptake, so that the tumor grade cannot be reliably predicted with this technique (Pauleit et al. 2005; Ceyssens et al. 2006; Dunet et al. 2012; Rapp et al. 2013b). A more reliable grading, however, appears to be possible with FET PET, since this tracer exhibits differences in the time activity curves of tracer uptake depending on tumor grade (Weckesser et al. 2005). HGGs are characterized by an early peak around 10–15 min after injection followed by a decrease of FET uptake, while LGGs typically exhibit delayed and steadily increasing tracer uptake. Using dynamic FET PET, a differentiation of HGG and LGG has been reported in primary tumors as well as in recurrent tumors with an accuracy >80 % (Pöpperl et al. 2006b, 2007; Calcagni et al. 2011; Kunz et al. 2011; Jansen et al. 2012).
The prognostic significance of increased amino acid uptake in gliomas is controversial. Some studies seem to show that lower amino acid uptake especially in astrocytic glioma is associated with a better prognosis, but there may be high uptake in oligodendrogliomas of WHO grade II and III despite their apparently better prognosis (Kaschten et al. 1998; Pöpperl et al. 2007; Singhal et al. 2008). A further study suggests that the pretreatment volume of MET uptake but not the semiquantitative MET uptake ratio is a useful biologic prognostic marker in patients with anaplastic astrocytoma and glioblastoma (Galldiks et al. 2012a).
There appears, however, to be a consensus concerning the clinical role of amino acid imaging in prognostication for patients with LGG. Significant longer survival has been reported for patients with lower MET uptake in the tumors compared to those with higher uptake (cutoff of the tumor-to-brain ratio: 2.1) (Ribom et al. 2001; Smits et al. 2008; Smits and Baumert 2011; Arbizu et al. 2012). Furthermore, the patients only had a benefit from a surgical procedure if increased MET uptake was present (Ribom et al. 2001). Using FET PET, the combination with MR morphology has also been found to be a significant prognostic predictor for patients with newly diagnosed LGG (Floeth et al. 2007). Baseline FET uptake and a circumscribed versus a diffuse growth pattern on MRI were highly significant predictors for the patients’ clinical course and outcome. Thus, combined assessment with amino acid PET and MRI can identify subgroups of patients with a stable course in which a “watch and wait” strategy is reasonable and patients with LGG who should receive early and aggressive treatment in order to avoid malignant transformation.
In summary, in comparison to morphological MR features, PET adds valuable information concerning grading and prognosis in patients with newly diagnosed cerebral lesions. The diagnostic accuracy, however, is not sufficient to make a final decision on the therapeutic procedure. Therefore, a histological evaluation of suspicious brain lesions by biopsy remains necessary in most of the patients with brain tumors.
5 Treatment Monitoring
The diagnostic value of MRI and CT concerning changes in tumor size or contrast enhancement in response to therapy is limited since the known reactive transient BBB alterations with consecutive contrast enhancement may mimic tumor progression. This phenomenon, so-called pseudoprogression, is seen in 20–47 % of cases and can lead to an unnecessary overtreatment (Lustig et al. 2007) (Fig. 5).
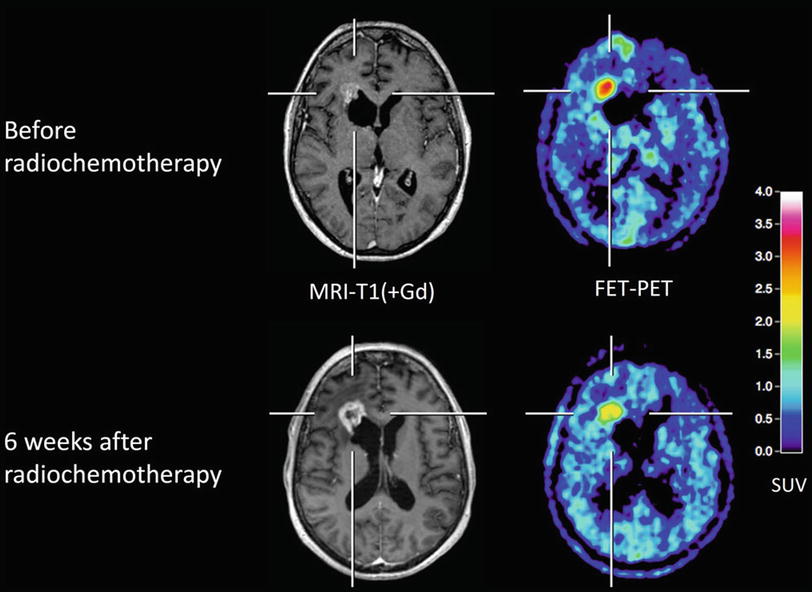
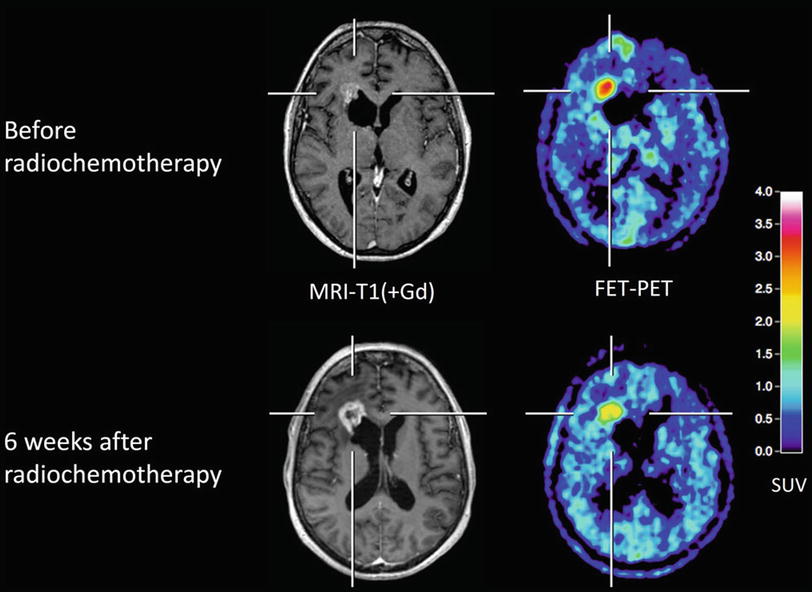
Fig. 5
Patient with a glioblastoma after resection (upper row) and 6 weeks after completion of radiochemotherapy (lower row). MRI shows enlargement of contrast enhancement after 6 weeks after completion of radiochemotherapy (lower row) suggesting tumor progression, whereas FET PET indicates a responder with decreasing amino acid uptake. The patient had a favorable outcome
FDG PET is considered not to be ideal to evaluate treatment response because of the high accumulation in nonspecific reactive changes in the tissue (Basu and Alavi 2009). The feasibility and usefulness of MET and FET PET for treatment assessment and follow-up after surgery, chemotherapy, and radiotherapy have been demonstrated in several studies. The currently available data suggest that a reduction of amino acid uptake of a glioma is a sign of a response to treatment. Recently, a prospective study evaluated the prognostic value of early changes of FET uptake after postoperative radiochemotherapy in patients with glioblastoma (RCX) (Piroth et al. 2011b; Galldiks et al. 2012c). It could be demonstrated that PET responders with a decrease of the tumor/brain ratio of more than 10 % had a significantly longer disease-free survival and overall survival than patients with stable or increasing tracer uptake after RCX. A reliable monitoring of temozolomide chemotherapy could also be demonstrated with MET in patients with recurrent HGG (Galldiks et al. 2006, 2010a) and also in some experimental therapeutic approaches like radioimmunotherapy, convection-enhanced delivery of paclitaxel, and chemotherapy with bevacizumab and irinotecan (Pöpperl et al. 2005, 2006a; Hutterer et al. 2011; Galldiks et al. 2013). Therefore, monitoring of treatment response using amino acid PET imaging is now utilized to provide an early assessment of therapy efficacy and aid oncologists to optimize therapeutic management of brain tumors.
A number of studies have examined the value of FLT PET, an imaging biomarker of cell proliferation, for treatment monitoring (Fig. 6), especially in patients with recurrent malignant glioma treated with an antiangiogenic therapy, i.e., bevacizumab, predominantly in combination with irinotecan (Chen et al. 2007; Schiepers et al. 2010; Harris et al. 2012; Schwarzenberg et al. 2012). In comparison to standard MRI, the authors found that changes of FLT uptake were highly predictive of progression-free and overall survival in patients with recurrent malignant glioma on bevacizumab therapy. FLT PET seems to be more predictive than standard MRI for early treatment response.
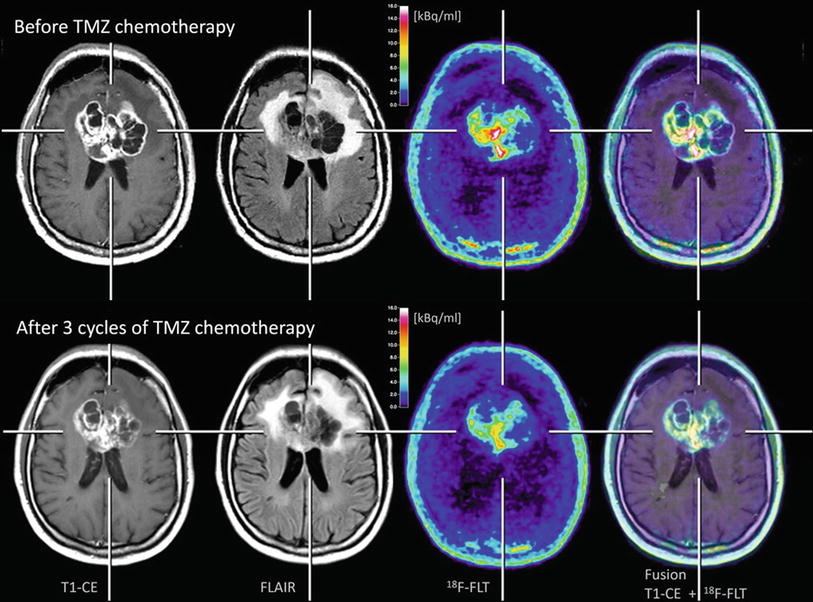
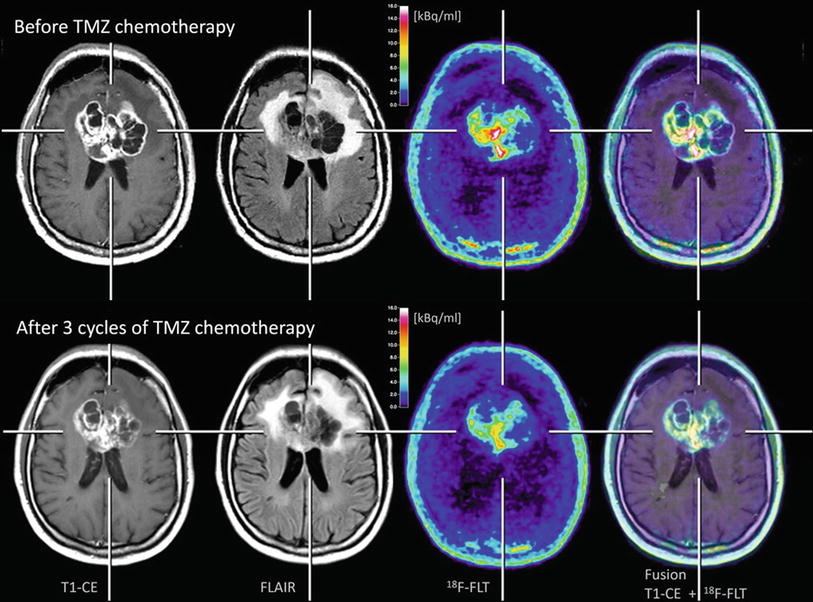
Fig. 6
Patient with a glioblastoma before (upper row) and after three cycles of temozolomide (TMZ) chemotherapy (lower row). A moderate reduction of tumor extent is observed both in the contrast-enhanced MR and FLAIR-weighted images as well as in the 18F-FLT PET scans. Furthermore, it can be observed that 18F-FLT uptake occurs not in areas without disruption of the blood-brain barrier (Courtesy of Lutz Kracht, Max-Planck-Institute for Neurological Research, Germany)
Furthermore, FMISO PET seems to have the potential to monitor treatment effects. In order to evaluate the tumor oxygenation status before and immediately after fractionated radiochemotherapy with temozolomide, two glioblastoma patients underwent serial FMISO PET studies (Narita et al. 2012). In comparison to the baseline FMISO scan, in both patients, the FMISO uptake in the tumor was notably decreased in the follow-up scan, supposing a reoxygenation of the tumor. These observations suggest that changes in the oxygenation status in glioblastoma may be suitable for monitoring of radiation therapy with concomitant temozolomide.
6 The Diagnosis of Tumor Recurrence/Progression
It is difficult to distinguish tumor recurrence/progression from nonspecific posttherapeutic changes with conventional MRI alone because pathological enhancement with contrast medium may reflect either new growth of tumor or unspecific changes after radio- or chemotherapy (Brandsma et al. 2008). In addition, progressive tumor growth may miss contrast enhancement especially under antiangiogenic therapy. The role of FDG PET in such cases is limited because of the frequency of nonspecific uptake (Ricci et al. 1998; Basu and Alavi 2009). Multiple studies have shown that MET PET is highly sensitive to detect tumor recurrence/progression, but the specificity for the differentiation of vital tumor tissue from nonneoplastic changes is limited with about 70 % (Tsuyuguchi et al. 2004; Van Laere et al. 2005; Singhal et al. 2008; Crippa et al. 2012). The accuracy of FET PET to distinguish tumor recurrence/progression from nonneoplastic changes appears to be higher compared to MET PET. The lower specificity of MET may be explained by its higher affinity for macrophages compared with FET as demonstrated in animal experiments (Salber et al. 2006, 2007). A sensitivity and specificity of FET PET for the detection of tumor recurrence/progression of 100 and 93 %, respectively, has been reported compared with 93 and 50 % for MRI alone (Pöpperl et al. 2004; Rachinger et al. 2003). The additional use of dynamic FET PET allowed a differentiation of high-grade and low-grade recurrences with a sensitivity and specificity of 92 % (Pöpperl et al. 2006b).
7 PET in Patients with Brain Metastasis
The improvement in the treatment of solid tumors has led to an increasing number of patients who experience brain metastases during the course of the disease. Stereotactic radiosurgery, brachytherapy, and whole-brain radiation therapy are commonly used to treat brain metastases and a growing percentage of patients live long enough to experience a local relapse of these metastases. Thus, the number of patients suffering local recurrence of previously irradiated brain metastases can be expected to increase. Contrast-enhanced MR imaging is the method of choice for the evaluation of metastatic brain tumors. However, in a considerable number of patients, the differentiation of local recurrent brain metastasis from radiation necrosis after radiotherapy using contrast-enhanced MRI is difficult (Dooms et al. 1986). FDG has been considered for evaluation of metastatic brain tumors, but the high physiological glucose consumption of the brain and the variable glucose uptake of metastatic brain lesions limit its use (Belohlavek et al. 2003; Lee et al. 2008). A recent study indicated that dual-phase imaging may improve the diagnostic accuracy of FDG PET for differentiation of recurrent brain metastasis from radiation necrosis (Horky et al. 2011). A limitation of that approach is the long time interval between the PET scans (range of duration, 2–5.7 h). PET using MET may be effective in differentiating recurrent metastatic brain tumor from radiation-induced changes with sensitivity and specificity of 70–80 % (Tsuyuguchi et al. 2004; Terakawa et al. 2008). The clinical usefulness of FET PET for the differentiation of local recurrent brain metastasis from radiation necrosis could be described recently in 31 patients with 40 metastases (Galldiks et al. 2012b). Using the tumor/brain ratios and results of kinetic studies, FET PET could differentiate local recurrent brain metastasis from radiation necrosis with a high sensitivity (95 %) and specificity (91 %). A first comparison of MET and 11C-choline in patients with brain metastasis indicated slightly better results for choline than for MET (Rottenburger et al. 2011).
8 Imaging of Brain Tumors in Children
The histological subtypes of brain tumors in children differ considerably from that in adults. Only few mainly retrospective studies have been performed in children with brain tumors. It is, however, evident that the assessment of glucose metabolism with FDG is less suitable for the evaluation of tumor malignancy than it is the case in adults (Weckesser et al. 2001). The main reason for this is the high glucose metabolism in pilocytic astrocytomas. These low-grade tumors may exhibit metabolic rates with the intensity of gray matter; an association of the metabolic activity of the tumor and clinical presentation or outcome is not evident.
Previous studies revealed that the use of amino acid PET with the tracer MET may improve the management in this patient population (Utriainen et al. 2002; Pirotte et al. 2003; Galldiks et al. 2010b). Results of these studies suggest that MET PET might be a useful tool to differentiate tumorous from nontumorous lesions in children and young adults when a decision for further therapy is difficult or impossible from routine structural imaging procedures alone.
However, it should be noted that the differentiation between high- and low-grade gliomas using amino acid PET may be difficult. A considerable overlap of amino acid uptake has been observed in low-grade and high-grade tumors (Utriainen et al. 2002). Similar to glucose metabolism, amino acid uptake may be high in low-grade tumors like pilocytic astrocytomas and gangliogliomas, and uptake may be relatively low in highly aggressive medulloblastomas (WHO IV).
9 Perspectives
Molecular imaging of cerebral gliomas with PET is becoming more and more available for clinical use. While most of the techniques cited in this review have limited influence on diagnostic practice, the use of radiolabeled amino acids is promising and permits a more specific representation of the spatial extent of solid and diffuse glioma tissue than is possible by conventional MRI alone. This is very advantageous for the planning of biopsies, resections, and radiotherapy. Furthermore, tumor recurrence/progression can be differentiated from posttherapeutic changes with a high degree of specificity, valuable prognostic information can be obtained for low-grade gliomas, and the treatment response can probably be judged early in the course of treatment. The scientifically documented impact of PET in brain tumors seems to justify its use as a routine diagnostic technique for certain indications, but it remains to be confirmed that this will improve the overall quality of care (e.g., improvement of survival). The logistical prerequisites especially for amino acid imaging have become markedly less difficult to achieve in recent years. The costs of these diagnostic techniques would appear to be well justified by their clinical utility, not least because their timely application in a larger number of patients can be expected to save the costs incurred today by the use of other, less diagnostically reliable techniques (Heinzel et al. 2012a, b, 2013).
References
Arbizu J, Tejada S, Marti-Climent JM, Diez-Valle R, Prieto E, Quincoces G, Vigil C, Idoate MA, Zubieta JL, Penuelas I, Richter JA (2012) Quantitative volumetric analysis of gliomas with sequential MRI and (11)C-methionine PET assessment: patterns of integration in therapy planning. Eur J Nucl Med Mol Imaging 5:771–781CrossRef
Bading JR, Shields AF (2008) Imaging of cell proliferation: status and prospects. J Nucl Med (official publication) 49(Suppl 2):64S–80S
Belohlavek O, Simonova G, Kantorova I, Novotny J Jr, Liscak R (2003) Brain metastases after stereotactic radiosurgery using the Leksell gamma knife: can FDG PET help to differentiate radionecrosis from tumour progression? Eur J Nucl Med Mol Imaging 30:96–100PubMedCrossRef
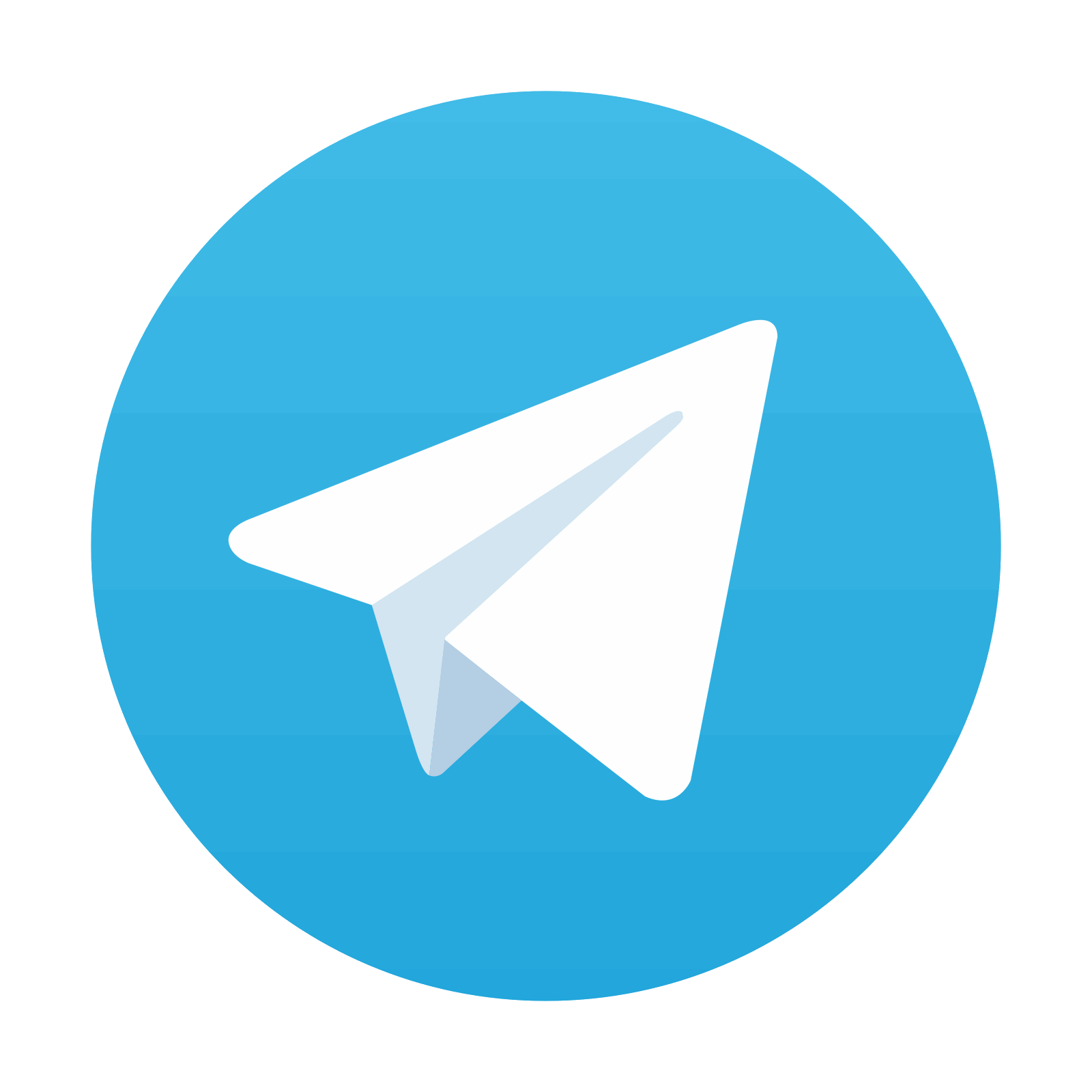
Stay updated, free articles. Join our Telegram channel

Full access? Get Clinical Tree
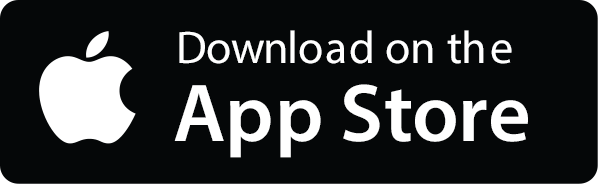
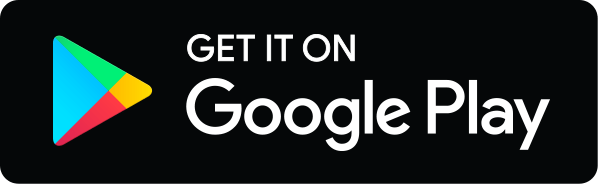