Fig. 23.1
A 21-year-old female sprinter with calf pain who has a grade 2 tibial stress injury. (a) Axial fat-suppressed T2-weighted MRI of the calf shows periosteal edema along the medial and posterior cortex of the tibial diaphysis (arrows). (b) Corresponding coronal fat-suppressed T2-weighted MRI of the calf shows periosteal edema along the medial cortex of the tibial diaphysis (arrowheads) with mild bone marrow edema within the intramedullary canal (arrow)
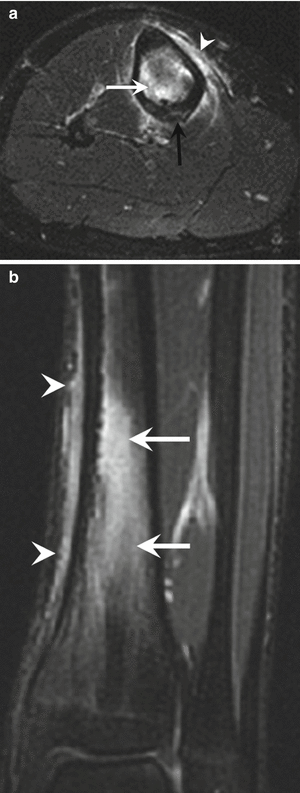
Fig. 23.2
An 18-year-old long-distance female runner with calf pain who has a grade 4 tibial stress injury. (a) Axial fat-suppressed T2-weighted MRI of the calf shows periosteal edema along the medial and posterior cortex of the tibial diaphysis (white arrowhead) with extensive bone marrow edema within the intramedullary canal (white arrow). Also note the low signal fracture line through the posterior cortex of the tibia (black arrow). (b) Corresponding coronal fat-suppressed T2-weighted MRI of the calf shows periosteal edema along the medial cortex of the tibial diaphysis (arrowheads) with extensive bone marrow edema within the intramedullary canal (arrows)
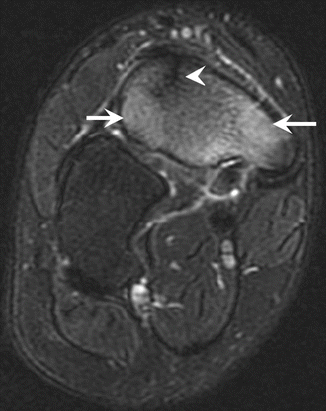
Fig. 23.3
A 22-year-old male hurdler with foot pain who has a grade 4 navicular stress injury. Coronal fat-suppressed T2-weighted MRI of the foot shows extensive bone marrow edema within the navicular (arrows) with a low signal fracture line through the dorsal cortex (arrowhead)
23.3.2 Muscle Strains
Muscle strains are the most common injury in track and field athletes and are caused by excessively forceful muscle contraction (Alonso et al. 2009, 2010, 2012). Strains typically occur in muscles which cross two joints, contain a high proportion of fast twitch fibers, and undergo eccentric contraction. Muscle strains primarily occur during powerful eccentric contraction because more tension is generated within the muscle during eccentric contraction than during concentric contraction (Linklater et al. 2010; Shelly et al. 2009; Slavotinek 2010). The most commonly injured muscles in track and field athletes are the hamstrings, quadriceps, and gastrocnemius muscles. However, strains of the adductors, abductor, and soleus muscles may also occur (Linklater et al. 2010; Shelly et al. 2009; Slavotinek 2010). Muscle strains cause disruption of capillary vessels with subsequent hemorrhage into the muscle which generates an acute inflammatory response with increased vascular permeability and accumulation of interstitial fluid (Jarvinen et al. 2005).
Muscle strains typically occur at the myotendinous junction which is the weakest link in the muscle. The myotendinous junction includes not only the point where the tendon emerges free from the muscle belly but also extends deeper into the muscle where the muscle fibers lie in close proximity to the centrally located tendon (Noonan and Garrett 1992). Hamstring muscle strains may occur in variable locations at the myotendinous junction with 33 % of injuries occurring at the proximal myotendinous junction, 53 % of injuries at the intramuscular myotendinous junction, and 13 % of injuries at the distal myotendinous junction. The biceps femoris is the most commonly injured hamstring muscle in track and field athletes (De Smet and Best 2000). Quadriceps muscle strains typically involve the rectus femoris muscle and less commonly the vastus muscles with the site of injury in the rectus muscle being equally distributed between adjacent to the central tendon and at the periphery of the muscle belly (Cross et al. 2004). The vast majority of gastrocnemius muscle strains involve the distal myotendinous junction of the medial head of the gastrocnemius muscle (Weishaupt et al. 2001).
MRI is useful for evaluating the extent of muscle injury and to grade the injury according to the degree of muscle fibers disruption (De Smet et al. 1990; Palmer et al. 1999; Kneeland 1997). Grade 1 strains represent a minor degree of microscopic tearing with no macroscopic muscle defect. On MRI, these injuries show increased signal intensity of the muscle on fluid-sensitive sequences with no discernible disruption of muscle fibers. Grade 2 strains or partial muscle tears represent an incomplete disruption of muscle fibers. On MRI, these injuries show edema and hemorrhage at the myotendinous junction with extension of the signal abnormality into the muscle belly and along the intramuscular and perimuscular fascia (Fig. 23.4). The identification of a hematoma at the myotendinous junction is helpful for distinguishing between grades 1 and 2 muscle strains (Fig. 23.5). Grade 3 strains or complete muscle tears represent complete disruption of muscle fibers with complete or near complete loss of muscle function. On MRI, these injuries show complete discontinuity of muscle fibers at the myotendinous junction with an associated hematoma and extensive surrounding intramuscular and perifascial edema. The musculotendinous unit is completely detached from its origin or insertion site, and the edges of the torn tendon are occasionally retracted (Fig. 23.6) (De Smet et al. 1990; Palmer et al. 1999; Kneeland 1997).
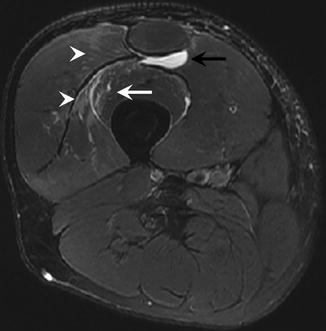
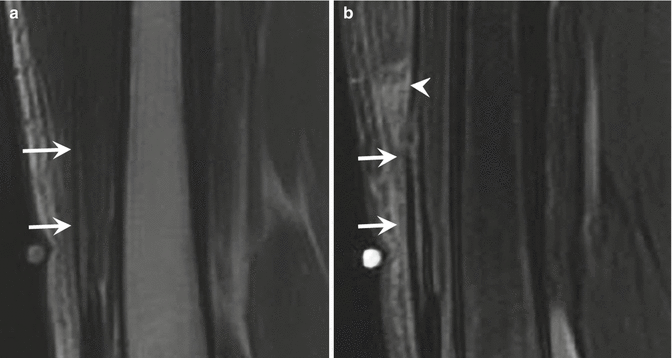
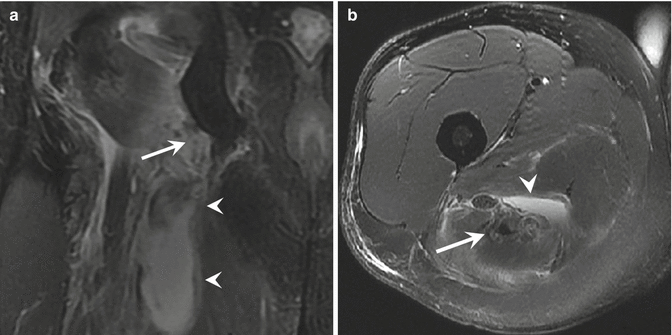
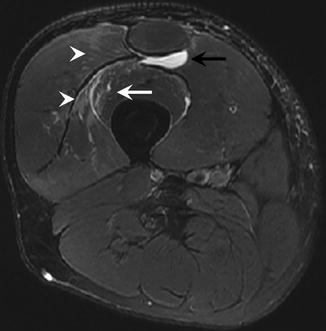
Fig. 23.4
A 23-year-old male sprinter with anterior thigh pain who has a grade 2 quadriceps muscle strain. Axial fat-suppressed T2-weighted MRI of the thigh shows edema within the vastus lateralis muscle (white arrowheads) and vastus intermedius muscle (white arrow) with a fluid collection within the adjacent intermuscular fascial plane (black arrow)
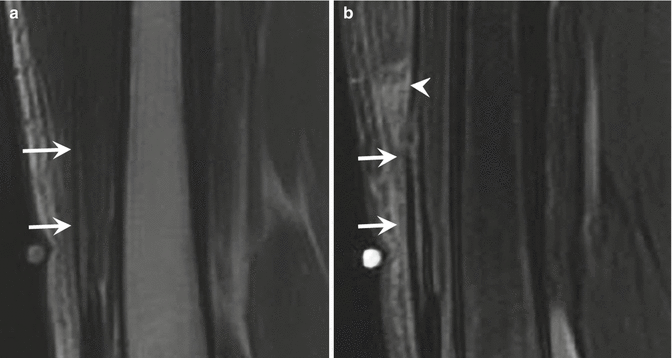
Fig. 23.5
A 19-year-old male hurdler with anterior thigh pain who has a grade 2 quadriceps muscle strain. (a) Sagittal T1-weighted MRI of the thigh shows a high signal intensity hematoma at the partially torn myotendinous junction of the quadriceps tendon (arrows). (b) Corresponding sagittal fat-suppressed T2-weighted MRI of the thigh shows a hematoma at the partially torn myotendinous junction of the quadriceps tendon (arrows) with edema more proximally within the rectus femoris muscle (arrowhead)
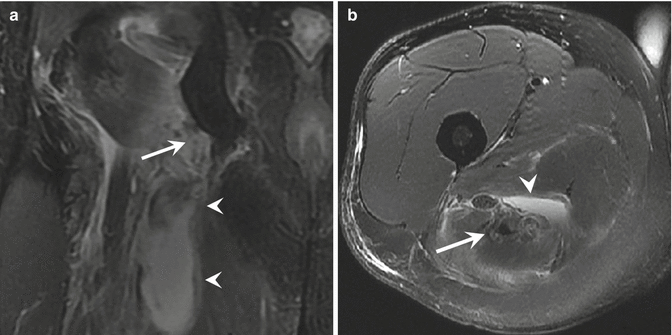
Fig. 23.6
A 24-year-old male sprinter with posterior thigh pain who has a grade 3 hamstring muscle strain. (a) Coronal fat-suppressed T2-weighted MRI of the thigh shows complete absence of the hamstring tendon origin at the ischial tuberosity (arrow) with a large fluid collection within the adjacent intermuscular fascial plane (large arrowheads). (b) Corresponding axial fat-suppressed T2-weighted MRI of the thigh shows the retracted hamstring tendon origin located more distally within the thigh (arrow) with a fluid collection within the adjacent intermuscular fascial plane (arrowhead)
Healing muscle strains on MRI are characterized by decreased amounts of high signal edema within the injured muscle on fluid-sensitive sequences. The degree to which muscle edema resolves on MRI is highly variable (Linklater et al. 2010; Shelly et al. 2009; Slavotinek 2010; Blankenbaker and Tuite 2010). Persistent edema following muscle strain implies ongoing healing as the healing response continues on the ultrastructural level for weeks and even months following an athlete’s return to competition (Fig. 23.7). As the healing process continues, edema within the strained muscle resolves, and there may be variable amounts of fatty atrophy and compensatory hypertrophy of the injured muscle. Focal areas of low T1 and T2 signal intensity can also be seen at the myotendinous junction and are due to scar formation and hemosiderin deposition following hemorrhage (Fig. 23.8). The formation of scar tissue at the site of muscle strain is common and can be seen as early as 6 weeks following muscle injury (Linklater et al. 2010; Shelly et al. 2009; Slavotinek 2010; Blankenbaker and Tuite 2010).
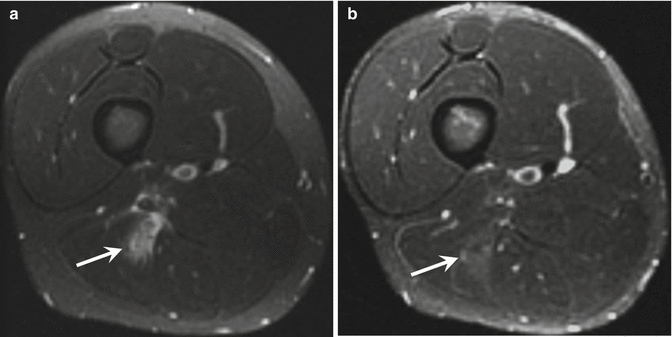
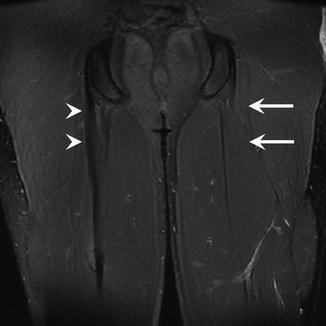
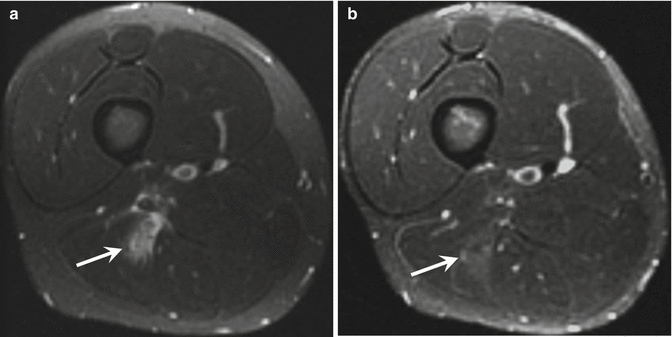
Fig. 23.7
A 19-year-old female sprinter with posterior thigh pain who has a grade 1 hamstring muscle strain. (a) Axial fat-suppressed T2-weighted MRI of the thigh performed at the time of injury shows mild edema within the biceps femoris muscle (arrow). (b) Axial fat-suppressed T2-weighted MRI of the thigh acquired 8 weeks after injury shows persistent edema with the biceps femoris muscle (arrow) despite the fact that the patient has successfully returned to sports activity
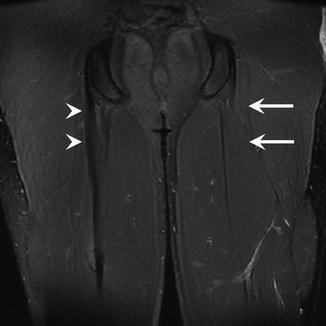
Fig. 23.8
A 20-year-old male sprinter with a prior history of hamstring muscle strain. Coronal fat-suppressed T2-weighted MRI of the thigh shows extensive low signal intensity at the hamstring myotendinous junction representing scar formation from a prior injury (arrowheads). Note the difference in appearance when compared to the normal hamstring myotendinous junction of the contralateral thigh (arrows)
MRI may provide prognostic information regarding the time to return to sports activity following muscle injuries. Askling and associates prospectively evaluated 18 elite sprinters with hamstring muscle strains diagnosed on MRI and reported that the median time to return to competitive activity was 16 weeks with a range between 4 weeks and 50 weeks (Askling et al. 2006). In a second study using the same patient cohort, the authors investigated the association between the time to return to competitive activity and the size and location of the hamstring muscle strain on MRI. There was a significant correlation between the time to return to sports activity and the volume of muscle edema on fluid-sensitive sequences. In addition, athletes with injuries located at or near the proximal myotendinous junction had a significantly longer recovery time than athletes with injuries located more distally within the hamstring muscle (Askling et al. 2007).
23.4 Knee Injuries
Knee injuries frequently occur in track and field athletes (van Gent et al. 2007). Knee injuries are especially prevalent in long-distance runners and are more commonly caused by chronic repetitive stress than acute trauma. In a retrospective review of 2002 injuries identified over a 2 year period in adult runners, Taunton and associates found that patellofemoral pain syndrome, iliotibial band friction syndrome, and patellar tendonitis were the first, second, and sixth most common diagnoses, respectively (Taunton et al. 2002). In a retrospective study evaluating 748 high school cross-country athletes over a single season, Tenforde and associates found that patellofemoral pain syndrome and iliotibial band friction syndrome were the third and fifth most common injuries, respectively (Tenforde et al. 2011). However, knee pain due to internal derangement is relatively uncommon in track and field athletes. Swenson and associated reported that track and field had one of the lowest rates of meniscus, anterior cruciate ligament, and medial collateral ligament injuries of the 20 sports recorded in the American High School Sports-Related Injury Surveillance System over a 5 year period (Swenson et al. 2013).
Patellofemoral pain syndrome is the most common cause of knee pain in runners (Taunton et al. 2002; Tenforde et al. 2011). While the exact etiology of patellofemoral pain syndrome is unclear, the disorder is thought to be due to abnormal motion of the patella within the femoral trochlea during knee flexion and extension. Various factors may cause abnormal patellofemoral biomechanics including patella alta, trochlear dysplasia, femoral anteversion, external tibial torsion, genu valgum, forefoot hyperpronation, lateral patellofemoral retinaculum tightness, and imbalance between medial and lateral quadriceps musculature. However, studies on the biomechanical factors that potentially contribute to patellofemoral pain syndrome demonstrate conflicting results, suggesting that the disorder is multifactorial in nature. Proper patellofemoral biomechanics requires balanced forces acting on the patella. If any force is too large or too small, alterations in normal patellar motion during knee flexion and extension may occur which places increased stress on the patella and adjacent soft tissue structures of the anterior knee. Patients with patellofemoral pain syndrome typically present with insidious onset of anterior knee pain (Collado and Fredericson 2010). MRI can detect areas of cartilage degeneration and bone marrow edema within the patella in these individuals which are due to chronic repetitive overload injury to the articular cartilage and underlying bone secondary to abnormal patellofemoral biomechanics (Fig. 23.9) (Chhabra et al. 2011).
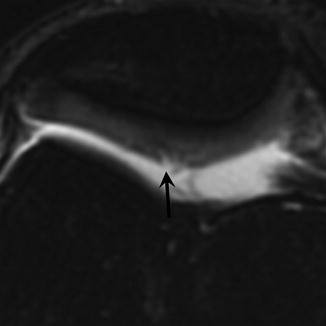
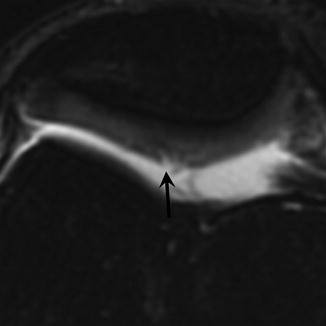
Fig. 23.9
A 25-year-old female long-distance runner with anterior knee pain who has patellofemoral pain syndrome. Axial fat-suppressed T2-weighted MRI of the knee shows superficial cartilage degeneration on the median ridge of the patella (arrow)
Iliotibial band friction syndrome is also a common cause of knee pain in runners (Taunton et al. 2002; Tenforde et al. 2011). The iliotibial band is formed by the coalescence of the fascia of the gluteus maximus, gluteus medius, and tensor fascia lata muscles at the level of the greater trochanter. The iliotibial band attaches to the supracondylar tubercle and intermuscular septum proximal to the knee joint and then continues distally to attach to the Gerdy tubercle on the anterior lateral tibia. The tense fibers of the iliotibial band move forward in extension and backward in flexion (Terry et al. 1986). Iliotibial band syndrome is due to repetitive friction between the iliotibial band and the adjacent lateral femoral epicondyle at 30° of knee flexion in athletes who perform strenuous physical activity (Noble 1980; Orchard et al. 1996). MRI findings of iliotibial band syndrome have been well described and typically consist of ill-defined edema superficial and deep to the distal iliotibial band. Thickening of the iliotibial band and a circumscribed fluid collection between the iliotibial band and the adjacent lateral femoral epicondyle may also be present (Fig. 23.10) (Murphy et al. 1992; Muhle et al. 1999). MRI is also useful to exclude other causes of lateral knee pain in runners including injuries to the lateral meniscus, lateral collateral ligament, popliteal tendon, and biceps femoris muscle (Noble 1980; Orchard et al. 1996).
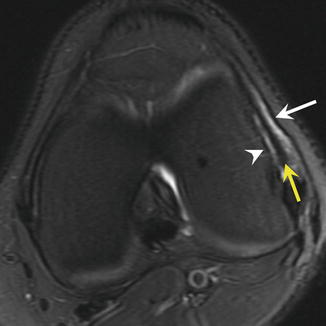
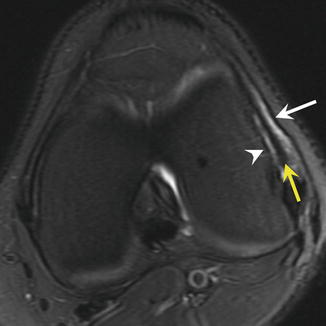
Fig. 23.10
A 22-year-old female long-distance runner with anterior lateral knee pain who has iliotibial band syndrome. Axial fat-suppressed T2-weighted MRI of the knee shows edema superficial (white arrow) and deep (white arrowhead) to the thickened iliotibial band (yellow arrow)
Patellar tendonitis is also a common knee injury in track and field athletes especially in long-distance runners and jumpers (Taunton et al. 2002; Tenforde et al. 2011). Patellar tendonitis is more prevalent in male than female athletes (Lian et al. 2005) and is commonly associated with abnormal patellofemoral biomechanics (Allen et al. 1999). Histologic features of the disease include collagen fiber disruption, mucoid degeneration, and fibrinoid necrosis of the patellar tendon with absence of associated inflammation (Martens et al. 1982). Thus, the term “tendonitis” is a misnomer as inflammatory changes, if present, are not the cause of the disease but rather a response to the pathologic process. Athletes with patellar tendonitis typically present with anterior knee pain associated with running and jumping which is initially intermittent but may become persistent (Martens et al. 1982; Roels et al. 1978). MRI shows thickening of the patellar tendon with increased intrasubstance signal which is especially prominent on fluid-sensitive sequences. The thickening and intrasubstance signal abnormality are primarily located within the proximal portion of the patellar tendon, but diffuse involvement may occur in the advanced stages of the disease process. Associated inflammatory changes in the patellar bone marrow and Hoffa fat pad may also be present (Fig. 23.11) (el-Khoury et al. 1992; Yu et al. 1995).
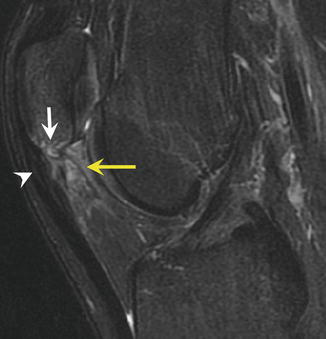
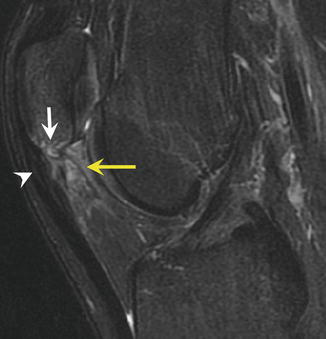
Fig. 23.11
A 19-year-old female high jumper with anterior knee pain who has patellar tendonitis. Sagittal fat-suppressed T2-weighted MRI of the knee shows thickening and increased signal intensity of the proximal patellar tendon (white arrowhead) with surrounding edema within the inferior pole of the patella (white arrow) and Hoffa fat pad (yellow arrow)
23.5 Ankle and Foot Injuries
Ankle and foot injuries are common in track and field athletes especially in individuals participating in long-distance running, jumping, and pole vaulting. Taunton and associates found that plantar fasciitis and Achilles tendinitis were the third and seventh most common injuries, respectively, in adult runners (Taunton et al. 2002), while Tenforde and associates found that ankle sprains, Achilles tendinitis, and plantar fasciitis were the second, fourth, and sixth most common injuries, respectively, in high school cross-country athletes (Tenforde et al. 2011). Rebella and associates also reported that ankle sprains were the most common injury in high school pole vaulters (Rebella et al. 2008). Chronic repetitive stress to the osseous structures of the ankle and foot in track and field athletes can also cause navicular, metatarsal, and talar stress injuries and sesamoiditis especially in sprinters, hurdlers, and jumpers (Bennell et al. 1996a).
Ankle sprains are common in track and field athletes (Malliaropoulos et al. 2006, 2009). Injuries to the lateral ankle ligaments are much more common than injuries to the medial ankle ligaments (Ferran and Maffulli 2006). Since the vast majority of ankle sprains are caused by forced inversion of the plantar-flexed ankle during running and jumping, the anterior talofibular ligament is the initial site of injury followed by the calcaneofibular and posterior talofibular ligaments (van Dijk et al. 1996). Most ankle sprains consist of isolated tears of the anterior talofibular ligament with full-thickness tears more common than partial-thickness tears. Combined tears of the anterior talofibular and calcaneofibular ligaments occur in 20–40 % of ankle sprains with tears of the posterior talofibular ligament extremely uncommon (Brostrom 1964; Kreitner et al. 1999). MRI findings of acute ligament sprain include irregularity, poor definition, and increased signal intensity of the ligament in a partial-thickness tear and complete disruption of the ligament in a full-thickness tear. Associated soft tissue edema and hemorrhage, joint effusion, and bone marrow edema may also be present (Fig. 23.12) (Kreitner et al. 1999; Rijke et al. 1993; Erickson et al. 1991; Nishimura et al. 1996). Bone marrow edema within the medial malleolus and medial talar dome following an acute ankle inversion injury has been associated with a full-thickness anterior talofibular ligament tear, a longer delay in return to sports activity, and persistent medial joint line pain (Chan et al. 2013). MRI findings of chronic ankle sprain consist of thickening or attenuation of the torn ligament with absence of surrounding soft tissue edema and hemorrhage (Labovitz et al. 1998).
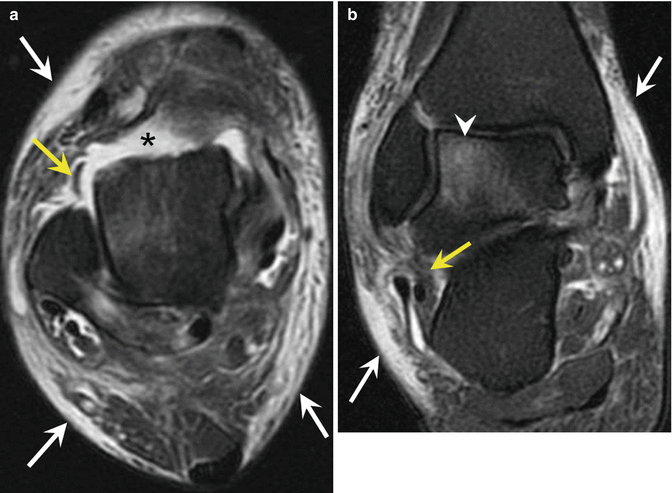
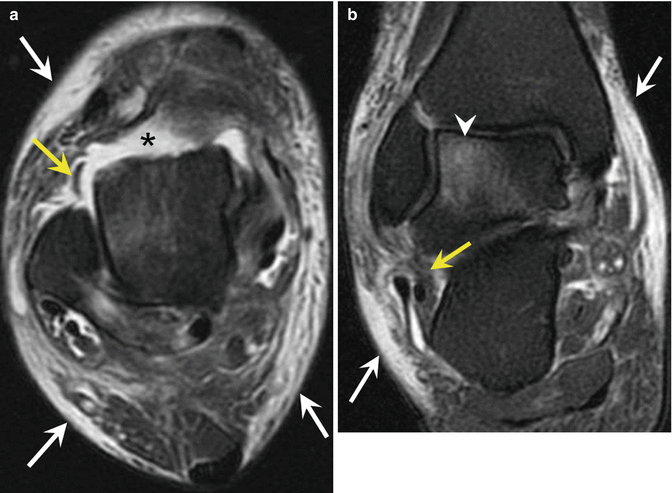
Fig. 23.12
A 20-year-old male pole vaulter with ankle pain who has a severe ankle sprain. (a) Axial fat-suppressed T2-weighted MRI of the ankle shows a full-thickness retracted tear of the anterior talofibular ligament (yellow arrow) with extensive surrounding soft tissue edema (white arrows) and a large joint effusion (*). (b) Corresponding coronal fat-suppressed T2-weighted MRI of the ankle shows and irregularity and increased signal intensity of the calcaneofibular ligament consistent with a partial-thickness tear (yellow arrow) with extensive surrounding soft tissue edema (white arrows) and bone marrow edema within the talar dome (arrowhead)
Plantar fasciitis is a frequent cause of heel pain in track and field athletes and is especially common in long-distance runners (DeMaio et al. 1993; Leach et al. 1983). The plantar aponeurosis is a thick band of fibrous tissue which arises from the plantar aspect of the posterior medial calcaneal tuberosity and divides into central, medial, and lateral components as it widens and courses distally into the forefoot (Hedrick 1996; Moraes do Carmo et al. 2008). The plantar fascia functions to elevate the longitudinal arch of the foot and to transmit large forces between the hindfoot and forefoot during the stance phase of gait (Thordarson et al. 1997; Erdemir et al. 2004). Plantar fasciitis is a low-grade inflammatory process involving the plantar aponeurosis and adjacent perifascial structures and is caused by chronic repetitive microtrauma associated with running and jumping (DeMaio et al. 1993; Leach et al. 1983). Various biomechanical factors may predispose an athlete to develop plantar fasciitis including pes cavus, forefoot hyperpronation, and external tibial torsion (Kwong et al. 1988). MRI findings of plantar fasciitis include thickening and increased signal intensity of the plantar aponeurosis with adjacent perifascial soft tissue edema and reactive bone marrow edema within the calcaneal tuberosity (Fig. 23.13). Tears within the plantar aponeurosis may also occur in more severe forms of the disease and appear as focal areas of fluid signal intensity within the thickened plantar fascia (Theodorou et al. 2000; Roger and Grenier 1997; Grasel et al. 1999).
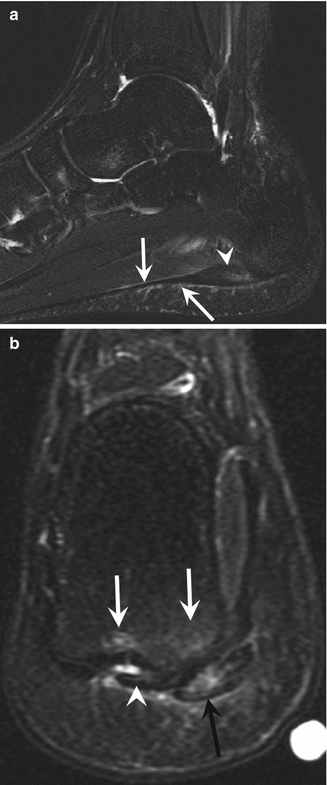
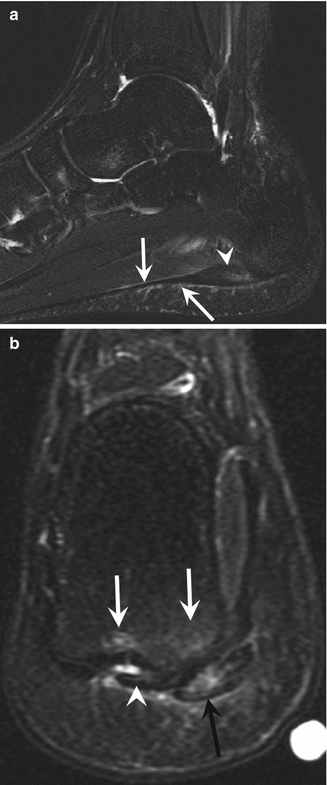
Fig. 23.13
A 24-year-old female long-distance runner with heel pain who has plantar fasciitis. (a) Sagittal fat-suppressed T2-weighted MRI of the foot shows thickening and increased signal intensity of the plantar fascia (arrowhead) with surrounding perifascial soft tissue edema (arrows). (b) Corresponding coronal fat-suppressed T2-weighted MRI of the foot shows thickening and increased signal intensity of the plantar fascia (black arrow) with surrounding perifascial soft tissue edema (white arrows) and bone marrow edema within the calcaneal tuberosity (white arrowhead)
Achilles tendinopathy is a frequent cause of posterior ankle pain in track and field athletes and is especially common in long-distance runners (DeMaio et al. 1993; Leach et al. 1983). In fact, Achilles tendinopathy was the most common injury reported in a recent study of elite runners (Kingma et al. 2007). The Achilles tendon is formed by the confluence of the gastrocnemius and soleus tendons. As it courses distally through the posterior calf, the Achilles tendon twists laterally by 90° so that the tendon fibers of the gastrocnemius and soleus muscles insert onto the posterior lateral and posterior medial aspects of the calcaneal tuberosity, respectively (Chao et al. 1997). The Achilles tendon is the strongest tendon in the body and can absorb tremendous energy before strain injury occurs (Kader et al. 2002) which is fortunate as loading of the Achilles tendon during running exceeds 12.5 times body weight (Kader et al. 2002; Komi et al. 1992). Various biomechanical factors predispose an athlete to develop Achilles tendinopathy including overuse, lack of flexibility, training errors, excessive lateral heel strike, altered knee kinematics, and reduced proximal muscle activity (Azevedo et al. 2009; Smart et al. 1980; van Sterkenburg and van Dijk 2011). Histologic features of the disease include mucoid degeneration and fibrinoid necrosis of the Achilles tendon (DeMaio et al. 1995). MRI findings of Achilles tendinopathy include thickening and increased signal intensity of the Achilles tendon with adjacent peritendinous soft tissue edema and reactive bone marrow edema within the calcaneal tuberosity (Fig. 23.14) (Quinn et al. 1987; Karjalainen et al. 2000; Movin et al. 1998).