Fig. 5.1
Brain shift. Preoperative FLAIR image (a) shows a hyperintense lesion in the right frontal lobe. Intraoperative FLAIR image (b) shows partial resection of the lesion and a change in the overall morphology of the surrounding right frontal lobe parenchyma
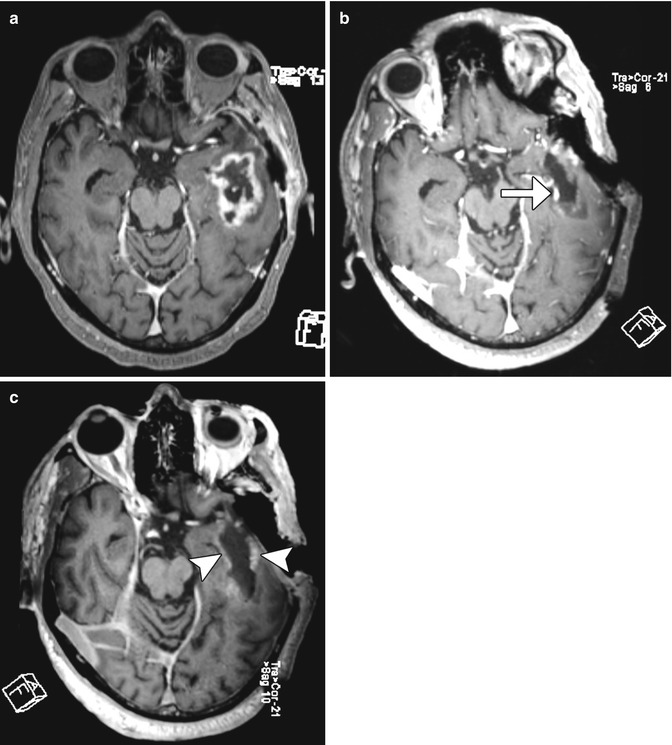
Fig. 5.2
Enhancing tumor resection and contrast leakage. Initial axial post-contrast T1-weighted image (a) shows a peripherally enhancing left temporal lobe glioblastoma. Axial post-contrast T1-weigthted MRI (b) obtained after the first resection attempt shows a punctate focus of nodular enhancement in the medial resection bed (arrow), which represented residual tumor. Axial post-contrast T1-weigthted MRI (c) obtained after further resection shows that there are no longer residual enhancing tumor components. Faint enhancement along the margins of the resection cavity represents contrast leakage (arrowheads)
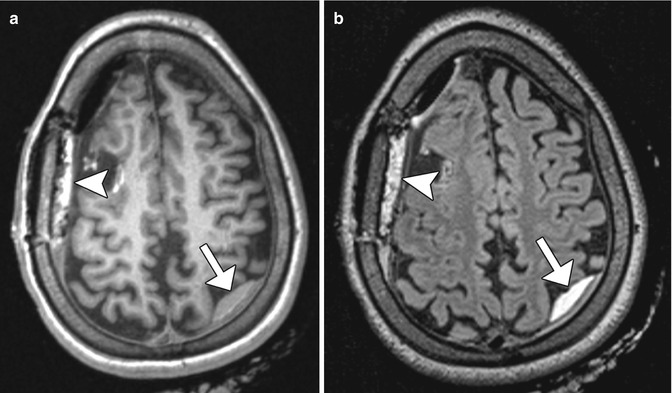
Fig. 5.3
Hyperacute hemorrhage and hemostatic material. Axial T1- (a) and T2-FLAIR (b) intraoperative MR images obtained at the end of right frontal lobe tumor resection show a small left parietal convexity subdural hematoma with intermediate T1 and high T2 signal (arrows). The hemostatic agent in the extradural space along the right frontal convexity surgical bed displays high T1 and T2 signal (arrowheads)
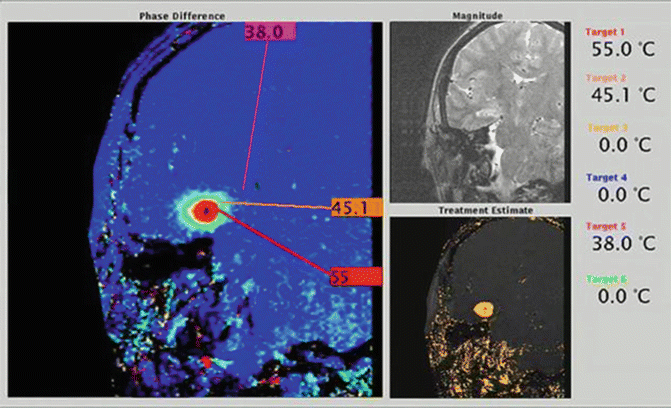
Fig. 5.4
Laser ablation. MR thermography performed during ablation of the right hippocampus shows real-time temperature monitoring and irreversible damage model (Courtesy of Amy Schneider, Medtronic)
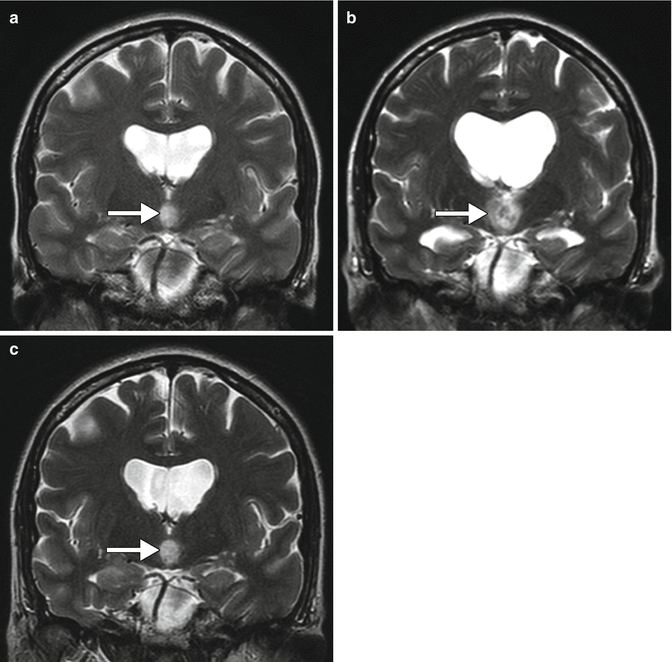
Fig. 5.5
Transient tumor swelling after laser ablation. Preoperative coronal T2-weighted MRI (a) shows a hyperintense hypothalamic tumor, which proved to be a pilocytic astrocytoma (arrow). The coronal T2-weighted MRI (b) obtained 1 week after laser ablation of the tumor when the patient developed memory formation difficulties shows increase in size of the tumor (arrows) and lateral ventricles. Follow-up coronal T2-weighted MRI (c) after steroid taper shows interval decrease in size of the tumor (arrow) and lateral ventricles
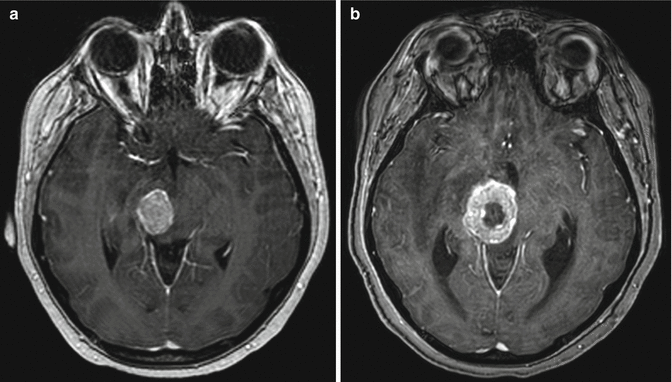
Fig. 5.6
Tumor progression after laser ablation. Preoperative axial T1-weighted MRI (a) shows a homogeneously enhancing right midbrain tumor. Axial T1-weighted MRI (b) obtained over 1 month after laser ablation shows central necrosis, but overall increase in size of the enhancing tumor
5.2 Brain Tumor Surgery and Treatment Accessories
5.2.1 Stereotactic Biopsy
5.2.1.1 Discussion
Stereotactic biopsy is an image-guided procedure that is commonly performed to obtain tissue samples of intracranial lesions. Hemorrhage is one of the most common findings after stereotactic brain biopsy, occurring in up to 9% of cases. Hemorrhage along the biopsy trajectory has a characteristic linear configuration. Small amounts of blood products along the path of the biopsy that may only be discernible on T2* GRE or SWI sequences are usually of no clinical concern and resolve spontaneously. Rather, such findings serve to delineate the path of the biopsy needle and can help account for new neurological deficits (Fig. 5.7). In addition, the blood products left along the biopsy path that are apparent on MRI can also serve as a useful indicator of whether the lesion was appropriately sampled. Nevertheless, some operators prefer to insert a metal marker in the biopsy cavity as a reliable indicator that is visible on imaging (Fig. 5.8). Although off-target biopsy can yield tumor cells if the lesion is an infiltrative tumor, the grade may be underestimated. Ideally, biopsy of the enhancing portion of the tumor with the highest cerebral blood volume (CBV) on perfusion-weighted MRI should be performed. Another uncommon, but notable complication of stereotactic biopsy is tumor seeding (Fig. 5.9). Otherwise, a mild degree of enhancement in the brain parenchyma along the biopsy path is often encountered on early postoperative imaging as an incidental finding that typically resolves within a couple of months (Fig. 5.10).
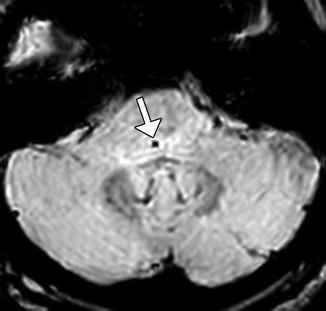
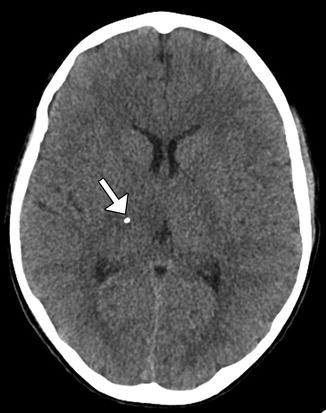
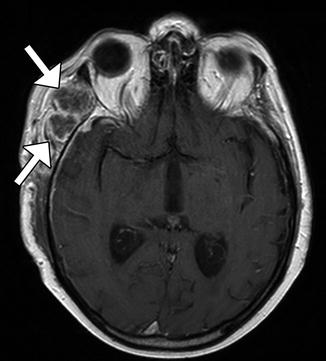
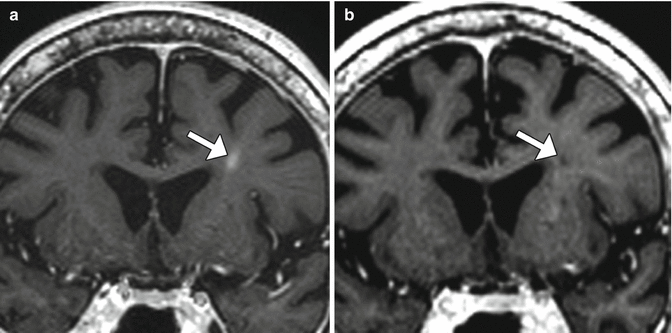
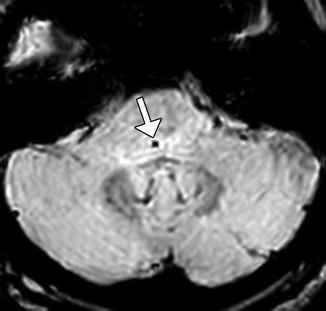
Fig. 5.7
Blood products along the path of biopsy. The patient experienced new right-sided abducens palsy after right transfrontal biopsy of a medulla lesion. Axial SWI shows susceptibility effect along the expected location of the right abducens nucleus/nerve (arrow)
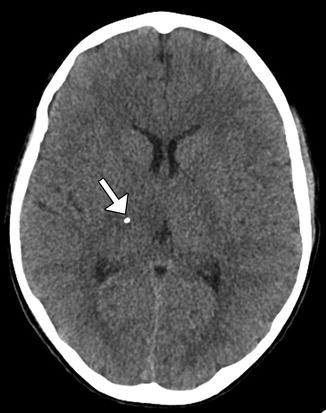
Fig. 5.8
Biopsy cavity marker. Axial CT image shows a titanium clip (arrow) deposited in the right thalamocapsular junction biopsy site, not to be mistaken for hemorrhage or an unintended foreign body
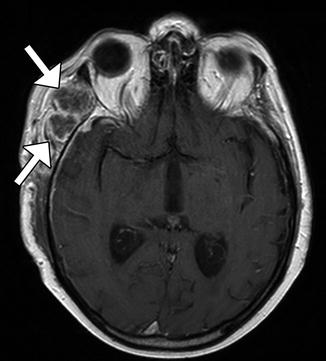
Fig. 5.9
Tumor seeding. Axial post-contrast T1-weighted MRI shows necrotic tumors (arrows) in the right temporal fossa, near the surgical approach in a patient with renal cell carcinoma that had metastasized to the brain
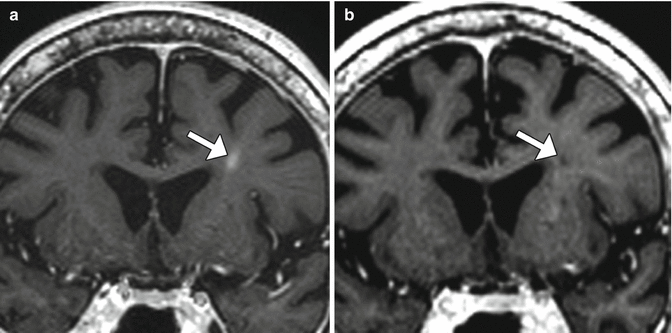
Fig. 5.10
Expected biopsy path enhancement. Initial coronal post-contrast T1-weighted MRI (a) obtained soon after left transfrontal biopsy shows enhancement along the path of the biopsy needle (arrow). Follow-up coronal post-contrast T1-weighted MRI (b) obtained 3 months later shows that the enhancement has resolved, leaving behind a small area of low signal due to encephalomalacia (arrow)
5.2.2 Resection Cavities
5.2.2.1 Discussion
The space that remains after a tumor is surgically removed is known as the resection cavity. The resection cavity is often lined or packed with hemostatic agents (refer to Chap. 4) and contains variable amounts of cerebrospinal fluid and blood products, especially during the early postoperative period (Fig. 5.11). Oftentimes, resection cavities eventually shrink and collapse, becoming nearly imperceptible (Fig. 5.12), although some cavities stay the same size, particularly if they communicate with the ventricular system.
Variable amounts of tumor may remain adjacent to the cavity depending on whether gross total, near-total, or subtotal resection was performed. The extent of tumor resection depends on several factors, including the location and type of tumor. Tumors that involve eloquent parts of the brain, that are in technically difficult areas to reach, or that involve critical structures, such as cranial nerves or major arteries, can limit the extent of tumor resection. Similarly, it is more difficult to achieve complete resection of infiltrative tumors than well-defined tumors. Ultimately, there is often a trade-off between removing as much tumor as possible versus preserving as much normal tissue and avoiding complications. Comparison with preoperative imaging should be performed when possible to help identify residual tumor.
Surgically induced parenchymal injury, postoperative hemorrhage, and enhancing conditions related to brain tumor surgery and adjunctive treatments are discussed in the following sections.
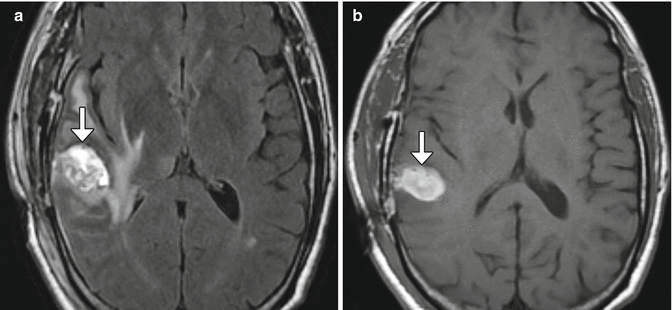
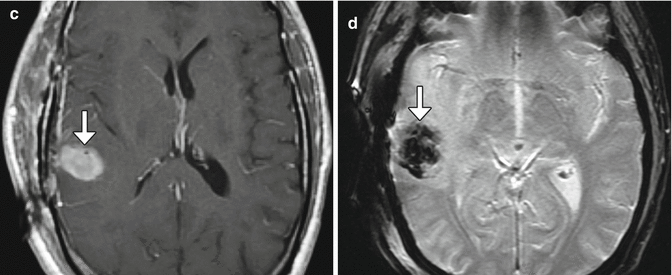
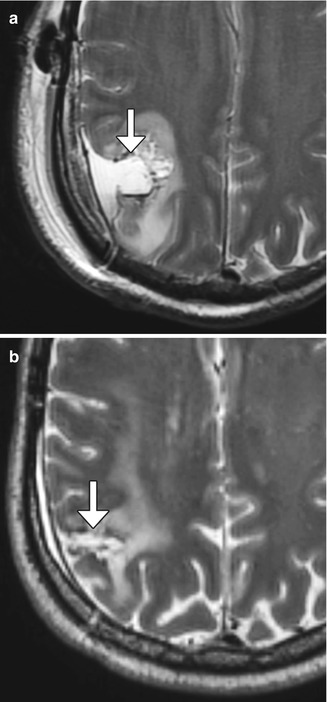
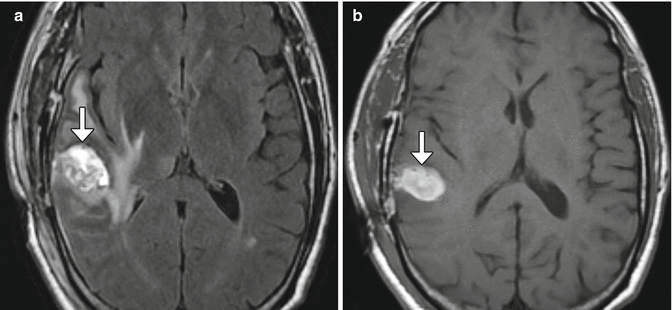
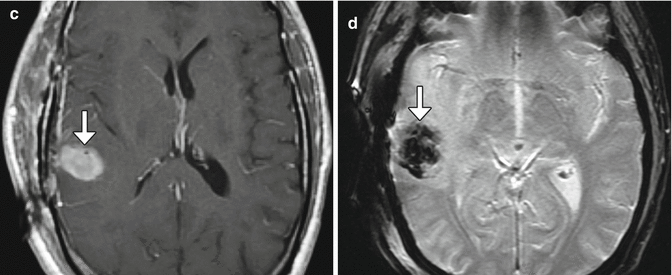
Fig. 5.11
Early surgical cavity with blood products. Axial FLAIR (a), T1-weighted (b), post-contrast T1-weighted (c), and GRE (d) MR images show subacute blood products within a right temporal resection cavity(arrows). There is no significant mass effect or enhancement
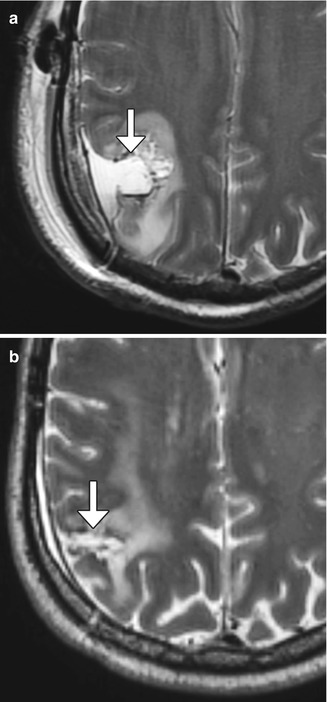
Fig. 5.12
Resection cavity evolution. Initial postoperative axial T2-weighted MRI (a) shows a fluid-filled resection cavity in the right parietal lobe (arrow). Axial T2-weighted MRI obtained 7 months later (b) shows near-complete collapse of the resection cavity (arrow)
Surgically Induced Parenchymal Injury
Local areas of devitalized brain tissue surrounding the resection cavity are encountered on early postoperative MRI in up to 70% of cases of high-grade glioma resection as well as many other tumor resections. This phenomenon manifests as focal areas of restricted diffusion (Fig. 5.13). Enhancement of the devitalized tissue occurs in over 40% of cases between 1 week and several months. Furthermore, this phenomenon can lead to overestimation of residual non-enhancing tumor volume due to the presence of swelling and high signal on T2-weighted sequences during the early postoperative period. Visible encephalomalacia eventually forms around the surgical cavity in over 90% of cases of resection site infarcts. Larger, territorial infarcts are uncommon complications of tumor resection, but are predisposed by proximity to or encasement of major arterial branches and occasionally venous occlusion.
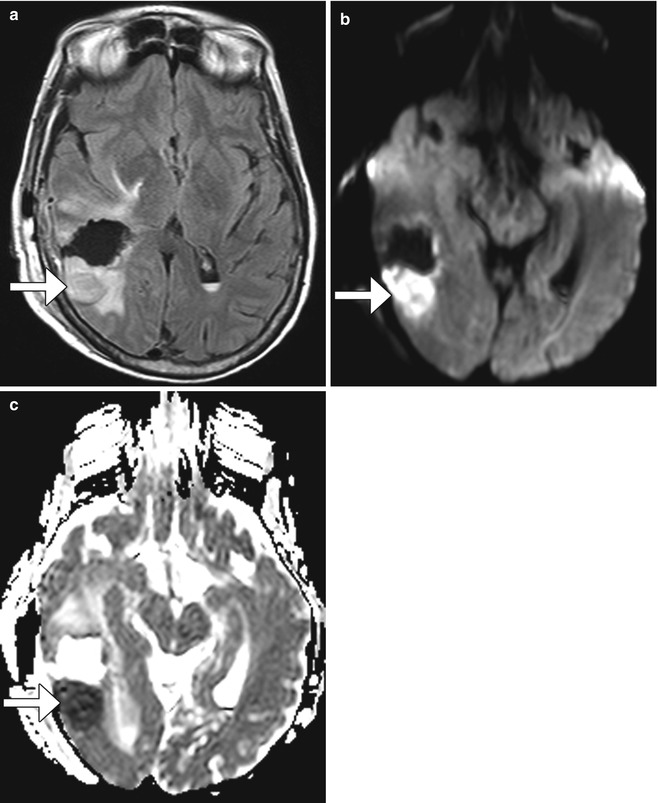
Fig. 5.13
Peri-resection infarction. The patient underwent recent resection of a right posterior temporal lobe glioblastoma. Axial FLAIR (a), DWI (b), and ADC (c) maps show an area of restricted diffusion posterior to the resection cavity (arrows)
Vasogenic edema can result from forceful intraoperative retraction, which is sometimes performed in order to access large or deep tumors. The edema may be related to hyperemia of the brain surface after the release of retraction. On imaging obtained during the early postoperative period, retraction-induced vasogenic edema appears as swelling along the path of the retractors (Fig. 5.14). Unlike acute infarction, the vasogenic edema demonstrates elevated diffusivity rather than restricted diffusion.
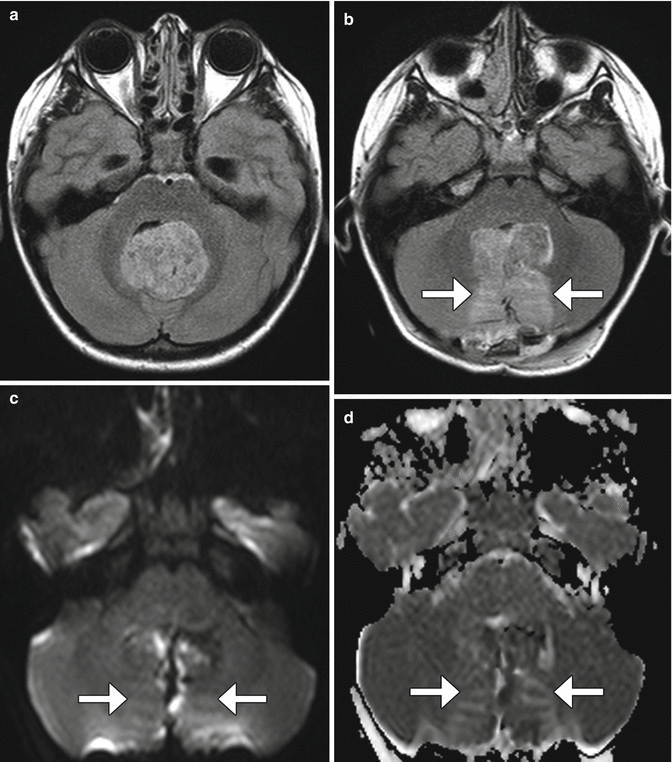
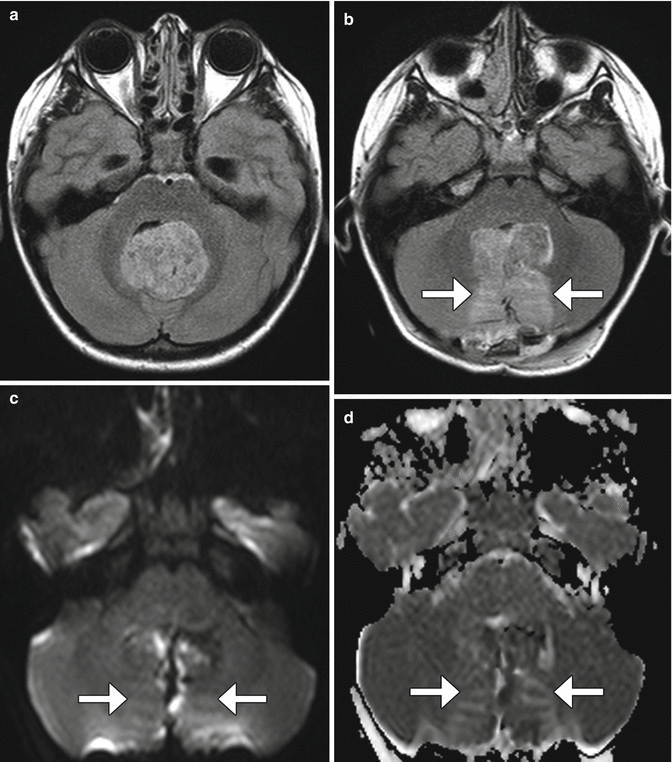
Fig. 5.14
Retraction-induced vasogenic edema. The patient has a history of fourth ventricular medulloblastoma. Preoperative axial FLAIR image (a) shows a large fourth ventricular mass, but no surrounding vasogenic edema. Postoperative FLAIR image (b) shows new areas of hyperintensity in the bilateral medial cerebellar hemispheres. The diffusion-weighted image (c) and ADC map (d) show corresponding mildly elevated diffusivity (arrows). Small areas of ischemia are also present medially
A peculiar complication related to posterior fossa tumor resections is hypertrophic olivary degeneration, which results from disruption of the dentato-rubro-olivary pathway (Guillain-Mollaret triangle). This phenomenon can occur after surgical resection of cerebellar tumors. If the resection site involves the central tegmental tract, the ipsilateral olivary nucleus is affected, while if the superior cerebellar peduncle is involved, the contralateral olivary nucleus is affected. Thus, bilateral hypertrophic olivary degeneration results from disruption of the central tegmental tract and superior cerebellar peduncle. Tongue fasciculations are characteristic of hypertrophic olivary degeneration. On MRI, hypertrophic olivary degeneration manifests as T2 hyperintensity with or without enlargement of the anterolateral medulla (Fig. 5.15). The differential diagnosis includes ischemia, demyelination, tumor spread, and infection. The lack of enhancement with hypertrophic olivary degeneration may help differentiate this entity from the other possibilities, such as some neoplasms. In addition, most cases demonstrate associated atrophy of the contralateral dentate nucleus or cerebellar cortex. Signal changes on MRI develop approximately 1 month after surgery and can persist for many years. Hypertrophy of the olivary nucleus tends to develop after several months and can resolve after 2–3 years.
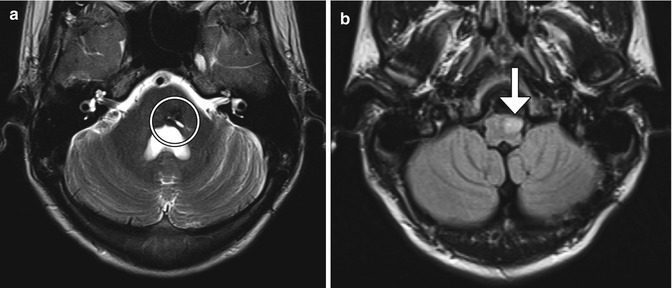
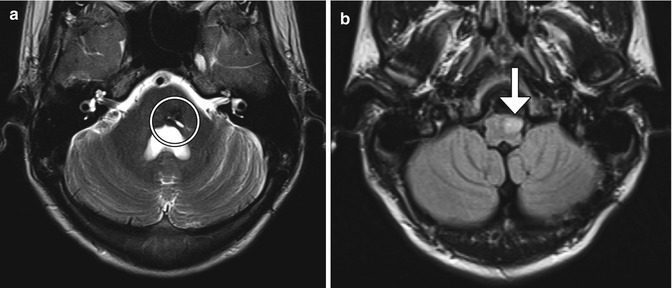
Fig. 5.15
Hypertrophic olivary degeneration. The patient presented with tongue fasciculations after resection of a right pontine cavernous malformation. Axial T2 MRI (a) shows the resection site (encircled). Axial FLAIR MRI (b) shows high signal within an enlarged left olivary nucleus (arrow)
Postoperative Hemorrhagic Lesions
Hemorrhage is a relatively common occurrence with tumor resection and usually occurs at the craniotomy site. CT or MRI can readily depict these hemorrhages. The lack of contrast enhancement and susceptibility effects help distinguish hematomas from residual tumor (Fig. 5.16). Hemorrhage that results from incomplete tumor resection is sometimes termed “wounded tumor syndrome” and is more commonly encountered with vascular tumors, such as melanoma, renal cell carcinoma, and glioblastoma (Fig. 5.17). Other risk factors for postoperative hemorrhage include inadequate hemostasis, underlying coagulopathies, and hypertension.
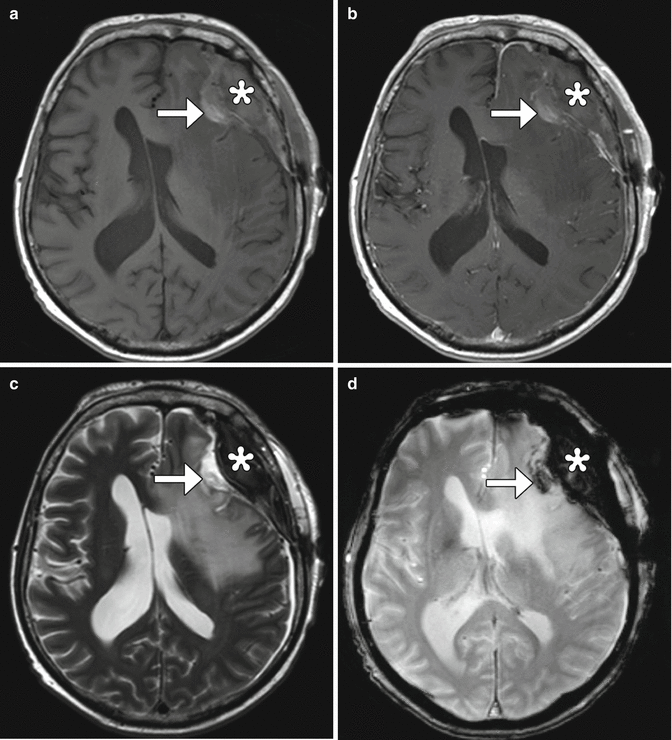
Fig. 5.16
Operative bed hemorrhage. The study was obtained to evaluate for residual tumor following recent meningioma resection. Copious oozing of blood was noted during surgery. Axial T1-weighted (a) and post-contrast T1-weighted (b), T2-weighted (c), and GRE (d) images show an intrinsically T1 hyperintense and T2 hypointense extradural collection (*) with blooming and mass effect upon the underlying brain. There is also a small amount of hemorrhage within the surgical cavity associated with hemostatic material (arrows)
Chronic hemorrhage after surgery can result in superficial siderosis and mainly occurs when there is a cystic cavity that contains friable vessels or residual/recurrent tumor (Fig. 5.18). Hemosiderin deposits can coat remote leptomeningeal surfaces, particularly the cerebellum and brainstem. On CT, superficial siderosis can appear as a mildly hyperattenuating coating of these structures, which may also become atrophic as a result. MRI is more sensitive for depicting hemosiderin deposits, which appear as very low signal intensity on all sequences. Blooming artifact on T2* GRE or SWI sequences accentuates the lesions. The significance of superficial siderosis is that it may cause symptoms such as ataxia and deafness.
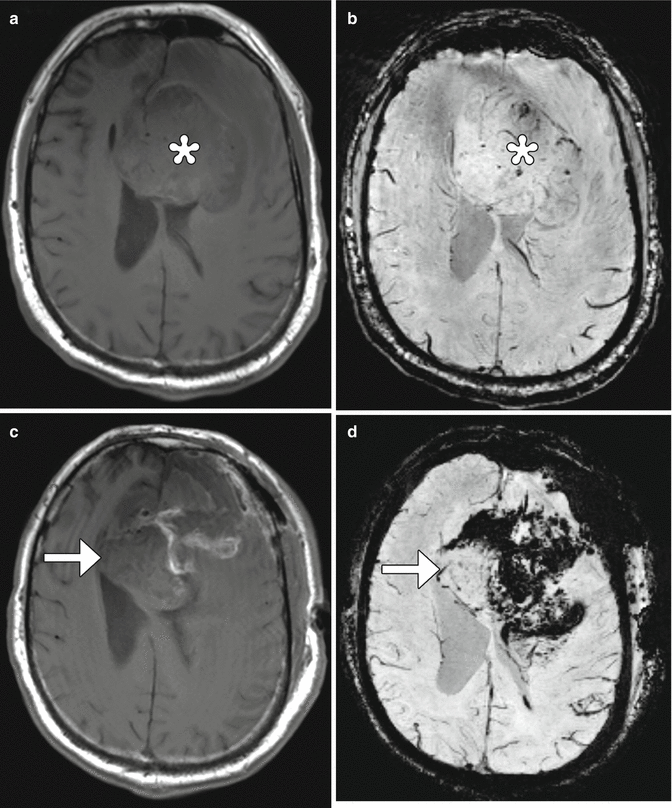
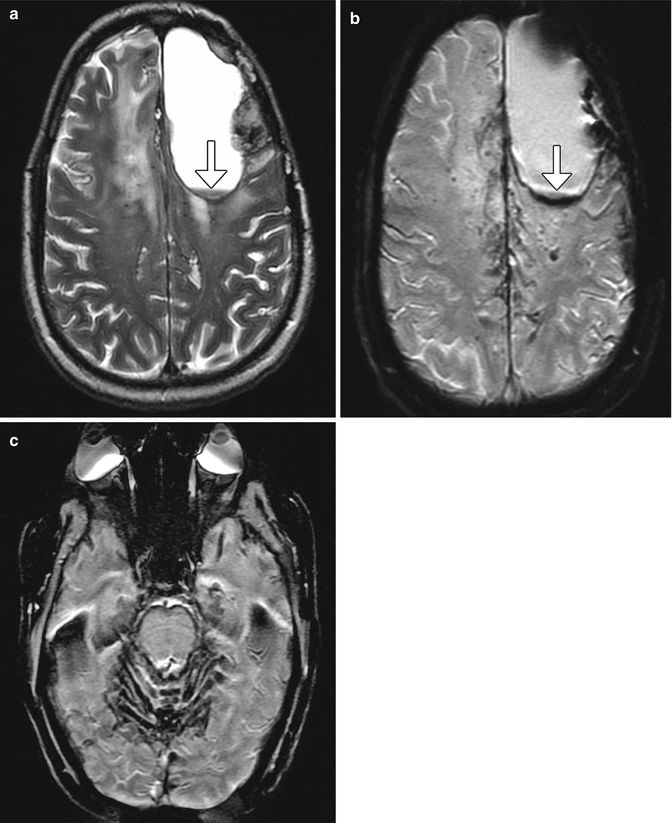
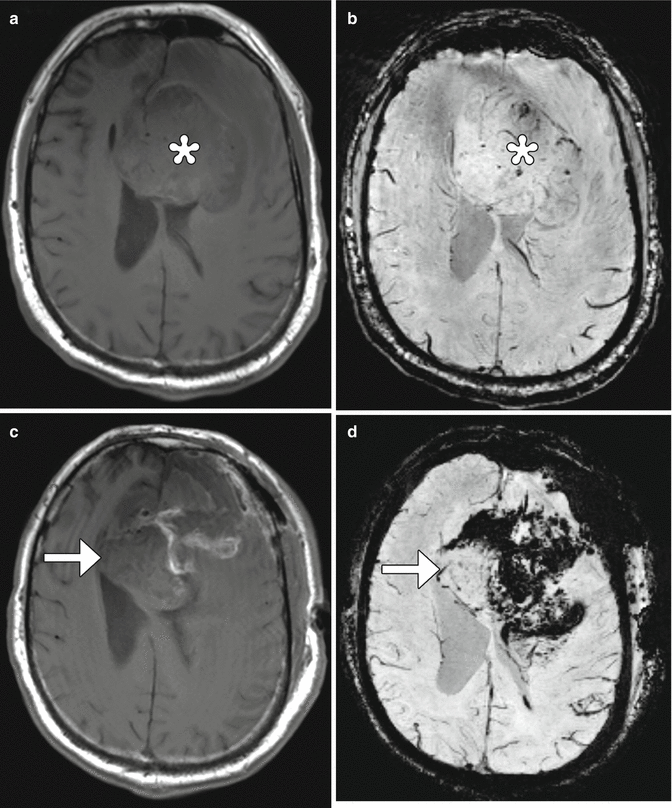
Fig. 5.17
Wounded tumor. The patient underwent subtotal resection of glioblastoma. Preoperative axial T1-weighted (a) and susceptibility-weighted imaging (b) show a large mass (*) in the left frontal lobe with only a few foci of microhemorrhage. Postoperative axial T1-weighted (c) and susceptibility-weighted imaging (d) show interval appearance of high T1 signal hemorrhage and extensive susceptibility effect within and adjacent to the residual tumor (arrows)
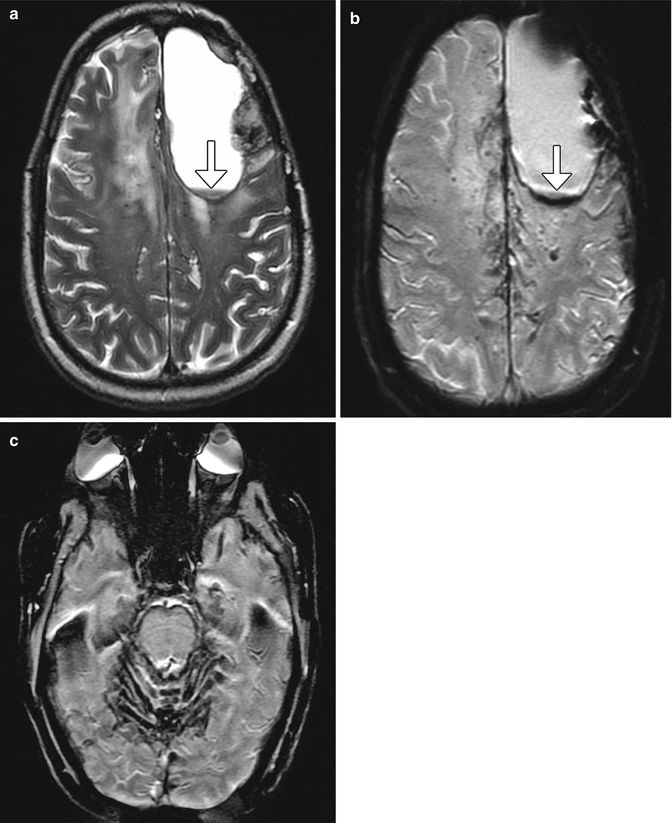
Fig. 5.18
Superficial siderosis. Axial T2-weighted MRI (a) and corresponding SWI (b) show a cystic left frontal lobe resection cavity with layering of blood products (arrows). In addition, there is blooming effect along the margins of the cavity and along the cerebral sulci. SWI at a more inferior level (c) shows extensive susceptibility effect in a leptomeningeal distribution in the brainstem and cerebellum
Enhancing Lesions in the Surgical Bed Region and Beyond
Many types of enhancing lesions can be encountered on imaging after surgery, as listed in Table 5.1 and depicted in Figs. 5.19, 5.20, 5.21, 5.22, and 5.23. Indeed, several of these conditions can coexist and make interpretation of the imaging a challenge. Differentiation of these conditions from recurrent enhancing tumor is based on morphology as well as timing. Advanced imaging techniques, such as perfusion MRI and MR spectroscopy, are often helpful for problem solving. Nevertheless, in some cases, biopsy or serial imaging can help elucidate ambiguous cases. It is also important to systematically evaluate the areas beyond the surgical bed on imaging exams, particularly with aggressive neoplasms, such as glioblastoma, which can undergo spread to remote parts of the brain, seed the scalp and face soft tissues, and undergo cerebrospinal fluid dissemination.
Table 5.1
Differential diagnosis of enhancing lesions on MRI after treatment for malignant glioma (Courtesy John W. Henson, MD and Jennifer Wulff, ARNP)
Condition | Onset | Other features |
---|---|---|
Granulation tissue | First postoperative week (usually after 2 or 3 days), intensifies over the ensuing weeks, and resolves over 3–5 months | The enhancement is typically linear and smooth, but can become more nodular by 1 week following surgery. Since residual enhancing tumor can be obscured or confounded by granulation tissue, baseline imaging is recommended within 48 h of surgery, before granulation tissue forms. Serial imaging can also help to differentiate granulation tissue from residual tumor in that tumor increases in size over time, while granulation tissue should remain stable and eventually resolves |
Perioperative ischemia | 2 weeks after surgery | Two-thirds of patients have focal infarcts around the resection cavity, and this can account for new post-op neurological deficits. Look for this on immediate post-op DWI. Can enhance after 10–14 days. Enhancement slowly resolves, leaving an area of encephalomalacia |
Postoperative infection | 1–3 weeks after surgery | Clinical deterioration and new enhancement 1–3 weeks after surgery should raise a question of infection. Wound breakdown and drainage, markedly tender wound, fever, and elevated ESR can occur. Focal infection may show restricted diffusion |
Pseudoprogression | Within 3 months following completion of concomitant RT and TMZ | Inflammatory response to treatment. Often symptomatic. Occurs within the RT port. Cannot be distinguished from true progression by either routine MRI or advanced* MRI or FDG-PET. More likely in glioblastoma with methylated MGMT promoter. Wanes with time (scans are performed every month until change determines likely diagnosis). Good prognostic factor |
True progression | Any time following surgery | Worsens with time. Routine MRI cannot distinguish from pseudoprogression and radiation necrosis, but tumor tends to have elevated blood volume on perfusion MRI. More likely in tumors without methylation of the MGMT promoter. Poor prognostic factor |
Radiation necrosis | Usually >1 year after radiation therapy | Routine MRI cannot distinguish from progression; advanced MRI and FDG-PET can be very useful in distinguishing from progression. Can progress or wane over time. SMART (stroke-like migraines after radiation therapy) syndrome is an unusual, late complication of localized radiation therapy for brain tumors, in which patients present with headache and neurological deficits between about 2 and 10 years after treatment, usually greater than 50 Gy of radiation. Treated with observation, steroids, bevacizumab, or surgery |
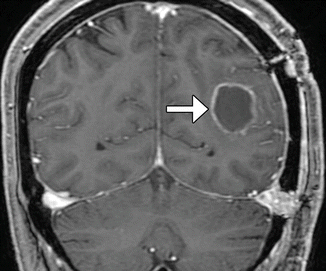
Fig. 5.19
Granulation tissue. The patient is status post meningioma resection 5 days prior to imaging. Coronal post-contrast T1-weighted MRI shows a thin circumferential rim of enhancement along the resection margin (arrow)
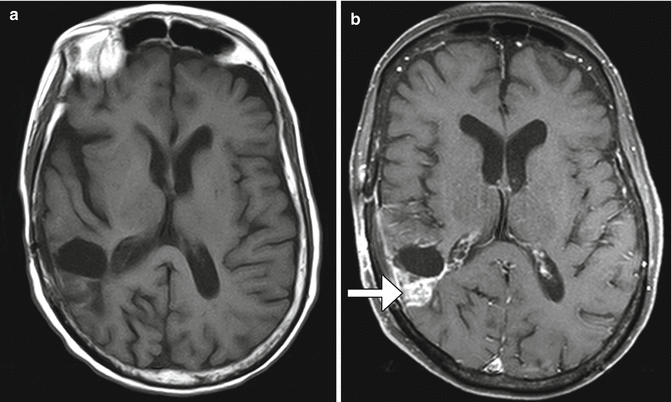
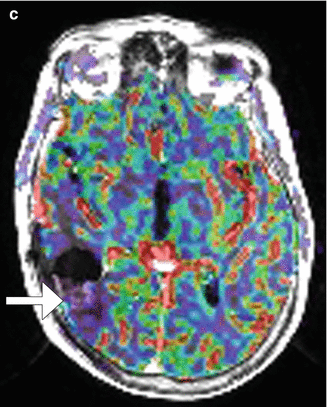
Fig. 5.20
Perioperative infarct. Pre- (a) and post-contrast (b) T1-weighted MR images obtained 1 month after surgery in the same case as in Fig. 5.13 show that the infarcted tissues enhance (arrow). Furthermore, the CBV map (c) shows corresponding hypoperfusion in the area (arrow)
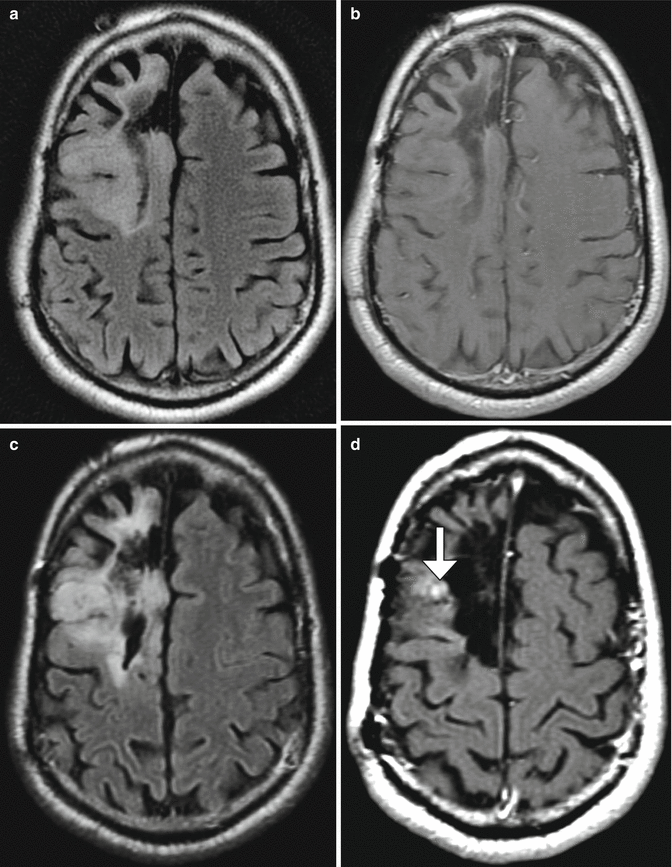
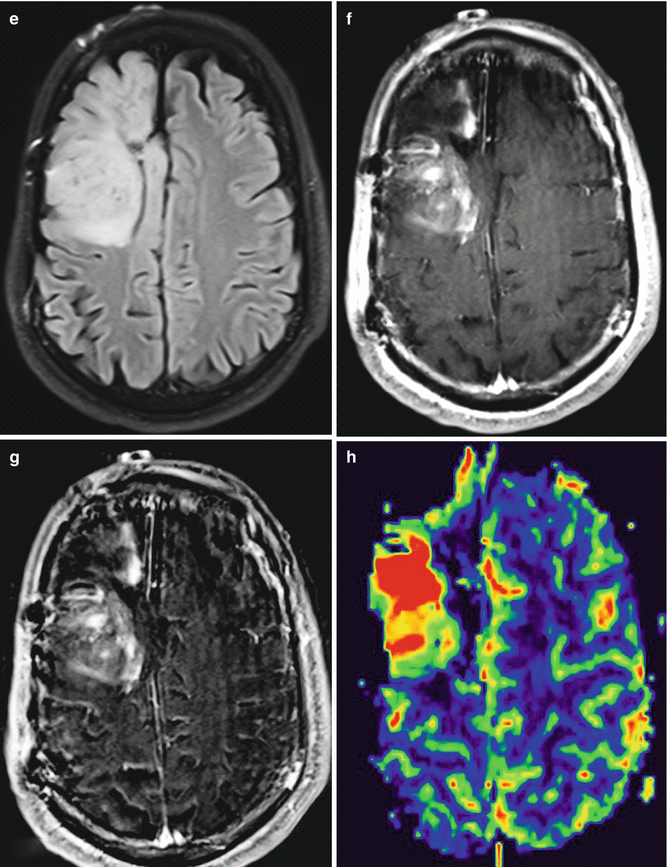
Fig. 5.21
Tumor progression. The patient underwent gross total resection of an oligoastrocytoma (WHO grade II/IV) in the right frontal lobe. Axial FLAIR (a) and post-contrast T1-weighted (b) MR images obtained approximately 10 years after resection show a right frontal resection cavity surrounded by non-enhancing FLAIR signal abnormality. Axial FLAIR (c) and post-contrast T1-weighted (d) MR images obtained approximately 1 year later show a new focus of enhancement adjacent to the resection cavity (arrow), but no obvious change in the FLAIR signal abnormality. Axial FLAIR (e), post-contrast T1-weighted (f), subtraction image (g), and CBV map (h) obtained approximately 6 months later demonstrate marked interval increase in volume of the FLAIR signal abnormality and enhancing adjacent to the resection cavity and associated elevated CBC (arrows)
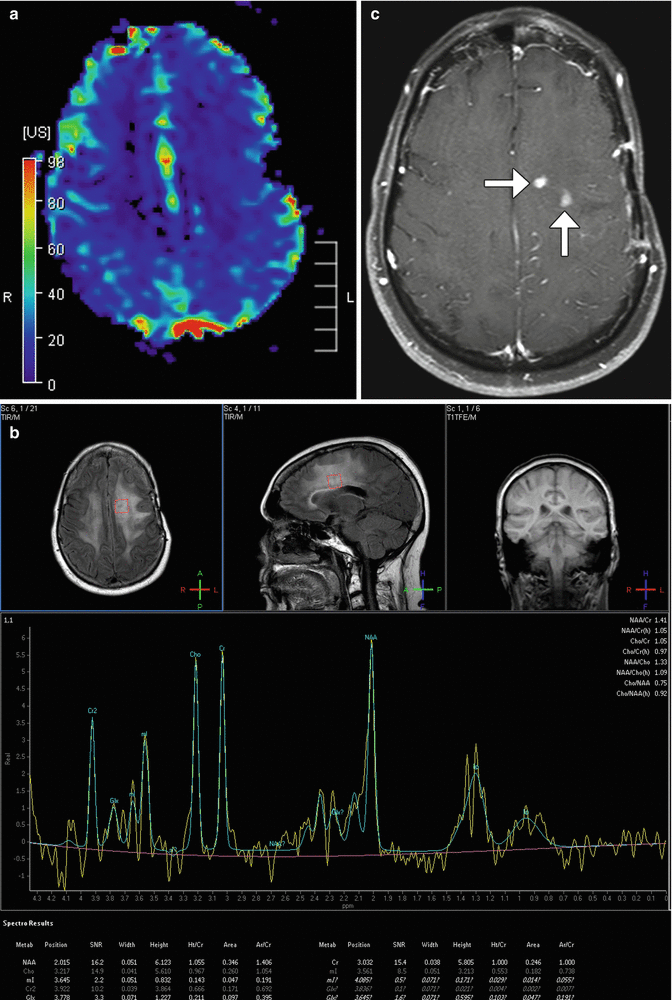
Fig. 5.22
Radiation necrosis. The patient has a history of left frontal lobe glioblastoma that was resected and radiated approximately 1 year before. Axial (a) post-contrast T1-weighted MRI shows small areas of enhancement in the treatment bed region (arrows). There is no corresponding hypermetabolism on the blood volume map (b). MRI spectroscopy (c) over the abnormality shows a lactate peak, mildly reduced NAA peak, and a Cho peak that is not particularly elevated with respect to Cr
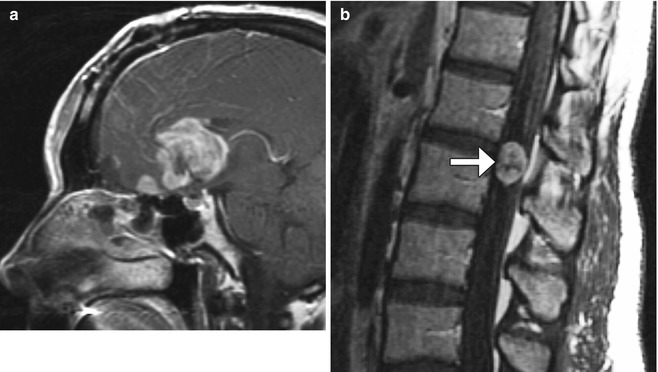
Fig. 5.23
Metastatic glioblastoma in the spinal canal. The patient presented with back and low extremity pain after gross total resection of a left frontal glioblastoma resection with recurrence. Sagittal post-contrast T1-weighted MRI (a) shows irregular enhancement involving the bilateral frontal lobes, extending to the meningeal surface. Sagittal post-contrast T1-weighted MRI (b) shows an intradural, extramedullary mass with irregular enhancement in the upper lumbar spinal canal (arrow)
5.2.3 Ommaya Reservoirs
5.2.3.1 Discussion
Intrathecal chemotherapy can lengthen survival and alleviate symptoms in patients with widespread leptomeningeal metastases. The two primary means of delivering intrathecal chemotherapy are Ommaya reservoirs and repeat lumbar puncture. Ommaya reservoirs are implanted in the subcutaneous tissues of the scalp and contain a pump mechanism for drug delivery agents into the ventricular system through an intraventricular catheter (Fig. 5.24). Ommaya reservoirs offer many advantages over repeat lumbar punctures, including greater patient comfort, diminished risk for patients with thrombocytopenia, more consistent drug levels, and possibly greater clinical efficacy. Tumor cyst devices are similar to Ommaya shunts, but are used to inject chemotherapeutic agents directly into tumors.
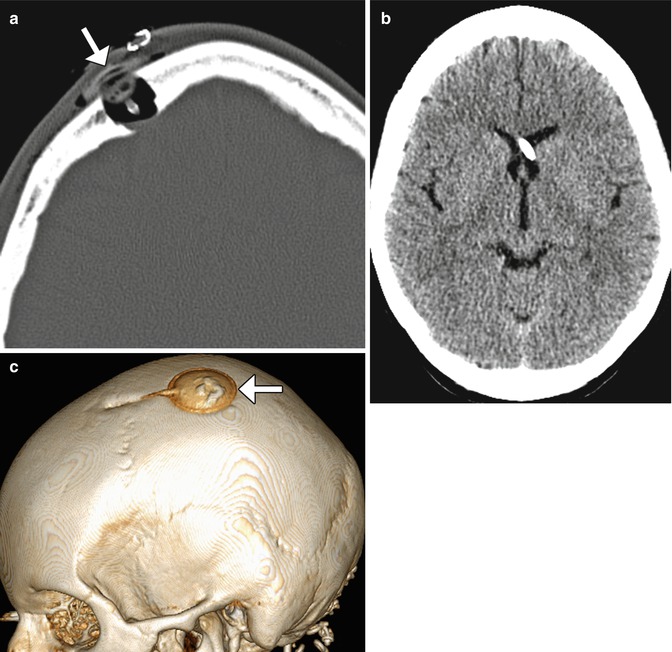
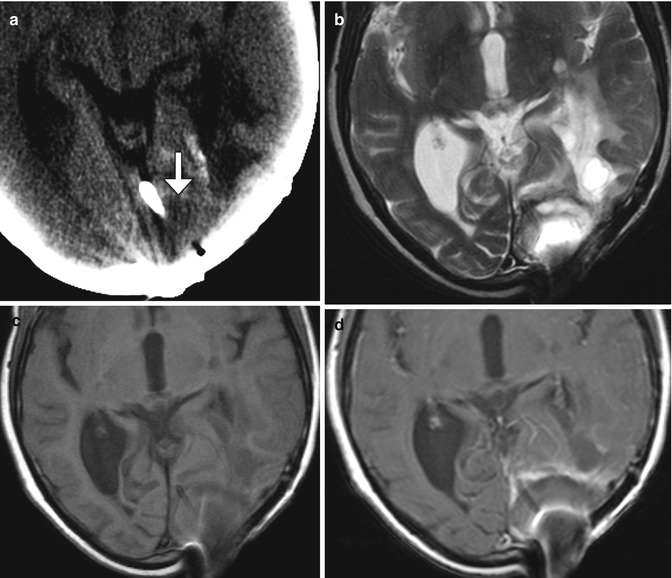
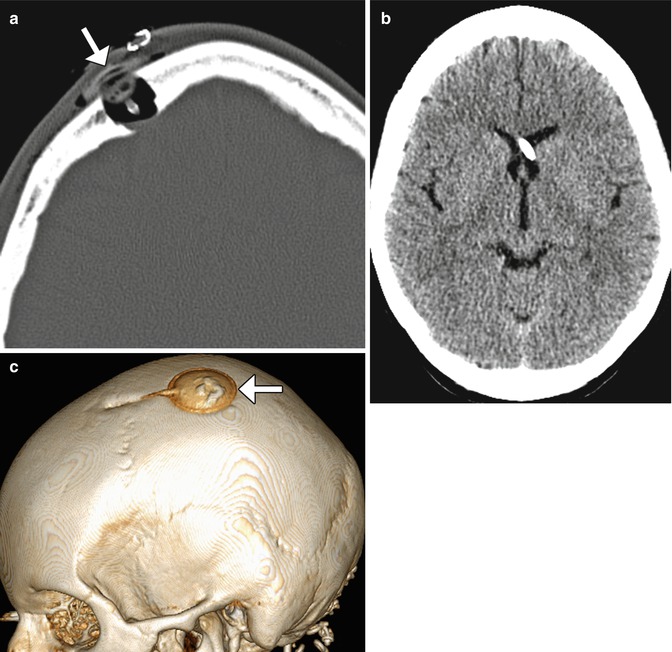
Fig. 5.24
Ommaya reservoir components. The patient has a history of leptomeningeal spread of breast cancer. Axial CT images show the Ommaya reservoir (arrow) positioned in the right frontal subcutaneous tissues (a). The drug delivery catheter enters the intracranial compartment via a burr hole. The tip of the catheter lies within the anterior horn of the left lateral ventricle (b). 3D CT image (c) shows the reservoir (arrows) and catheter entering the skull through a burr hole
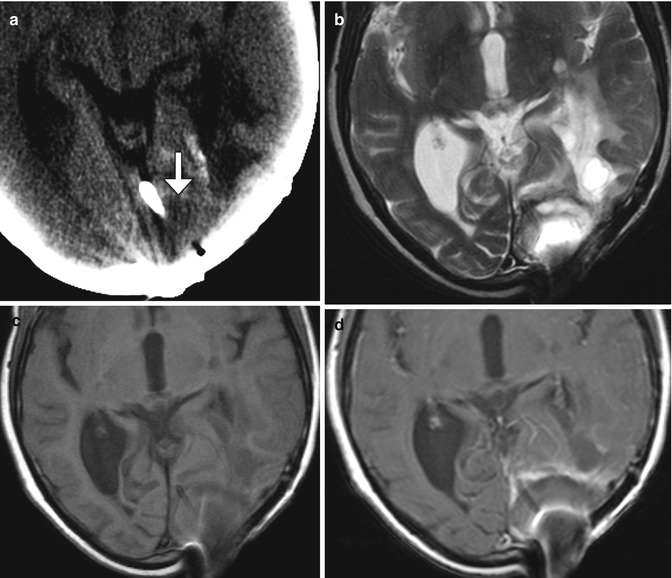
Fig. 5.25
Ommaya catheter infection. The patient presented with exposed Ommaya reservoir hardware and cellulitis. Axial CT image (a) shows a left parietal Ommaya catheter surrounded by a fluid collection (arrow), which is difficult to discern amidst streak artifact. Axial T2-weighted (b), T1-weighted (c), and post-contrast T1-weighted (d) MR images show a complex fluid collection with rim enhancement surrounding the Ommaya catheter, compatible with a pericatheter abscess
Infection is a major complication of Ommaya catheter placement. The incidence of Ommaya-associated infection is 15% within the first year of placement (range 2–23%). Staphylococcus aureus and Staphylococcus epidermidis are the most common causative organisms. Manifestations of catheter-associated infection range from meningitis to abscess, for which imaging is useful for identifying fluid collections surrounding the catheter (Fig. 5.25). Debris in the fluid and enhancement helps differentiate infection from hygromas (simple fluid collections), which can also occur with Ommaya catheter placement. Management consists of antibiotic therapy and possible hardware removal and debridement depending on the extent of the infection.
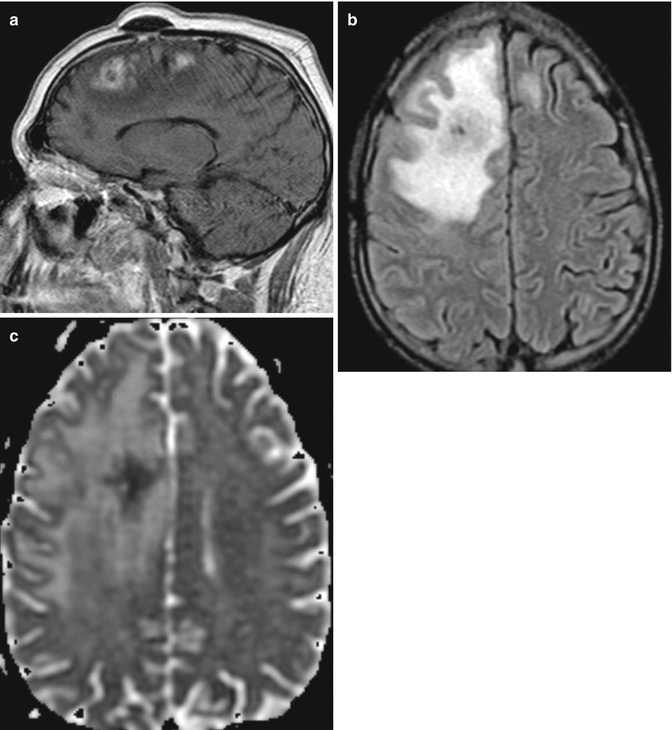
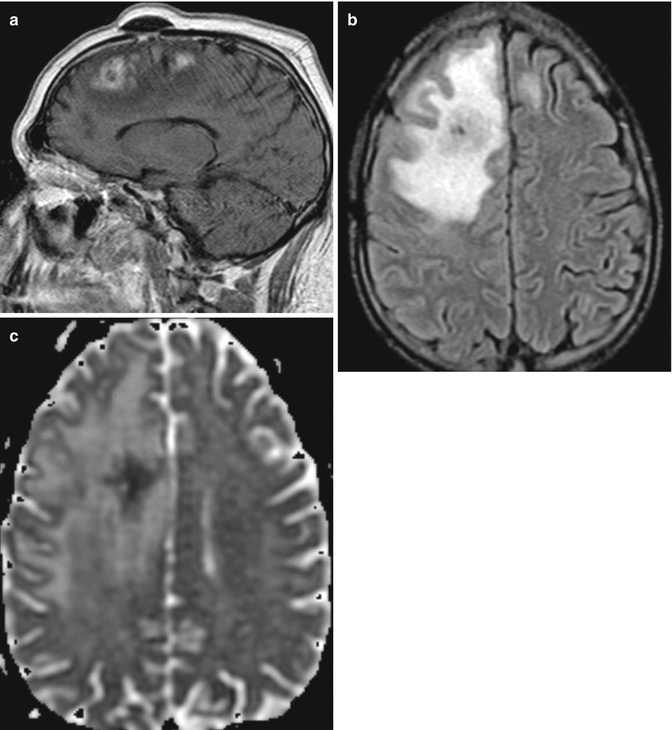
Fig. 5.26
Extravasation of methotrexate through blocked Ommaya reservoir with focal brain necrosis. Post-contrast sagittal T1-weighted MRI (a) shows edema and patchy enhancement surrounding the catheter. Axial FLAIR (c) better delineates the extent edema surrounding the Ommaya catheter, and the corresponding ADC map (c) shows restricted diffusion surrounding the path of the Ommaya catheter, consistent with cytotoxic edema
Focal brain necrosis due to chemotherapy extravasation secondary to Ommaya reservoir catheter obstruction is rare, with an incidence of 0.6% of patients. This condition is caused by displacement of the catheter tip into the brain parenchyma. Imaging demonstrates circumferential areas of necrosis surrounding the retracted Ommaya catheter, manifesting as patchy enhancement, high T2 signal, and restricted diffusion, representing cytotoxic edema (Fig. 5.26). A unique and serious complication of methotrexate extravasation is progressive leukoencephalopathy. This entity involves the white matter diffusely and can be either hemorrhagic or nonhemorrhagic.
Cerebrospinal fluid cysts can sometimes form around Ommaya catheters and may be caused by distal shunt obstruction, although this complication can also occur when the catheter is appropriately positioned, with or without hydrocephalus. The pericatheter cysts do not have perceptible walls or rim enhancement, but may have surrounding edema. Although the cysts may be asymptomatic, it is important to evaluate for predisposing factors that could be addressed, such as malpositioning of the Ommaya catheter (Fig. 5.27).
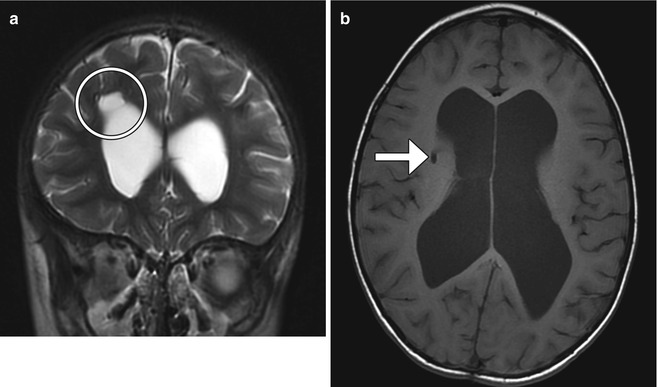
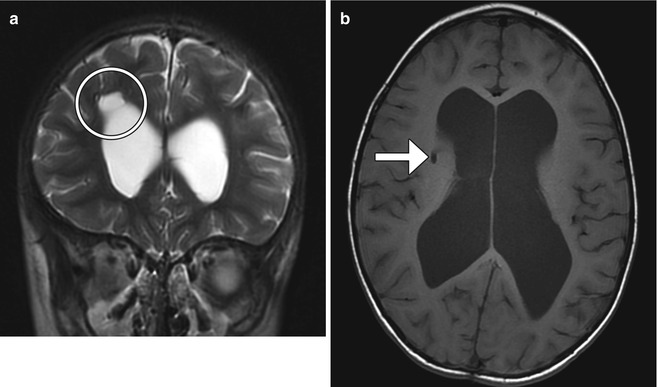
Fig. 5.27
Ommaya catheter-associated cyst and catheter malpositioning (a) shows a right frontal lobe periventricular cerebrospinal fluid cyst (encircled). Axial T1-weighted MRI (b) shows the catheter (arrow) has penetrated the right basal ganglia instead of the lateral ventricle. There is also hydrocephalus
5.2.4 Chemotherapy Wafers
5.2.4.1 Discussion
Chemotherapy wafers, such as carmustine implants (Gliadel), are sometimes implanted in the surgical bed after malignant brain neoplasm resection. The wafers are biodegradable sheets of polymers that are impregnated with the chemotherapy agent. Initially, the wafers appear as hypointense linear structures on T1- and T2-weighted MRI sequences, but they can change in signal intensity characteristics over time (Fig. 5.28).
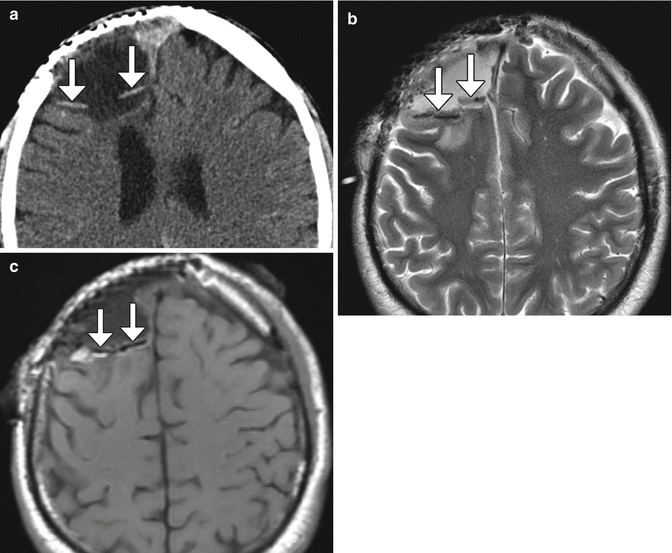
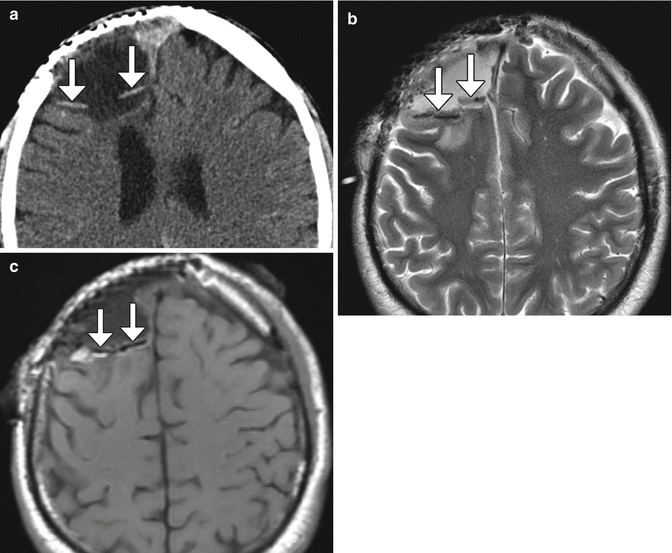
Fig. 5.28
Chemotherapy wafers. The patient has a history of glioblastoma status post resection and implantation of Gliadel wafers. Axial CT (a) shows hyperattenuating linear structures along the edges of the right frontal resection cavity (arrows). T2-weighted (b) and axial T1-weighted (c) MR images obtained 1 day after surgery demonstrate the low signal linear Gliadel wafers (arrows) lining the resection cavity
The presence of wafers does not alter the pattern of tumor recurrence. Perfusion MRI is particularly useful to monitor the treatment effects and differentiate these from recurrent neoplasm. The presence of foci with elevated CBV suggests tumor recurrence. MR spectroscopy can also be useful for monitoring tumor response to chemotherapy wafers. For example, it has been noted that increased peritumoral NAA/Cr and decreased peritumoral Cho/NAA compared with normal brain tissue by 3–5 weeks suggest treatment response.
5.2.5 Brachytherapy Seeds
5.2.5.1 Discussion
Local radiation therapy can be administered for treatment of brain tumors via brachytherapy (interstitial) seed implantation. The seeds contain radioactive isotopes, such as I-125, and can be implanted temporarily (for approximately 1 week) or permanently. Temporary seeds are typically introduced and removed using plastic catheters passed through burr holes, while permanent seeds are implanted in the tumor/surgical bed. The seeds appear as tiny metallic cylinders on CT or signal voids on MRI (Fig. 5.29).
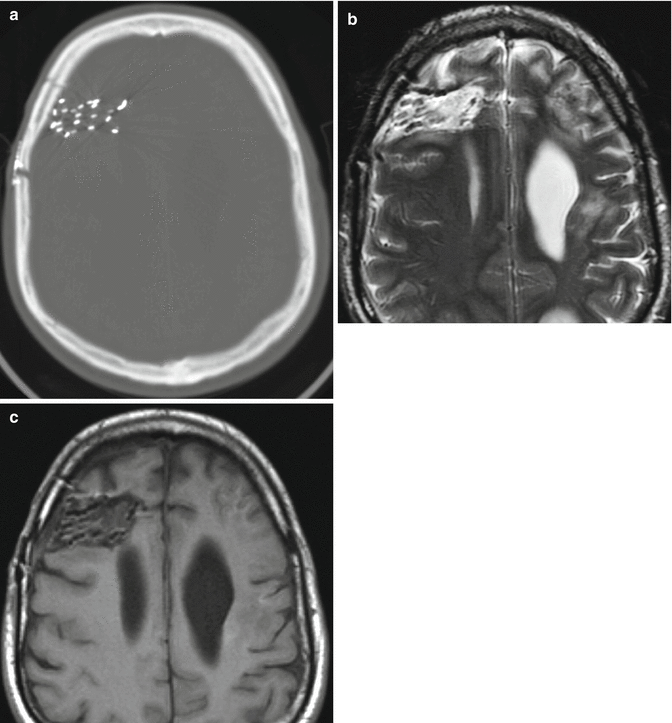
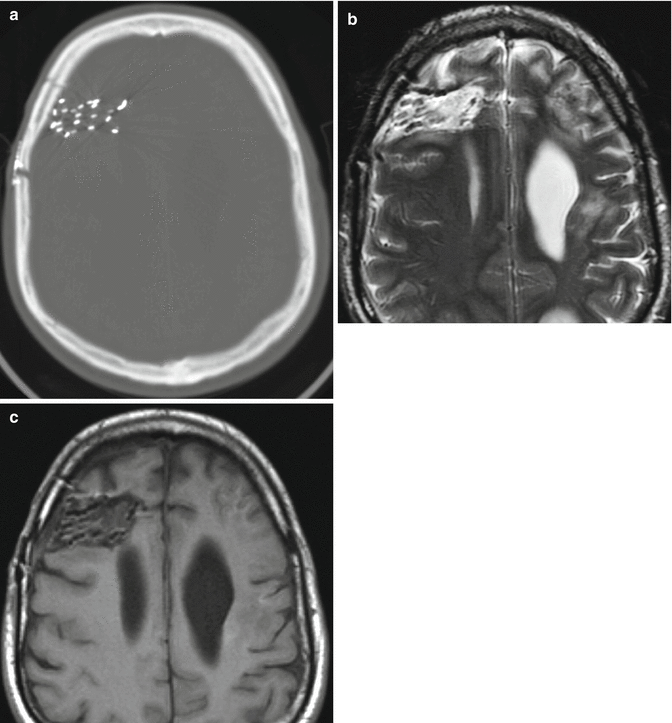
Fig. 5.29
Brachytherapy seeds. The patient has a history of metastatic sarcoma to the right frontal lobe and is status post right frontal craniotomy, gross total tumor resection, and placement of I-125 interstitial radiation seeds. Axial CT image (a) demonstrates numerous metallic interstitial seeds each measuring a few millimeters in length within the surgical cavity. On both T2-weighted (b) and T1-weighted (c) MRI, the seeds are of low signal intensity
5.2.6 GliaSite Radiation Therapy System
5.2.6.1 Discussion
The GliaSite radiation therapy system is used to administer intracranial brachytherapy for brain tumor treatment. The system is a catheter-based device that consists of an infusion port on one end and a double balloon on the other. Positioning markers are also included along the length of the catheter. The balloon, which contains the radioactive isotope solution, is positioned within the surgical cavity. The filled balloon is hyperattenuating on CT and displays fluid signal on MRI (Fig. 5.30). Normally, there can be enhancement in the tissues surrounding the balloon. The catheter and its position markers are also visible on both modalities. However, the balloon and surrounding tissues are better assessed on MRI, particularly when there is tumor recurrence. Perfusion MRI is especially helpful for evaluating enhancing lesions in the tumor bed, whereby elevated rCBV suggests recurrence of high-grade tumor.
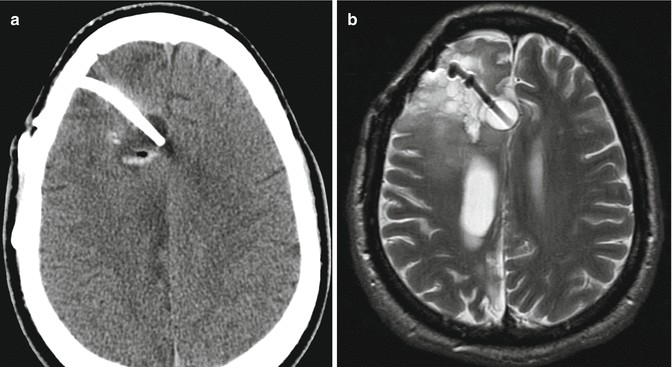
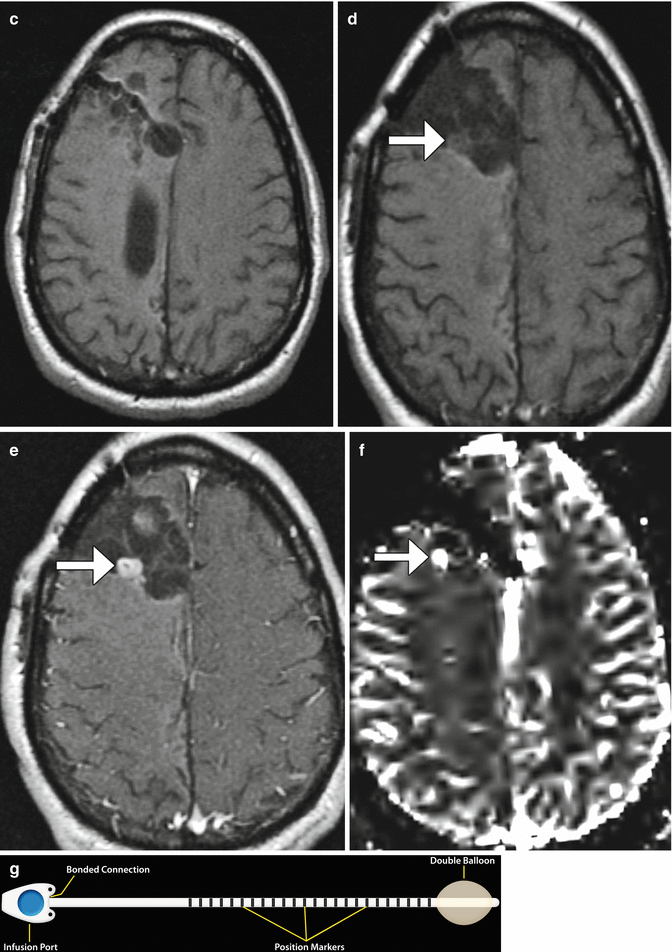
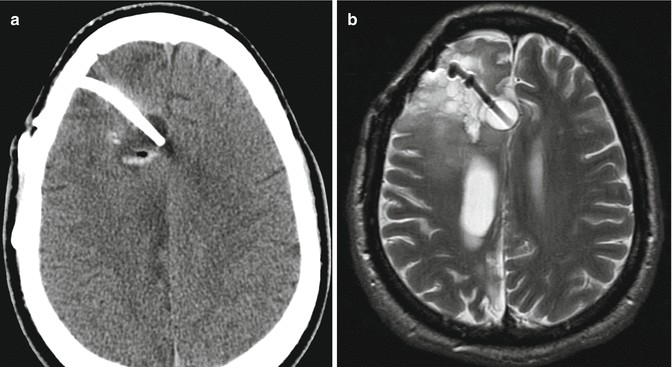
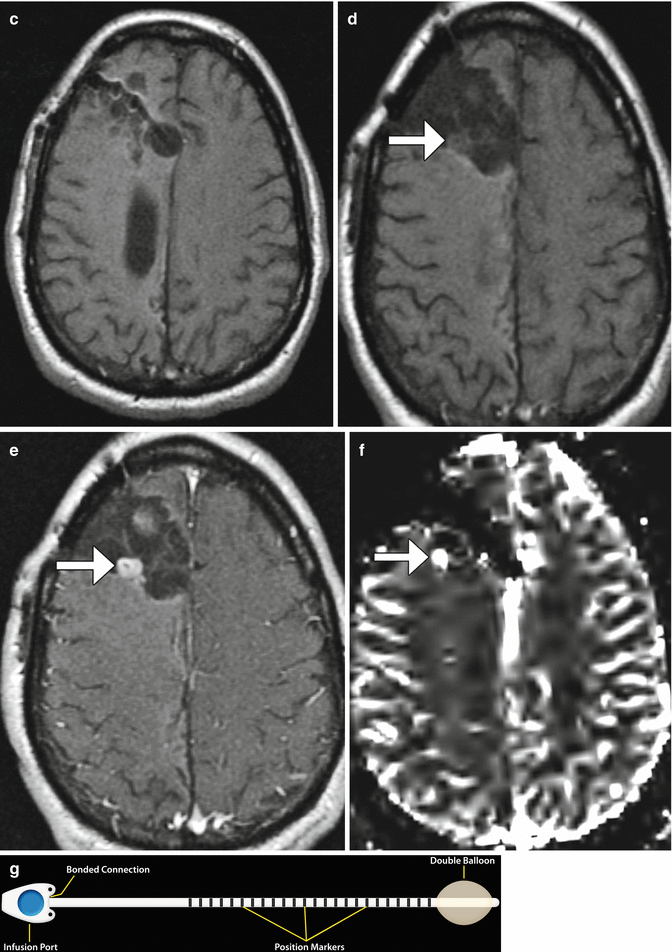
Fig. 5.30
GliaSite system. The patient has a history of right frontal glioblastoma, status post resection. Axial CT (a) and axial T2-weighted (b) and T1-weighted (c) MR images that show the fluid-filled GliaSite radiation therapy system balloon at the end of the low signal intensity catheter with positioning markers. Pre- (d) and post-contrast (e) T1-weighted images and CBV map (f) obtained 1 year later show an enhancing nodule with corresponding increased perfusion adjacent to the catheter in the surgical bed, consistent with recurrent tumor (arrows). Illustration of the GliaSite Radiation Therapy system (g)
5.3 Neurodegenerative, Neuropsychiatric, and Epilepsy Surgery
5.3.1 Prefrontal Lobotomy
5.3.1.1 Discussion
Prefrontal lobotomy (leucotomy) is a now obsolete procedure that was introduced in 1935 as a treatment option for psychiatric illnesses, such as schizophrenia. The procedure essentially consists of ablating the frontal lobe white matter tracts using a probe-like device known as the leukotome via a transorbital or transcranial approach. This produces the appearance of band-like cavitary lesions in the frontal lobe white matter (Fig. 5.31). On MRI, FLAIR sequences show a hyperintense rim of gliosis surrounding the cavitary defects. Focal atrophy of the frontal lobe and corpus callosum is common and often pronounced. High-attenuation foci on CT and susceptibility effects on MRI can be observed along the lobotomy margins, which correspond to residual Pantopaque used for visualization of the lobotomy plane during the operation.
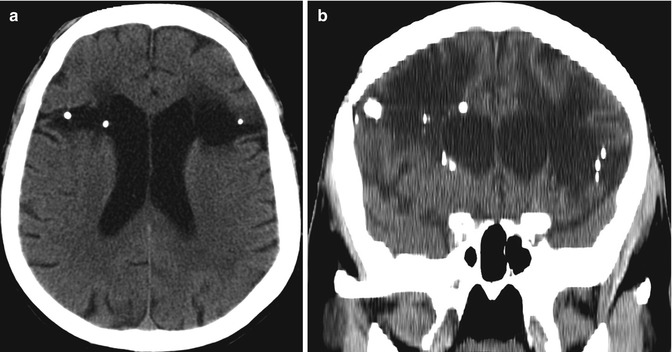
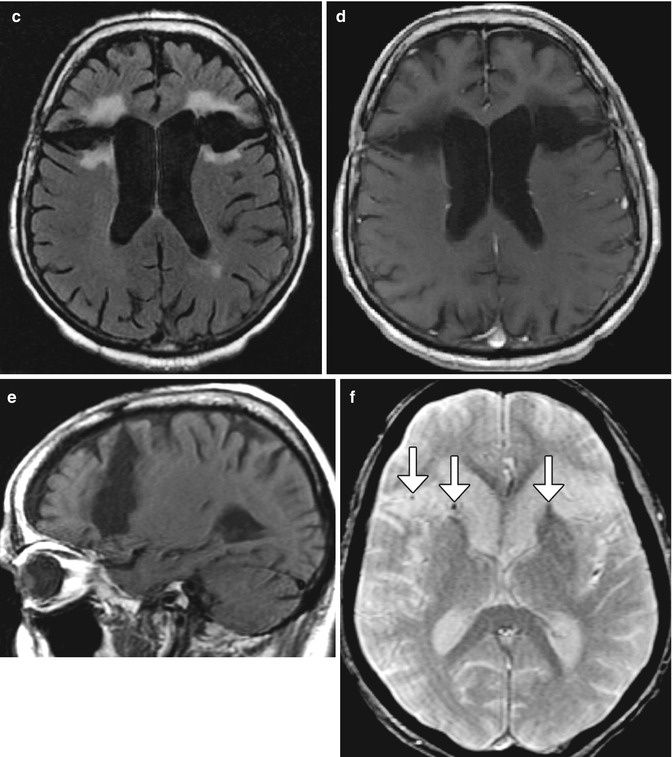
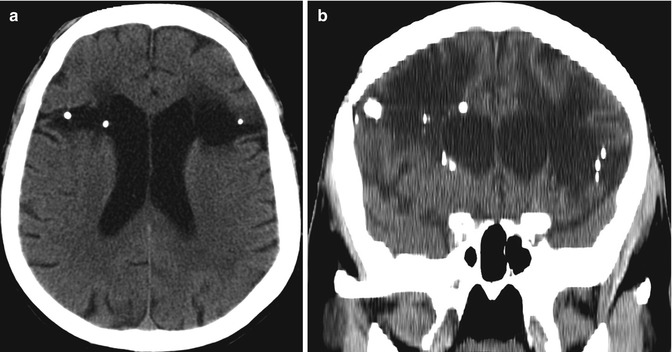
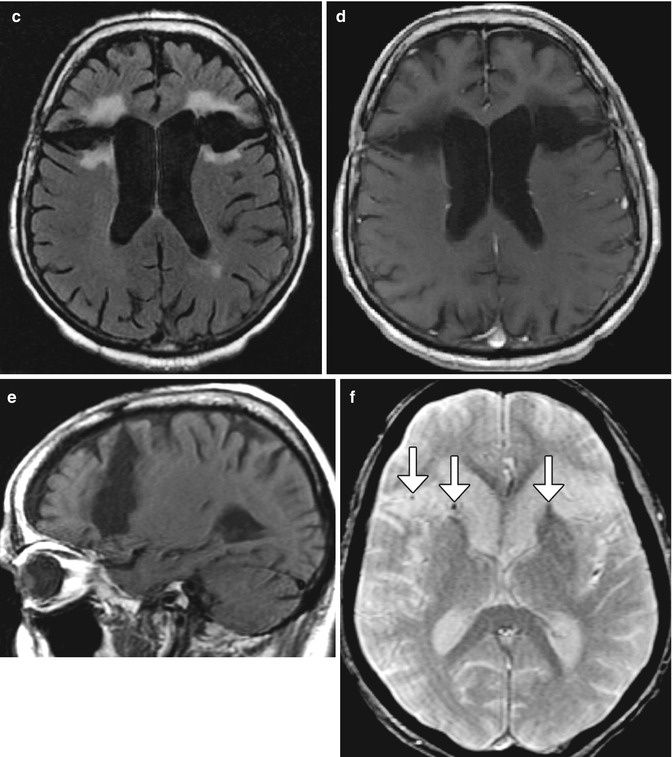
Fig. 5.31
Bilateral prefrontal lobotomy. The patient has a history of schizophrenia treated with bifrontal lobotomy many years before. Axial (a) and coronal (b) CT images show low-attenuation defects in the bilateral frontal lobe white matter. There are scattered punctate hyperattenuating foci in the surgical defects bilaterally, consistent with Pantopaque. There is also disproportionate enlargement of the bilateral frontal lobe sulci. Axial FLAIR (c), axial T1-weighted (d), and sagittal T1-weighted (e) MR images demonstrate linear cystic defects in the bilateral frontal lobes with surrounding white matter signal abnormality, consistent with gliosis. Axial GRE (f) shows small foci of susceptibility, which correspond to residual deposits of Pantopaque (arrows)
5.3.2 Pallidotomy
5.3.2.1 Discussion
Pallidotomy is a procedure that can be performed in Parkinson’s disease patients who do not experience adequate symptom relief from medical therapy. The surgery consists of introducing probes via frontal burr holes for ablation of the posteroventral portion of the globus pallidus interna (Fig. 5.32). The goal of the procedure is to interrupt excessive inhibitory output from the basal ganglia. On CT, the pallidotomy lesions appear as hypoattenuating foci of encephalomalacia that become more pronounced over time. On MRI, acute pallidotomy lesions are usually hyperintense centrally on T1 and hypointense centrally on T2 due to hemorrhage surrounded by a rim of T2 hyperintensity and hypointensity on T1 and GRE, which represents edema. Restricted diffusion due to focal cytotoxic edema can also be encountered. Eventually, the lesion-edema complex evolves into a smaller focus of low T1 signal and high T2 signal. Lesion sizes can be variable depending upon technique implemented.
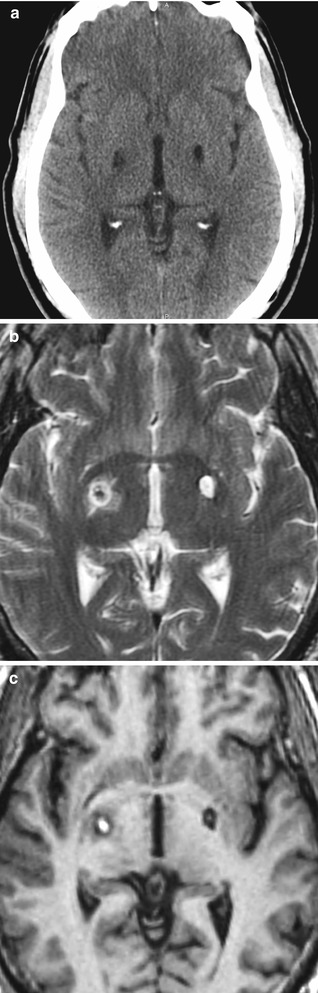
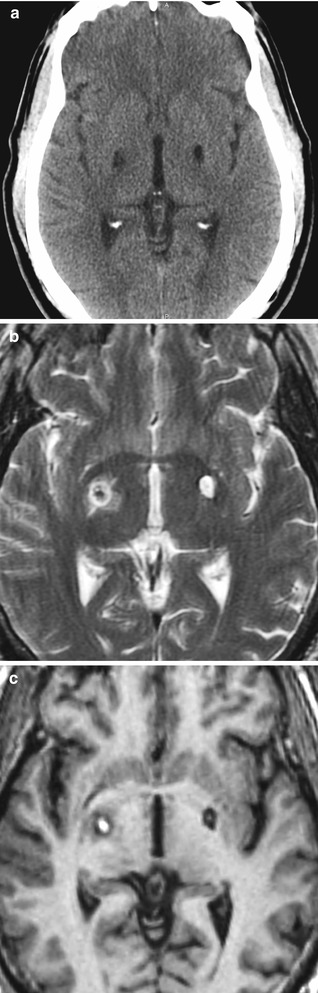
Fig. 5.32
Pallidotomy. The patient has a history of Parkinson’s disease and underwent pallidotomy approximately 1 year prior to imaging on the left side and several days earlier on the right side. Axial CT image (a) shows hypoattenuating foci in the bilateral globus pallidi. The lesion on the right is more recent and slightly less hypoattenuating than the lesion on the left. Axial T2-weighted (b) and axial T1-weighted (c) images show subacute blood products within the right pallidotomy lesion surrounded by edema and fluid within the chronic left pallidotomy lesion
5.3.3 Cingulotomy
5.3.3.1 Discussion
Cingulotomy is a form of psychosurgery that is used to treat conditions, such as intractable obsessive-compulsive disorder. The procedure can be performed in a minimally invasive manner via thermal ablation. This process results in necrosis of the surrounding brain tissue, which appears as concentric rings of signal abnormality (Fig. 5.33). There can be T1 hyperintensity due to petechial hemorrhage, as well as T2 hyperintensity from edema and restricted diffusion due to ischemia during the early postoperative period, which then evolves over time. Diffusion tensor imaging is also useful for confirming interruption of the cingulum. In particular, the dorsolateral region of the cingulotomy lesion is associated with improved behavior.
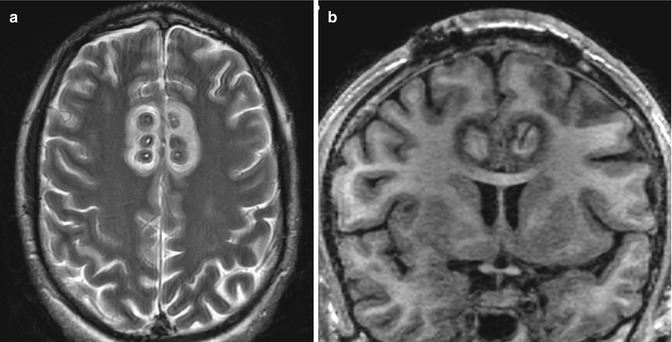
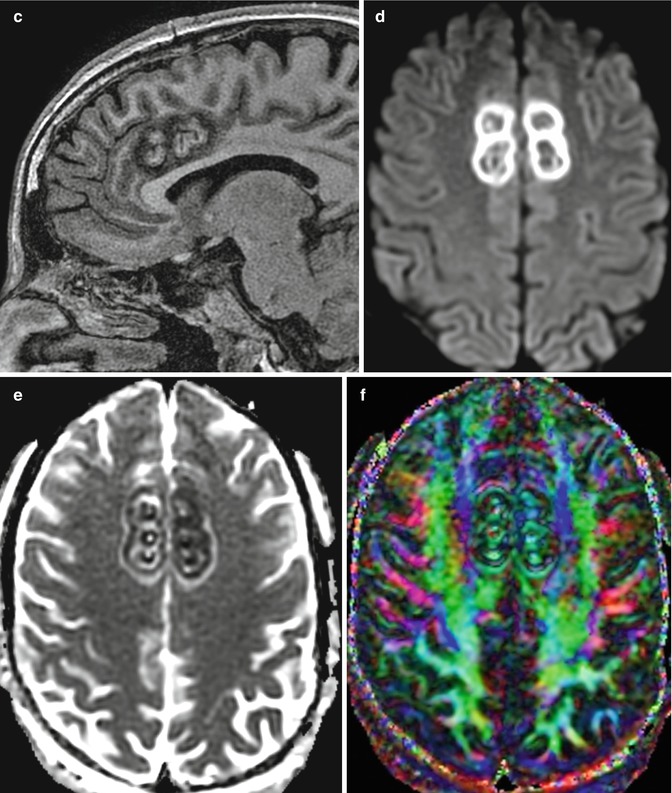
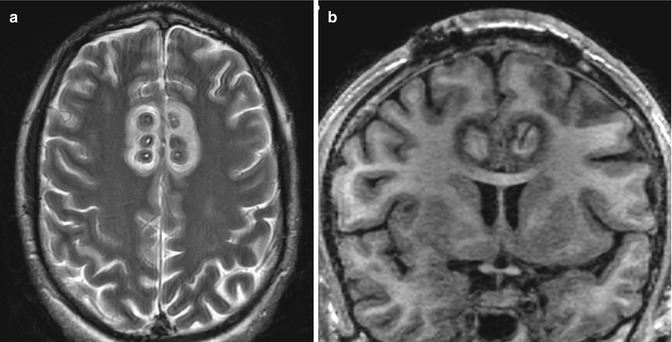
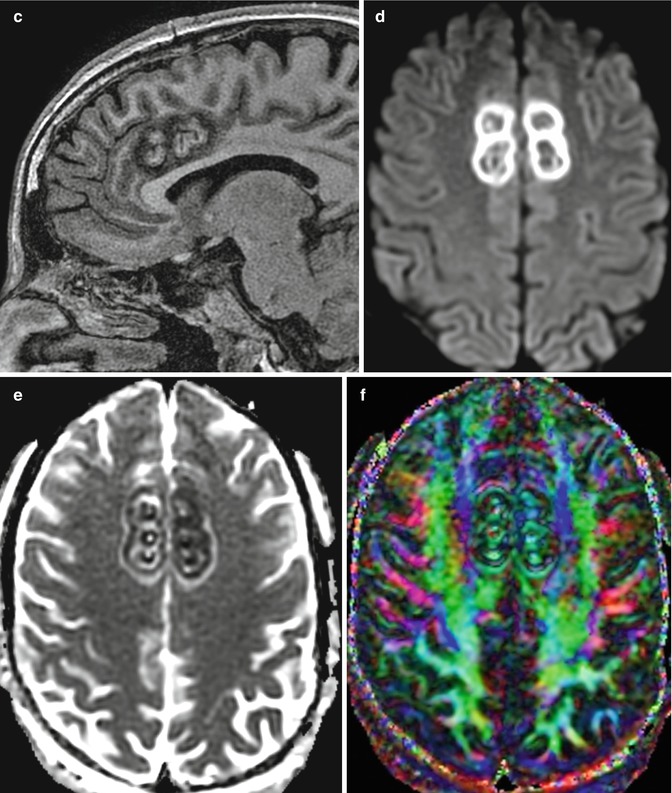
Fig. 5.33
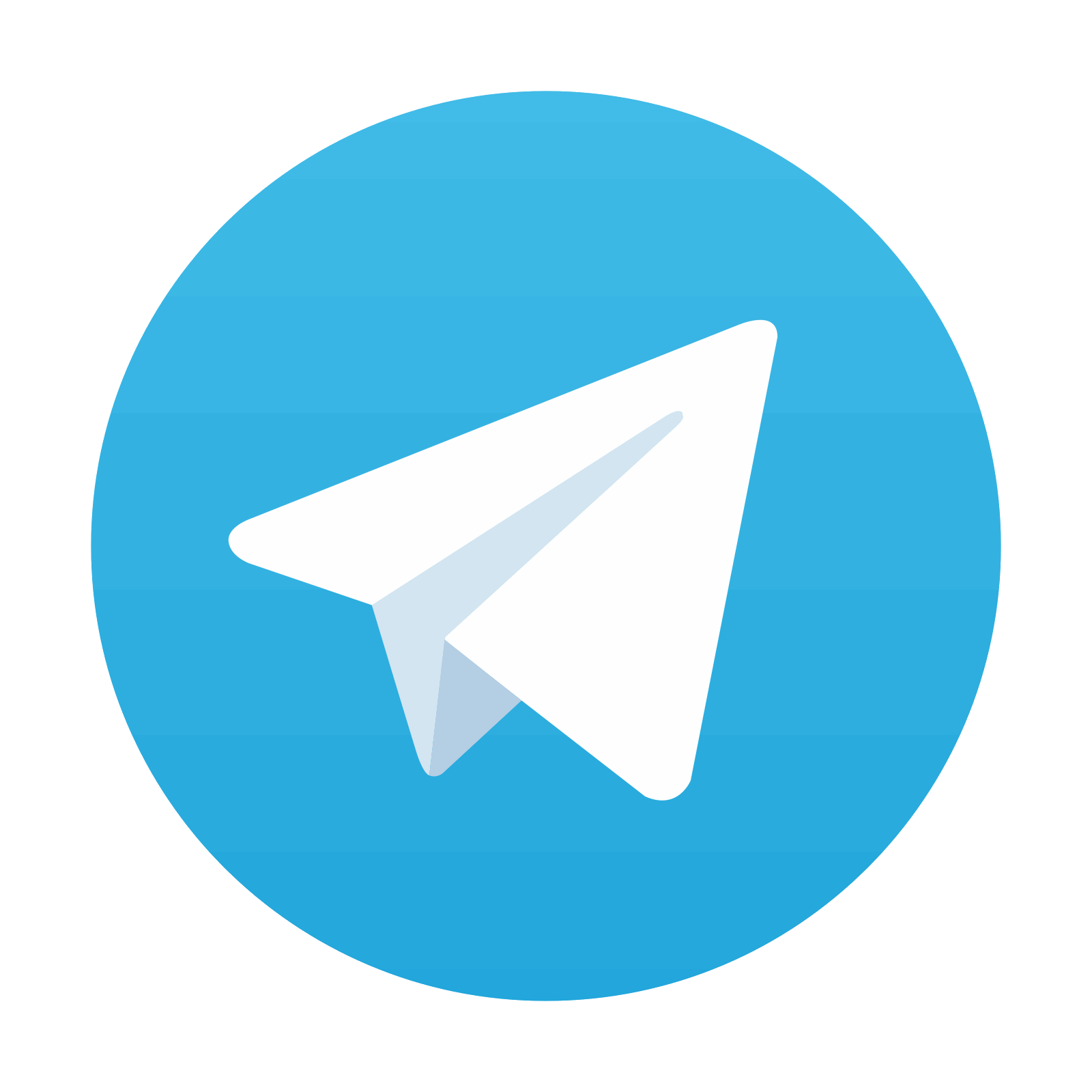
Bilateral anterior cingulotomy. The patient has a history of medically intractable obsessive-compulsive disorder treated with bilateral stereotactic microelectrode-guided anterior dorsal cingulotomy. Axial T2-weighted (a) and coronal (b) and sagittal (c) T1-weighted MR images show concentric rings of signal changes at each microelectrode insertion site in the bilateral anterior cingulate gyri. The diffusion-weighted image (d) and ADC map (e) show circular zones of restricted diffusion consistent with ischemia. The color fractional anisotropy map (f) shows interruption of the bilateral anterior cingulate fiber tracts
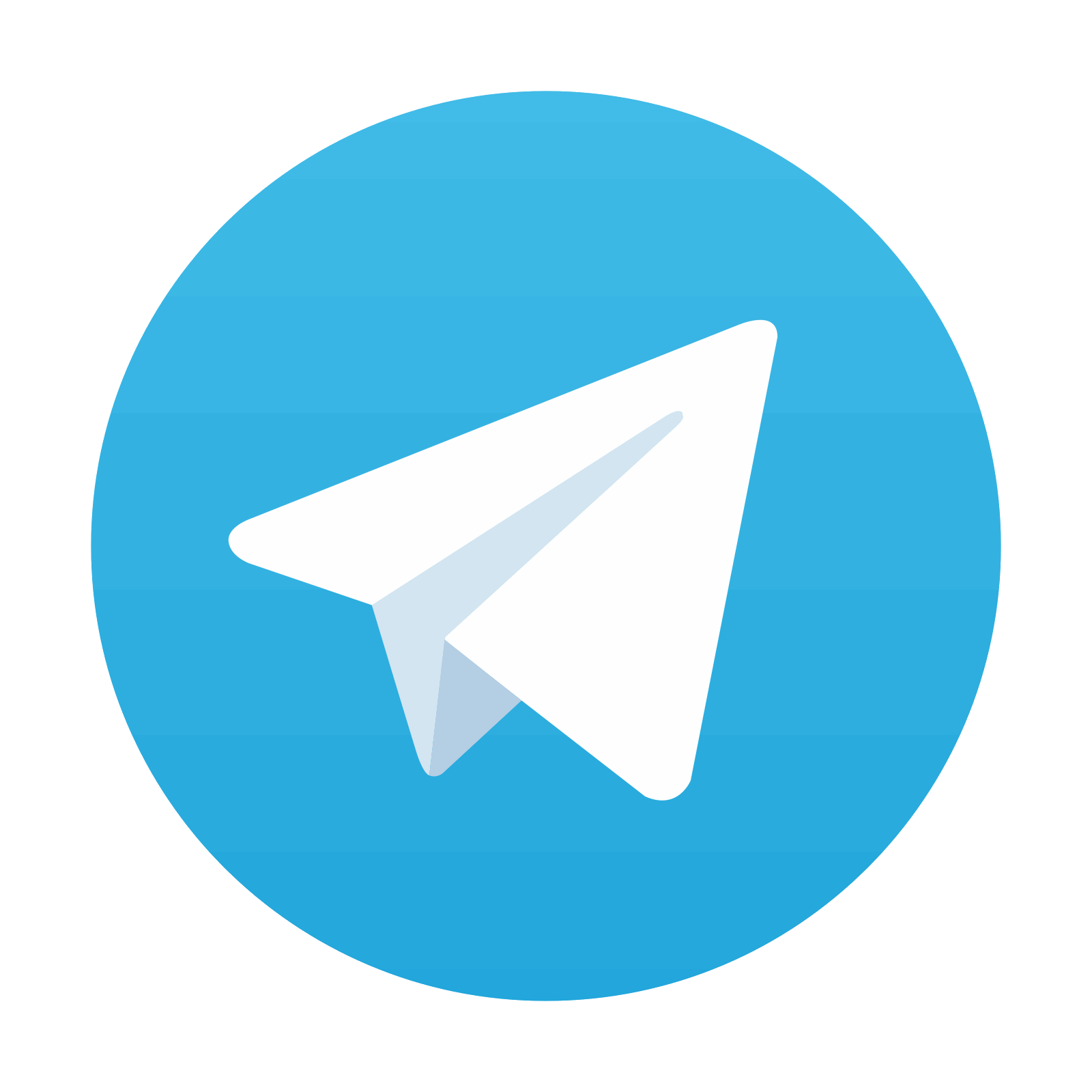
Stay updated, free articles. Join our Telegram channel

Full access? Get Clinical Tree
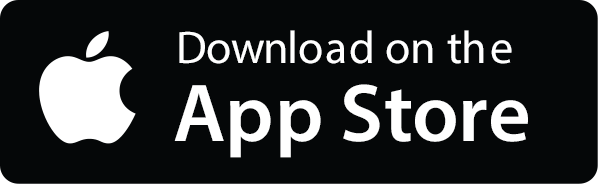
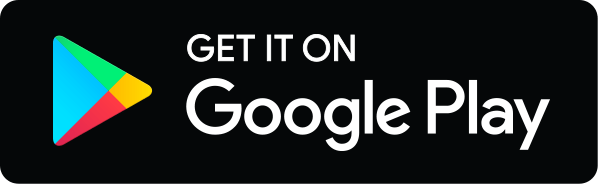
