Key points
- •
The incorporation of a simple, short, and easy-to-acquire sequence to visualize the vessel wall and detect intraplaque hemorrhage (IPH) is achievable now.
- •
Assessment of carotid disease should include baseline imaging to show stenosis, such as time of flight or contrast-enhanced magnetic resonance angiography, and commonly includes simple brain imaging to assess prior neuroischemic disease.
- •
Total imaging time is therefore likely to be in the order of 15 to 20 minutes.
- •
The addition of an IPH-detecting vessel wall sequence only adds a further 5 to 6 minutes to a baseline examination, which overall translates into 30 to 40 minutes of scanner table time.
Introduction
Over the last 20 years magnetic resonance(MR) imaging has become established as one of the mainstays of noninvasive imaging of carotid artery disease. Almost all investigations of the carotid artery are for the detection of the effects of atherosclerotic plaque at or around the carotid artery bifurcation. Investigation of the carotid artery may be triggered in the search for potentially symptomatic lesions as part of primary prevention, or in response to a neuroischemic event to secondarily prevent further brain ischemia.
Routine clinical carotid artery imaging comprises techniques that attempt to quantify vessel stenosis, which can be used to decide whether the patient is eligible for a nonmedical intervention. Interventions to reduce future cerebroischemic events have been shown to be warranted when the carotid vessel reaches a certain significant stenosis. However, although patients with significant stenosis are at higher risk of events, patients without such degrees of vessel narrowing may also be prone to transient ischemic attack or stroke, which suggests that the culprit vascular lesion is defined not only by stenosis but by other, currently hidden, features that make the vessel wall disease more prone to cause thromboembolic events.
In the same time period in which MR angiography (MRA) of the carotid artery has gained clinical acceptance as an accurate and clinically useful technique for detecting and measuring carotid artery stenosis, there has also been an active research agenda to use MR imaging to provide more information regarding vessel wall disease in an attempt to add to the MRA data already available. This work has added greatly to the understanding of the natural history of vessel wall disease in vivo. By identifying vessel wall components of atherosclerotic disease, an understanding of what is now known to be the complex pathobiology of atherosclerosis has informed the development of imaging biomarkers. One characteristic of high-risk plaque that has such an imaging surrogate is intraplaque hemorrhage (IPH). This article discusses the opportunity for introducing vessel wall imaging into clinical practice using a single sequence to detect IPH. Reluctance to do so earlier, despite the development of vessel wall MR imaging techniques, has likely stemmed from the perceived notion that implementation is a complex process requiring specialized equipment and sequences, and needing the addition of long imaging protocols with off-line postprocessing for interpretation. In addition, evidence that the information provided by such imaging is useful clinically has previously been sparse. This article therefore addresses some of these issues and positions the current and future role of clinical vessel wall MR imaging to detect IPH, and discusses the use of time-efficient imaging protocols to provide additional, clinically relevant information on current MR imaging platforms.
Introduction
Over the last 20 years magnetic resonance(MR) imaging has become established as one of the mainstays of noninvasive imaging of carotid artery disease. Almost all investigations of the carotid artery are for the detection of the effects of atherosclerotic plaque at or around the carotid artery bifurcation. Investigation of the carotid artery may be triggered in the search for potentially symptomatic lesions as part of primary prevention, or in response to a neuroischemic event to secondarily prevent further brain ischemia.
Routine clinical carotid artery imaging comprises techniques that attempt to quantify vessel stenosis, which can be used to decide whether the patient is eligible for a nonmedical intervention. Interventions to reduce future cerebroischemic events have been shown to be warranted when the carotid vessel reaches a certain significant stenosis. However, although patients with significant stenosis are at higher risk of events, patients without such degrees of vessel narrowing may also be prone to transient ischemic attack or stroke, which suggests that the culprit vascular lesion is defined not only by stenosis but by other, currently hidden, features that make the vessel wall disease more prone to cause thromboembolic events.
In the same time period in which MR angiography (MRA) of the carotid artery has gained clinical acceptance as an accurate and clinically useful technique for detecting and measuring carotid artery stenosis, there has also been an active research agenda to use MR imaging to provide more information regarding vessel wall disease in an attempt to add to the MRA data already available. This work has added greatly to the understanding of the natural history of vessel wall disease in vivo. By identifying vessel wall components of atherosclerotic disease, an understanding of what is now known to be the complex pathobiology of atherosclerosis has informed the development of imaging biomarkers. One characteristic of high-risk plaque that has such an imaging surrogate is intraplaque hemorrhage (IPH). This article discusses the opportunity for introducing vessel wall imaging into clinical practice using a single sequence to detect IPH. Reluctance to do so earlier, despite the development of vessel wall MR imaging techniques, has likely stemmed from the perceived notion that implementation is a complex process requiring specialized equipment and sequences, and needing the addition of long imaging protocols with off-line postprocessing for interpretation. In addition, evidence that the information provided by such imaging is useful clinically has previously been sparse. This article therefore addresses some of these issues and positions the current and future role of clinical vessel wall MR imaging to detect IPH, and discusses the use of time-efficient imaging protocols to provide additional, clinically relevant information on current MR imaging platforms.
Pathology
Atherosclerosis is initiated by several factors that disturb endothelial function, including aging, hyperglycemia, hypercholesterolemia, hypertension, and smoking. Endothelial dysfunction manifests as loss of normal antiinflammatory, antithrombotic, and vasoreactive functions. Normal cholesterol handling at the interface between the vessel wall and lumen is disturbed such that there is a net ingress of cholesterol into the intima and increased cholesterol residency. Over time, cholesterol coalesces into lipid pools. The intraplaque environment results in lipid oxidation with the production of oxygen free radicals.
In response to these changes, circulating white cells are attracted within the plaque through endothelial activation and expression of surface receptors. Circulating monocytes enter the plaque and transform into tissue macrophages. Phagocytosis of plaque lipids by macrophages results in foam cell formation commonly leading to macrophage apoptosis rather than removal of lipid from the vessel wall. A reparative response to disease within the plaque results in stimulation of smooth muscle cell proliferation and the development of intima-medial thickening in an attempt to stabilize the inflammatory process.
An indirect effect of plaque surface thickening is loss of the usual oxygen diffusion from the lumen into the vessel wall once the thickening increases to more than 200 μm. In response to this, secondary to release of factors such as HIF-1 (hypoxic inducible factor), and the presence of intraplaque macrophages, there is a release of VEGF (vascular endothelial growth factor), which stimulates neovessel growth within the plaque predominantly from the normal vasa vasorum within the adventitia. However, the new vessel growth results in leaky, friable vessels, vulnerable to red cell deposition and frank hemorrhage within the vessel wall. The trigger for plaque hemorrhage is not clearly understood but plaque morphology, hemodynamics, or changes in systemic blood pressure, particularly the pulse pressure, may result in repeated microhemorrhages within the plaque ( Fig. 1 ).
The delivery of red blood cells within the plaque has several deleterious effects. First, if the volume of blood deposited in the atherosclerotic plaque is large it can result in a significant change in volume of vessel wall disease and potential encroachment on the lumen. This process has been seen as one of the causes of rapid changes in stenosis; rapid reduction can also occur with blood resorption. Likely, and more common, are repeated small hemorrhages, not causing a significant change in plaque volume. These hemorrhages result in repeated delivery of red cells deep within the vessel wall lesion, which has 2 significant and related effects. Red blood cell membranes have one of the highest cholesterol contents of any cell in the human body, and this is further increased in states of hypercholesterolemia. Each red cell delivered into the plaque therefore adds to the lipid core. Following red cell lysis, the other major component directly introduced within the plaque is hemoglobin. As this undergoes degradation, free heme is released. Heme is highly inflammatory and through the Fenton reaction drives the repetitive production of oxygen free radicals, which continue to feed the hostile plaque environment.
The combination of cholesterol and heme delivered with every red cell therefore provides an inflammatory combination driving the atherosclerotic process. In response there is further monocyte/macrophage migration into the plaque, which results in further inflammatory cytokines, angiogenesis, lipid oxidation, and cell death causing enlargement of the plaque’s necrotic core, neovascularization, and subsequent hemorrhage. This vicious cycle likely accounts for the acceleration in atherosclerotic disease from a slow, predictable progression to more rapid, unpredictable plaque progression and disruption. The presence of plaque hemorrhage therefore denotes a more advanced atherosclerotic state (American Heart Association [AHA] type VI) in keeping with increased plaque vulnerability and plaque progression .
Targeting of many of these plaque characteristics with imaging can provide significant information about the state of vessel wall disease, but, at the present time, the clinical need is evidence that, in addition to their theoretic credentials, one or more of these imaging markers can provide useful information to further guide patient management. One of these markers, IPH, has come under particular scrutiny because of its many pathobiological and imaging features that make it attractive as a biomarker of plaque vulnerability. The World Health Organization has broadly defined a biomarker as “any substance, structure, or process that can be measured in the body, or its products, that influence or predict the incidence of outcome or disease.” IPH could represent such a substance/process provided that it predicts outcomes related to carotid disease. Accepting this, there are still several barriers to adoption that must be overcome in order for this to be introduced into routine clinical imaging of carotid disease.
Introduction to clinical practice
Introduction of IPH MR imaging to clinical carotid artery imaging has few real barriers to adoption. The sequences required to apply this technique are readily available on all scanner platforms; they are rapidly acquired; IPH detection is accurate and interpretation is simple, presently comprising dichotomization into presence or absence of IPH. Furthermore, MR imaging at present is the only readily available imaging technique for the detection of plaque hemorrhage, and MR imaging is already a technique used for investigating carotid artery disease, thus making the addition of this 1 sequence to the standard carotid artery imaging protocol simple, with little time-cost impact.
Clinical applications
Addition of IPH imaging to routine carotid artery MR imaging assessment has the potential to affect patient management in several clinical settings: whether the patient is asymptomatic but at high risk of vascular disease; symptomatic and being assessed for risk of future event; or in the work-up before carotid artery intervention. Newer applications may include identifying high-risk disease requiring modified, intensive, or costly therapy; identification of patients requiring increased monitoring; entry criteria for therapeutic trials; and phenotyping of high-risk patients who may require more comprehensive vascular investigation and potentially therapy modification.
Primary prevention
In the absence of organized vascular screening programs, asymptomatic carotid arteries are most commonly imaged because of high-risk atherosclerotic vascular disease in another vascular bed, such as the coronary or peripheral vascular circulation. Alternatively, asymptomatic vessels are commonly imaged in conjunction with a symptomatic contralateral carotid artery. Despite the silent nature of these carotid vessels, study of asymptomatic cohorts has repeatedly shown an increased risk of future clinical events, irrespective of the degree of stenosis, in those patients with MR-demonstrated IPH rather than in those without ( Fig. 2 ). Importantly, the very low rate of events in the IPH-negative groups is probably an even more compelling reason to investigate patients using IPH MR imaging. The absence of IPH seems to confer a level of low risk that might be useful in managing patients, even those with significant carotid artery stenosis, potentially allowing the withholding of invasive interventions Carotid vessels with no stenosis are also found to contain IPH, suggesting that, despite representing advanced vessel wall disease, IPH can occur even when vessel wall thickening is minimal ( Fig. 3 A). Large cohort trials currently underway may provide information on the relevance of finding this high-risk biomarker in otherwise normal people, potentially confirming whether this can act as an early marker of future events even in the absence of other more established risk markers.
Asymptomatic patients found to have IPH may be candidates for more intensive management despite the lack of recognized risk factors such as luminal stenosis. Patients screened for carotid disease because of vascular disease elsewhere are known to have asymptomatic normal or nonstenotic carotid disease identified with IPH MR imaging. Knowing that IPH is a stimulus for plaque progression and potentially carotid stenosis progression these patients could be identified for more follow-up in order to better detect the occurrence of significant stenosis (which may then be eligible for surgical intervention). Before the need for surgical intervention, the risk/benefit ratio of more intensive medical therapy can be weighed, in the knowledge that these patients are at higher risk of disease progression ( Fig. 3 B).
The concept of identifying high-risk patients by identifying high-risk lesions could be further expanded. From the prospective study of clinical outcomes of patients with histologically proven IPH in their carotid endarterectomy specimens and the retrospective review of comorbid cardiovascular conditions in patients with and without carotid IPH, it seems that the status of the carotid artery, and in particular the presence or absence of IPH, provides a useful biomarker of the patients’ vascular phenotypes. IPH in the carotid bed therefore seems to associate with symptomatic vascular disease peripherally and in the coronary circulation, and is also able to predict risk of future cardiovascular events. It could be argued that medical therapy for 1 vascular bed (ie, carotid) has an equally beneficial effect in all vascular beds and therefore no further investigation is required elsewhere. However, a case could be made for the investigation and exclusion of other high risk, silent lesions (eg, in the proximal coronary arteries) once carotid IPH has been detected.
Knowing that IPH represents a marker of increased risk of atherosclerotic progression and clinical outcomes, the technique could be used to improve the selection of patients for proof-of-principle trials of new interventions. Using MR imaging–detected IPH as a predictive classifier to select those patients at greater risk of events, smaller recruitment numbers will be required, allowing the demonstration of effect more efficiently.
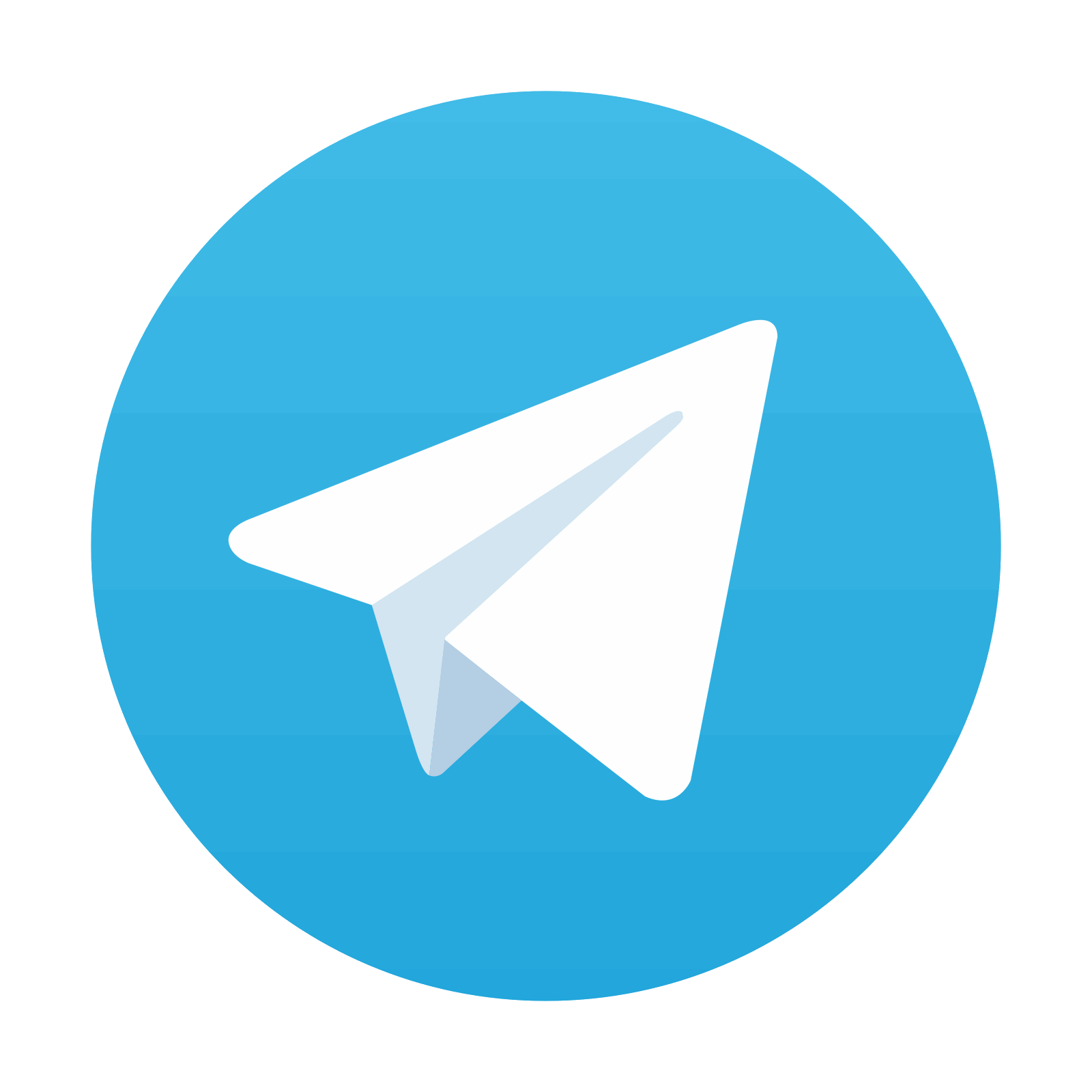
Stay updated, free articles. Join our Telegram channel

Full access? Get Clinical Tree
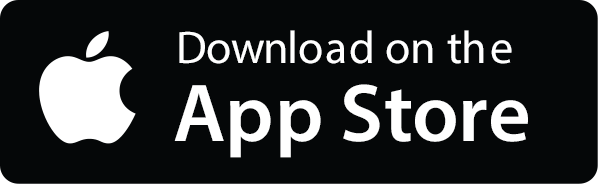
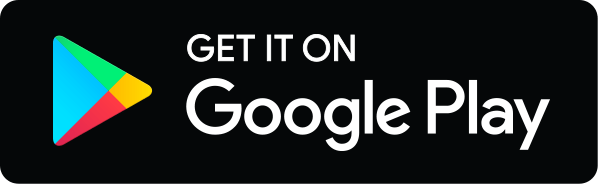