Fig. 7.1
Lateral radiograph demonstrating infective spondylitis at the T11–12 level. Note destruction of the two opposite end plates and subchondral bone with bone fragmentation (arrow)
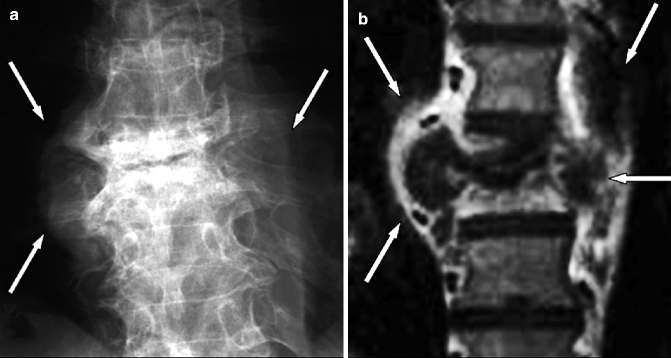
Fig. 7.2
Tuberculous spondylitis at T7–8 level in a 69-year-old man. (a) Anteroposterior radiograph showing a bilateral area of fusiform soft tissue swelling (arrows). (b) Post-contrast T1-weighted fat-suppressed MR image clearly demonstrates the corresponding paraspinal abscesses (arrows)
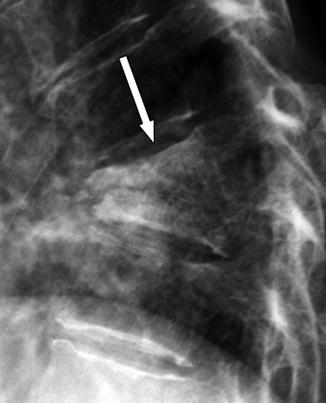
Fig. 7.3
Tuberculous spondylitis in the same patient as in Fig. 7.2. Lateral radiograph of the thoracolumbar spine shows ankylosis of the residual vertebral bodies and formation of a single bony block (arrow)
7.2.4.2 Computed Tomography
Rapid advances in CT technology have led to the current generation of multidetector CT scanners which have faster acquisition, increased anatomical coverage, higher spatial resolution, and isotropic data acquisition. The improvement in diagnostic accuracy and the gain is perhaps best exemplified by the rise in the use of detailed multiplanar reformations in spinal imaging. Spiral CT imaging with high-quality 2D- and 3D-reformatted images allows a clear assessment of very small vertebral foci of infection, minimal erosions of the end plates, bone destruction, and soft tissue involvement in the paravertebral and epidural spaces (Fig. 7.4). Although not overall as sensitive as MR imaging, CT remains the preferred imaging modality for the assessment of bone sequestra (Fig. 7.5) and pathological calcifications (Fig. 7.6). Furthermore, it is particularly helpful in identifying atypical foci of TB, especially in the posterior neural arch (Fig. 7.7) allowing differentiation from other destructive processes such as metastases and other infectious etiologies. Additionally, percutaneous diagnostic needle biopsy and drainage of abscesses with identification of the causative agent can be performed with the guidance of CT images. Notably, small to large paraspinal abscesses may be better demonstrated on CT and MR imaging in the pre- and paravertebral regions. The thick nodular rim of an abscess on a pre-contrast scan represents the hypervascular, hypercellular, fibrotic wall of the inflammatory cavity; after contrast administration, there usually is strong rim enhancement around low-attenuation multiloculated fluid collection (Brandt-Zawadzki et al. 1983). The identification of a multilocular and partially calcified paraspinal abscess with a contrast-enhancing rim associated to a destructive vertebral body lesion is highly suggestive of a tuberculous rather than pyogenic infection.
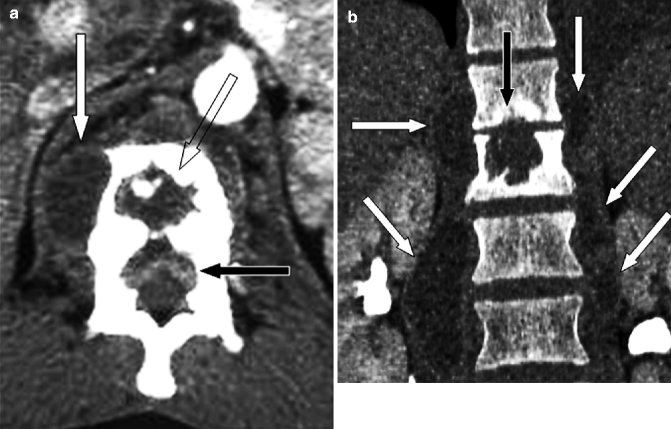
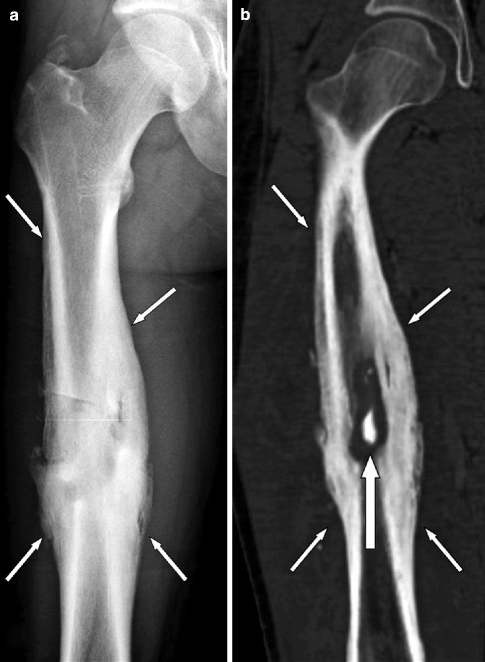
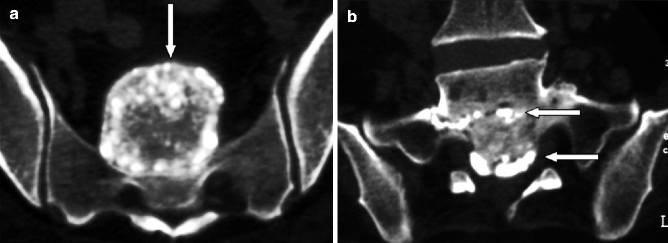
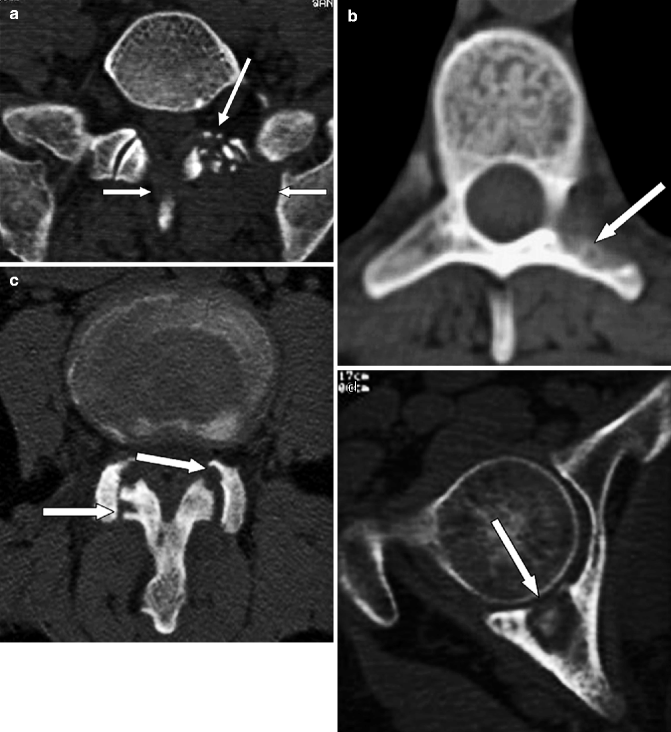
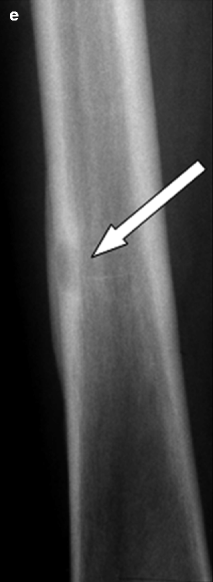
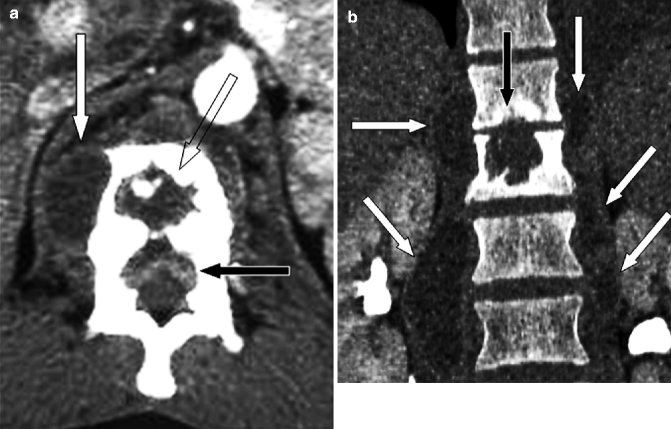
Fig. 7.4
Tuberculous spondylitis at T12–L1 level in a 63-year-old Chinese man. (a) Contrast-enhanced axial CT image at L1 level shows a paravertebral abscess (white arrow), lytic destruction of the vertebral body (empty arrow), and an epidural abscess (black arrow) compressing the thecal sac. (b) Coronal reconstruction CT image better defines the lytic destruction of L1 vertebral body (black arrow) and shows large abscesses in the psoas muscles (white arrows)
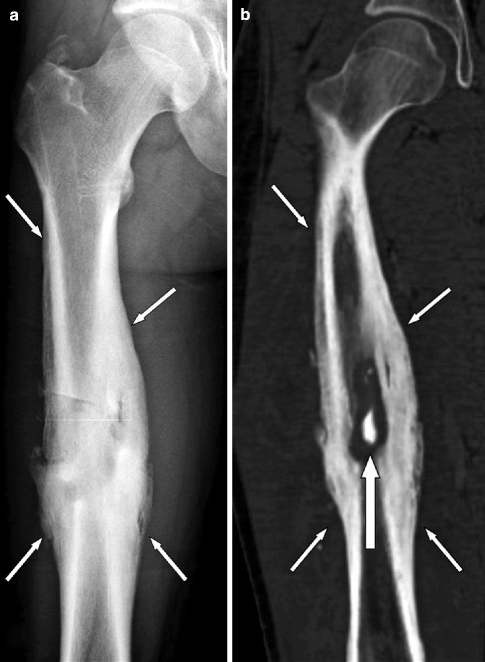
Fig. 7.5
Chronic osteomyelitis of the right femur in a 61-year-old man. (a) Anteroposterior radiograph demonstrating a medullary osteolysis associated with surrounding sclerosis and endosteal and periosteal new bone formation (arrows). (b) Coronal CT-reformatted image demonstrating a bone sequestrum (arrow) walled off by involucrum (small arrows)
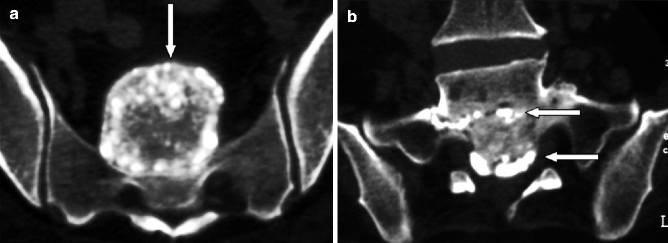
Fig. 7.6
Chronic TB at L5–S1 level in a 65-year-old man. (a, b) Coronal CT scans showing multiple calcified caseous deposits in a well-marginated sacral and presacral mass (arrow in a) as well as in the L5–S1 and S1–2 disk spaces (arrows in b)
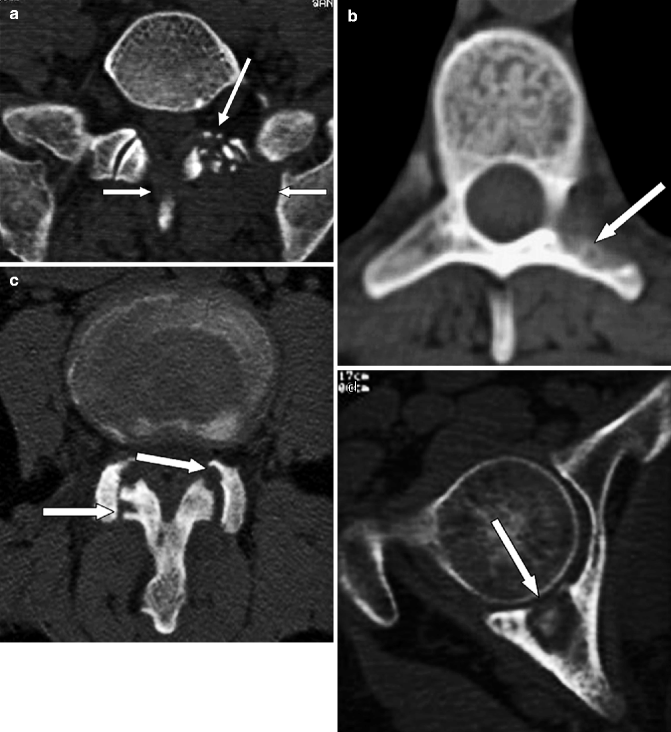
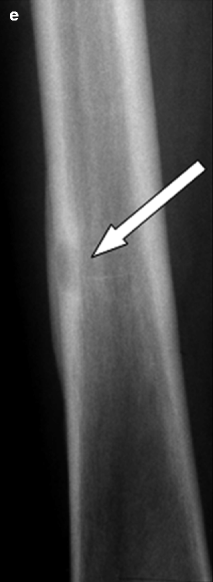
Fig. 7.7
Skip tuberculous lesions in a 69-year-old man with concurrent active pulmonary disease. (a) Axial CT image of the lumbosacral junction (bone window) shows a large paraspinal mass (arrows) with bony fragments within (long arrow) on the left. (b) Axial CT image (bone window) in the lower thoracic spine demonstrates bony destruction of the left pedicle and transverse process mimicking metastases (arrow). (c) Axial CT image (bone window) at L2–3 level shows facet joint arthritis with erosions and bony destruction of the articular processes (arrows). (d) Axial CT image (bone window) passing through the right acetabulum shows an osteolytic lesion of the posterior wall with a bony fragment within and erosion of the articular surface (arrow). (e) AP radiograph of the left femur shows sharply marginated lytic focus (arrow) in the diaphysis with surrounding sclerosis and laminated periosteal reaction rather difficult to differentiate from other infections
7.2.4.3 Magnetic Resonance Imaging
High contrast resolution, high sensitivity for soft tissue and bone marrow lesions, direct multiplanar imaging capability, and its lack of ionizing radiation make MR imaging the modality of choice for the diagnosis of inflammatory disorders and their sequelae (Peterfy et al. 1994). MR imaging should be employed as soon as infection is suspected because of its high sensitivity and specificity to detect early infection, evaluate the full extent of disease, and monitor therapeutic response (Sharif et al. 1990; Tyrrell et al. 1999; Leone et al. 2000; Ledermann et al. 2003; Jevtic 2004; Tins and Cassar-Pullicino 2004; Tali and Gültekin 2005). A disadvantage of MR imaging is that cortical bone involvement is not as well visualized as by CT. The standard MR imaging protocol should routinely include fluid-sensitive images (i.e., short-tau inversion recovery (STIR) or fat-saturated T2-weighted images), due to their high sensitivity to early inflammatory edema and initial infectious foci. On T2-weighted spin-echo (SE) images, the signal changes may not be readily apparent due to the bright signal intensity of marrow fat (Fig. 7.8). The addition of T1-weighted SE pre- and post-contrast T1-weighted fat-suppressed sequences clearly demonstrates all anatomical details and differentiates between vascularized and nonvascularized tissue, including necrotic inflammatory components such as soft tissue involvement, abscesses, dural involvement, and bone sequestra (Figs. 7.9, 7.10, and 7.11). Unenhanced T1-weighted images should be nonfat-suppressed, since intrinsic contrast resolution of bone marrow and paraspinal fat is the guide for image interpretation. The suppression of the signal from fat tissue is necessary to increase sensitivity on T2-weighted and post-contrast T1-weighted images. This is clearly understood when dealing with gadolinium-enhanced T1-weighted images, due to the difficulty of differentiating gadolinium enhancement from fat tissue signal. Diffusion-weighted (DW) imaging with measurement of the apparent diffusion coefficient (ADC) has been widely utilized in musculoskeletal radiology (Harish et al. 2011); it can assess the response of tumors to therapy, differentiate residual tumor from necrosis, distinguish pathologic from benign vertebral body compression fractures, diagnose vertebral osteomyelitis and epidural abscess (Fig. 7.10), identify active changes in inflammatory arthritis, and evaluate muscle involvement in inflammatory myositis, including assessing disease progression and monitoring treatment response. The restricted diffusion in inflammatory myositis is mild and better assessed quantitatively. In contrast, there is usually more avid restriction of diffusion within pockets of pus in skeletal abscesses obviating the need for quantifying ADC values. Diffusion-weighted imaging has technical challenges in the musculoskeletal system: imaging parameters should be adjusted to account for decreased signal-noise ratio, susceptibility artifacts, and motion artifacts from patient movement and arterial pulsation. In the musculoskeletal system, diffusion-weighted imaging can be improved by shortening the acquisition time by using a single reference slice and decreasing the slice matrix, decreasing the strength of the diffusion gradient from 500 to 1,000 s/mm2, and using proper restraint and avoiding large vessels when possible. Quantitative MR imaging (Stabler et al. 2000) clearly shows reactive bone marrow changes in adjacent unaffected vertebrae in about 25 % of patients with infectious spondylitis. The signal intensity changes and increased percentage enhancement associated with this disease are similar to those of myeloproliferative diseases, hematologic neoplasia, and stimulation of cellular marrow in hemolytic anemias. In clinical practice, one should be aware of this phenomenon so as not to confuse diffuse bone marrow changes in infectious spondylitis with bone marrow diseases. MR imaging signs of an infective process include low-intensity signal on T1-weighted images, high-intensity signal on fluid-sensitive images, contrast enhancement of the vertebral bodies and intervening disk, evidence of either paraspinal or epidural inflammatory tissue, and erosion or destruction of the vertebral end plates (Fig. 7.10) (Leone et al. 2000; Ledermann et al. 2003; Hong et al. 2009). Pathophysiologically, the earliest response to infection in a vertebral body is accumulation of extracellular fluid within the bone marrow. MR imaging demonstrates this bone marrow edema before the destructive bone changes take place (Fig. 7.8). The infective process usually is adjacent to vertebral end plates and on T1-weighted images the end plates are ill defined: the intervertebral disk and adjacent vertebral bodies show low-intensity signal, while fluid-sensitive images show high signal intensity from the bone marrow and affected intervertebral disk (Fig. 7.10). The lack of visualization of the nuclear cleft in the infected disk on T2-weighted images has been reported to be indicative of spinal infection (Modic et al. 1985). However, this sign is only rarely applicable since the nuclear cleft is rarely visible in the cervical and thoracic spine on T2-weighted images, and its clinical use is therefore limited (Ruiz et al. 2000; Ledermann et al. 2003). Following intravenous gadolinium administration, the pattern of disk enhancement is variable including homogeneous enhancement of most of the disk, patchy nonconfluent areas of enhancement, and thin-to-thick peripheral enhancement. Generally, infected bone marrow enhances diffusely (Sharif 1992). In untreated infections, signal changes progress across the disk to involve the adjacent vertebral body giving the classical appearance of two vertebrae on either side of an involved disk, with destruction of the vertebral end plates (Fig. 7.9). The extension of inflammatory edema beyond the limits of the vertebral bodies and the annulus fibrosus into the pre- and paravertebral region will cause paraspinal phlegmon (i.e., a mass of granulation tissue) or abscesses (Figs. 7.9 and 7.10). The posterior extension may intrude into the epidural space or posterolaterally into the intervertebral foramina; this is well demonstrated on parasagittal and axial images, with obliteration of the perineural fat. Abnormal soft tissue within the epidural space is usually located anteriorly, close to the primary infectious site (Fig. 7.10). More rarely, distant epidural involvement or isolated epidural abscess may occur. Differentiation between a phlegmon and an abscess is extremely important, since treatment is different. Surgery is often indicated for an abscess, whereas a more conservative approach with antibiotic therapy is preferred for phlegmon. The differential diagnosis is possible only by intravenous gadolinium administration (Numaguchi et al. 1993), since diffuse homogeneous contrast enhancement is consistent with phlegmon (Fig. 7.11) and a rim of contrast enhancement lining a nonenhancing liquefied center indicates an abscess (Figs. 7.1, 7.9, and 7.10). The postinflammatory phase is histologically characterized by the presence of vascularized fibrous tissue, fat bone marrow transformation, subchondral fibrosis, and osteosclerosis, all changes which are clearly demonstrated by MR imaging. Therefore, MR imaging allows assessment of the response to treatment. This is shown as the normalization of intervertebral disk and adjacent bone marrow signal intensity, reduction in paravertebral soft tissue, and intraspinal involvement (Fig. 7.11), including reduction of gadolinium enhancement. A progressive increase in signal intensity on T1-weighted images within previously affected vertebrae suggests fatty marrow replacement and indicates healing; this has been found to correlate well with resolving clinical signs and symptoms (Fig. 7.11) (Hong et al. 2009). The disk space is narrow, but the high-intensity signal on fluid-sensitive images regresses, demonstrating the resolution of disease process. However, high-intensity signal on fluid-sensitive images may persist for some time after the patient has improved clinically. Notably, imaging abnormalities often persist in patients with bacterial spondylodiskitis despite a favorable clinical and biological response to antibiotic treatment. This is not associated with relapses, neurological sequelae, or persistent pain. Close imaging controls are not necessary when bacterial spondylodiskitis responds favorably to treatment (Zarrouk et al. 2007). Continuing destructive bone changes suggest inappropriate diagnosis or treatment.
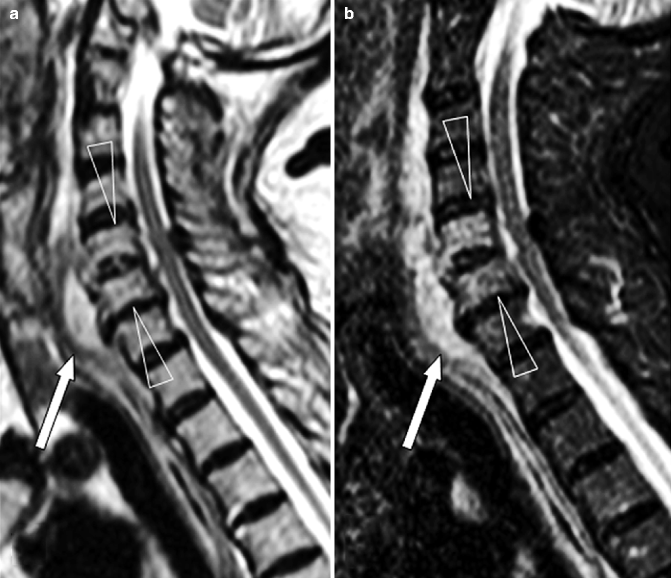
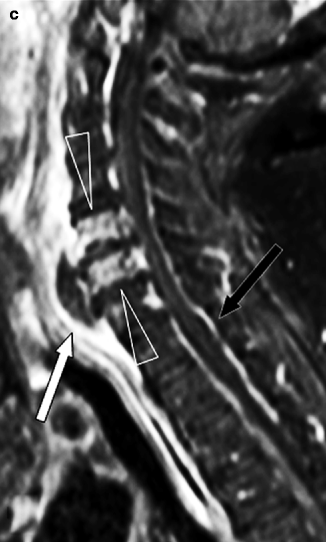
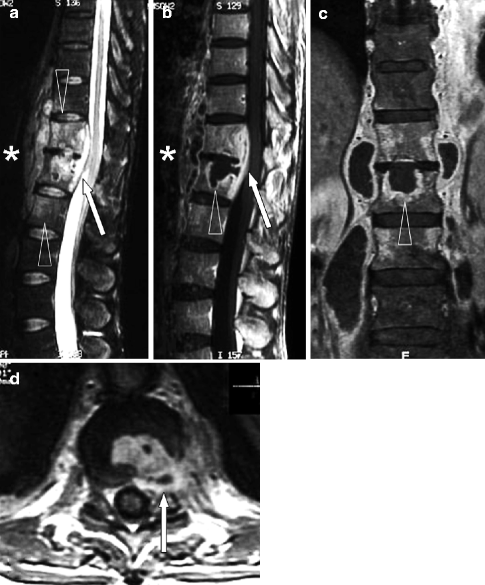
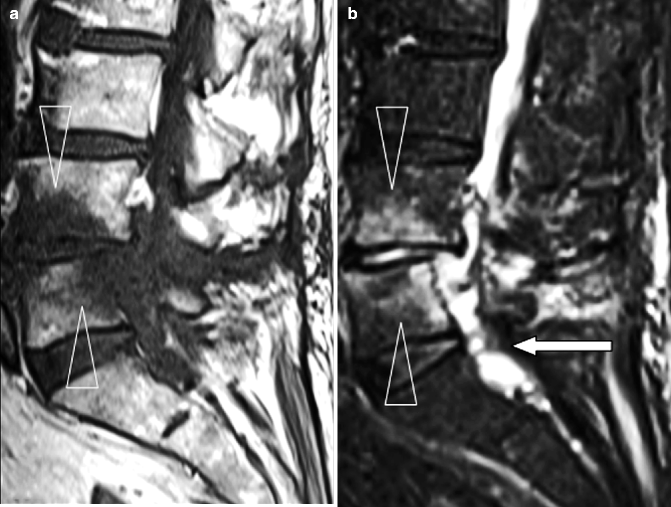
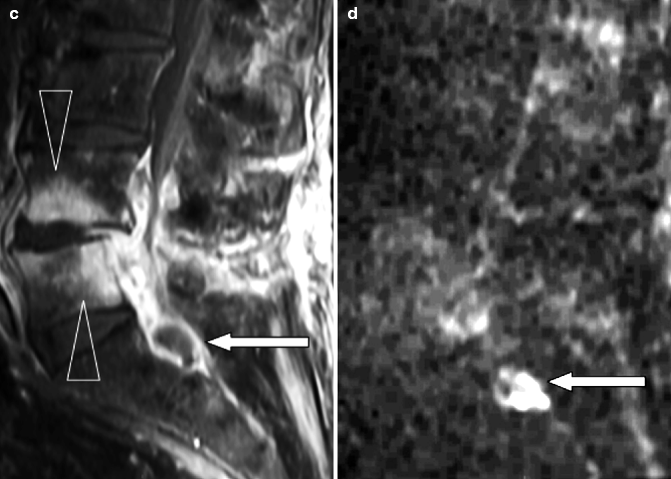
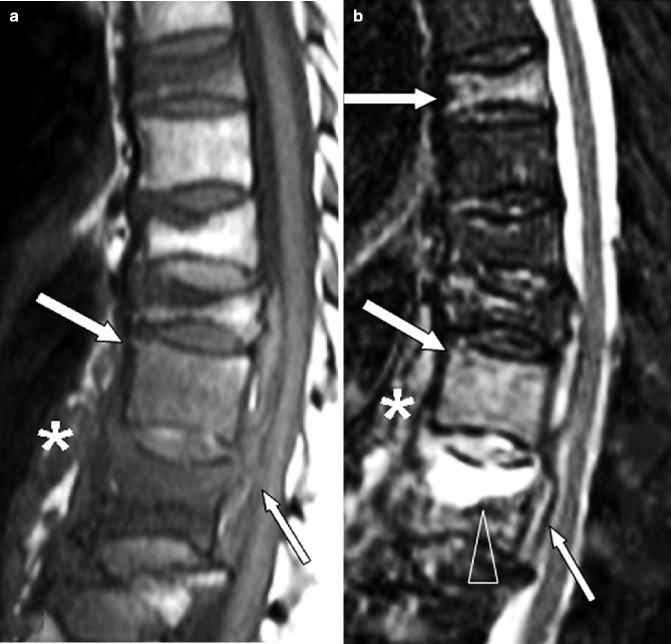
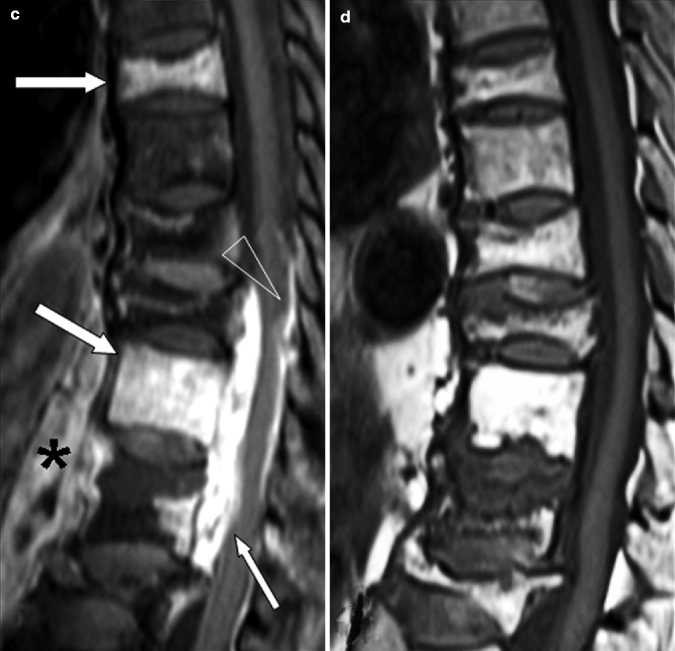
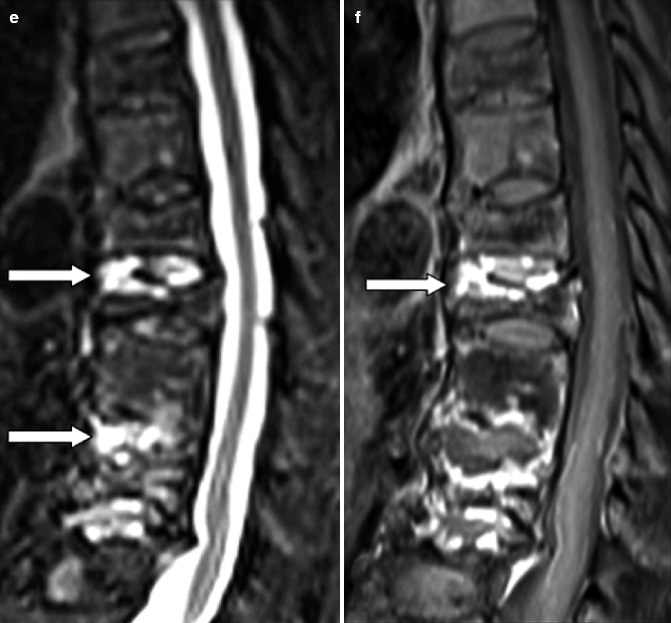
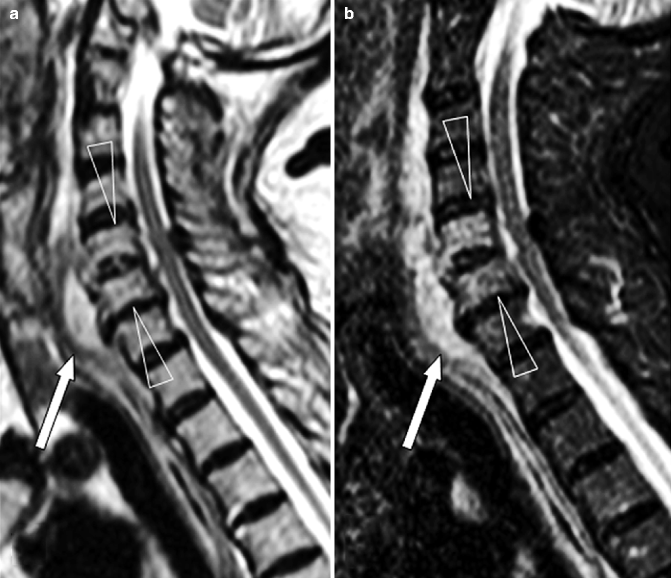
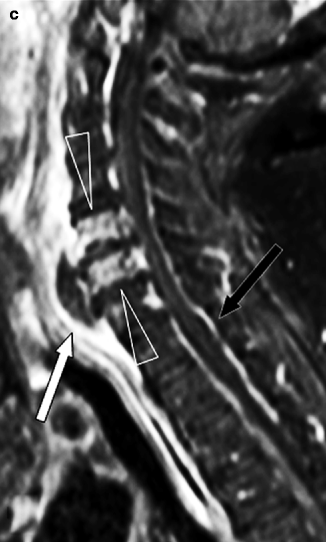
Fig. 7.8
Cervical spondylodiskitis and pachymeningitis in a 72-year-old man. (a) Sagittal T2-weighted, (b) STIR, and (c) post-contrast T1-weighted fat-suppressed images show a prevertebral peripherally enhancing soft tissue fluid collection consistent with abscess (arrows in a–c), bone marrow edema of the bodies of C5 and C6 (arrowheads in b), and pachymeningeal post-contrast enhancement (black arrow in c). Note that the STIR image is superior to T2-weighted image in demonstrating bone edema (arrowheads in a, b). Post-contrast T1-weighted fat-suppressed images are unique in their ability to demonstrate pachymeningeal involvement
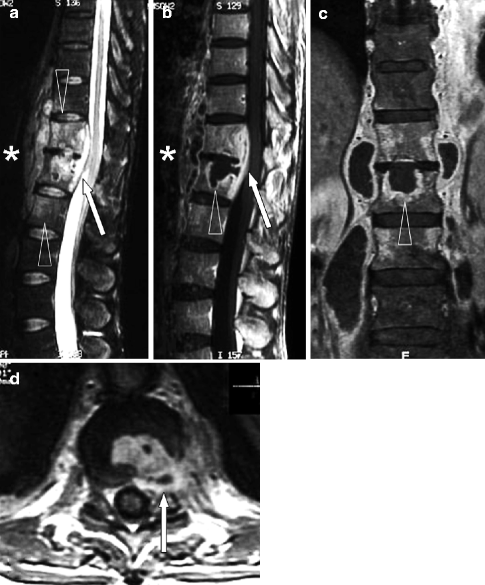
Fig. 7.9
Tuberculous spondylitis in the same patient as in Fig. 7.4. (a) Sagittal fat-suppressed T2-weighted image shows diffuse increased signal within the T12 and L1 bodies and regions of increased signal within the L2 body (arrowheads) and T12–L1 intervertebral disk. Note the large prevertebral soft tissue mass spreading upwards up to T10 and downwards as low as L2 (*) and the epidural soft tissue mass obliterating the anterior subarachnoid space (arrow). (b) Sagittal post-contrast T1-weighted fat-suppressed image shows thick rim of enhancement around the intraosseous fluid collection (arrowhead), the epidural mass (arrow), and within the prevertebral soft tissues (*). Rim enhancement denotes abscess formation. (c) Coronal post-contrast T1-weighted fat-suppressed image confirms the intraosseous fluid collection (arrowhead), and shows tuberculous involvement of T12, L1, and L2 vertebral bodies, as well as large peripherally enhancing abscesses in the psoas muscles. (d) Axial post-contrast T1-weighted fat-suppressed image better defines the epidural abscess (arrow)
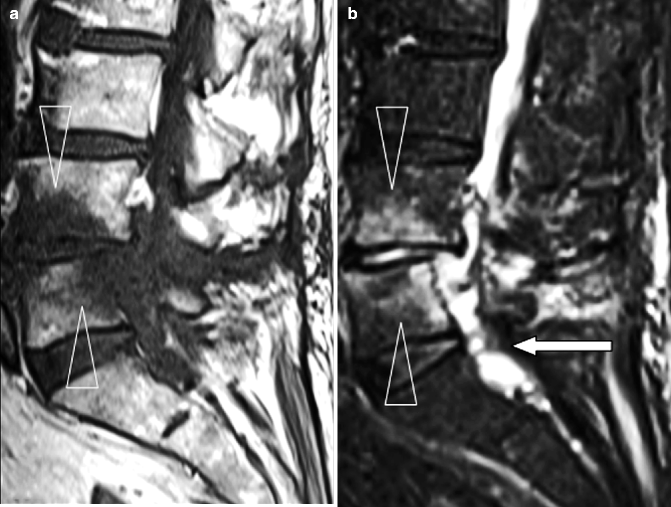
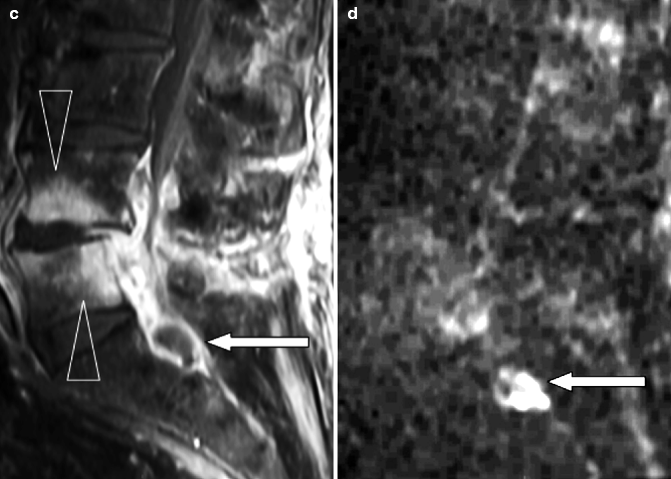
Fig. 7.10
Postoperative spondylodiskitis at L4–5 level. (a) Sagittal T1-weighted MR image demonstrating reduction of disk height at the L4–5 level with decreased signal within the adjacent vertebral bodies (arrowheads). (b) Sagittal fat-suppressed T2-weighted image showing diffuse increased signal of L4 and L5 bodies and intervening intervertebral disk (arrowheads). There is a soft tissue mass obliterating the spinal canal (arrow). (c) Sagittal post-contrast T1-weighted fat-suppressed image shows enhancement of the L4 and L5 bodies and posterior aspect of the intervening disk (arrowheads). Rim enhancement of the epidural mass denotes abscess formation. (d) Sagittal diffusion-weighted (b: 800 s/mm2) MR image demonstrating restricted diffusion of the epidural abscess
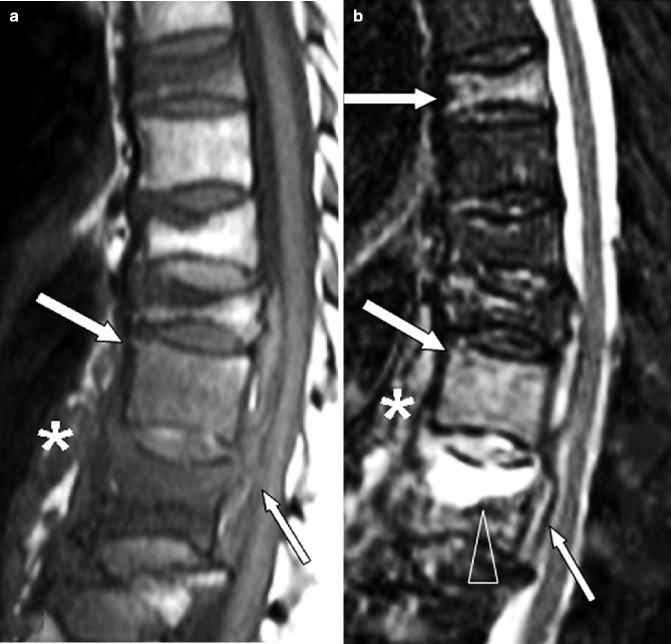
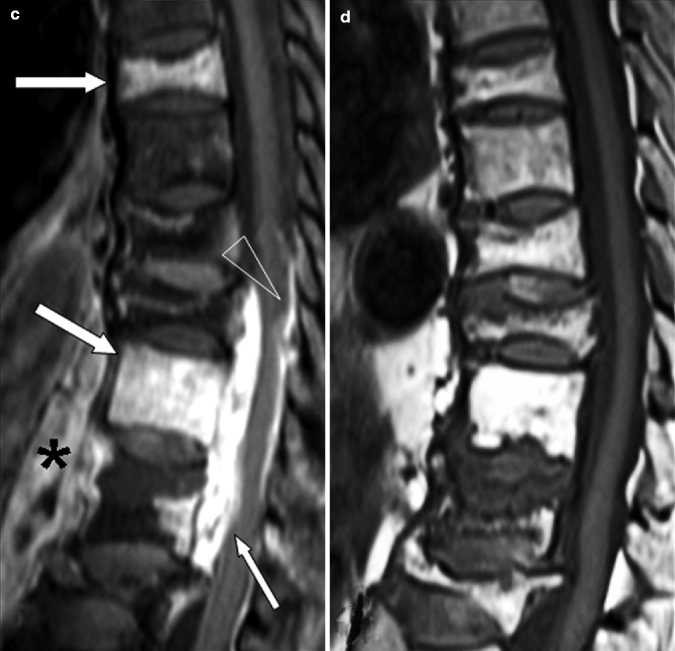
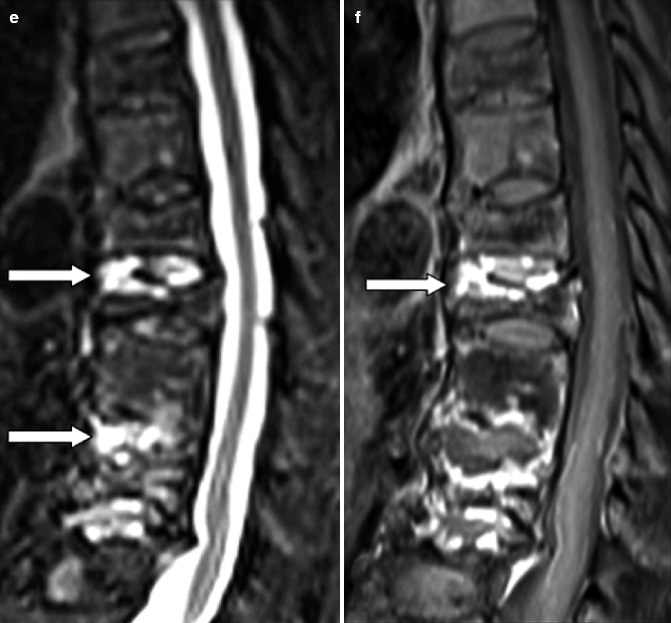
Fig. 7.11
Multilevel tuberculous spondylitis in a 72-year-old man. (a) Sagittal T1-weighted and (b) STIR images show typical signs of multilevel infection. There is marrow edema within the vertebral bodies of T7, T11, and T12 (white arrows in a, b); increased signal within the T11–12 disk; a soft tissue mass posterior (small arrow in a, b) and anterior (* in a, b) to the T10, T11, and T12 bodies, respectively; and a large intraosseous fluid collection (arrowhead in b). (c) Sagittal post-contrast T1-weighted fat-suppressed image demonstrates enhancement within T7, T11, and T12 vertebral bodies (white arrows), almost uniform enhancement of the epidural mass indicative of an epidural phlegmon (small arrow), and a pachymeningeal involvement (arrowhead). Rim enhancement within the prevertebral soft tissues denotes abscess formation (*). (d) Sagittal T1-weighted, (e) STIR, and (f) post-contrast T1-weighted fat-suppressed MR images 1 year after appropriate antibiotic therapy. There is increased signal within T9–10 and T11–12 intervertebral disks (arrows in e), a little enhancement within the T9–10 intervertebral disk (arrow in f), but there has been very marked improvement in the overall appearance. The epidural and prevertebral masses as well as the pachymeningeal involvement have disappeared. Compared to the previous corresponding T1-weighted image, there is an increased signal in the affected vertebral bodies, the expression of medullary fat replacement indicative of healing
7.2.4.4 Etiological Diagnosis
Although imaging in general, and MR imaging in particular, cannot diagnose the specific causative pathogen, some findings suggest certain infections and may support the specific diagnosis (Sharif et al. 1990; Tyrrell et al. 1999; Leone et al. 2000; Ledermann et al. 2003; Jevtic 2004; Tins and Cassar-Pullicino 2004; Tali and Gültekin 2005; Pourbagher et al. 2006).
Pyogenic spondylitis most commonly involves the lumbar spine and one spinal motion segment consisting of two vertebrae and the interconnecting soft tissue (Fig. 7.10). Spinal TB, which most commonly involves the thoracolumbar junction, is characterized by subligamentous spread of infection, paravertebral and intraosseous abscesses, and dural (pachymeningeal) involvement; all these signs are best demonstrated on post-contrast fat-suppressed T1-weighted images (Figs. 7.9 and 7.11). Similar to pyogenic spondylitis, the classical appearance of spinal TB is two adjacent vertebrae collapsed anteriorly with destruction of the intervening disk. Extension of TB from the vertebra and disk to adjoining ligaments and soft tissues is seen frequently and usually occurs anterolaterally. Furthermore, because of subligamentous spread (beneath the anterior longitudinal or below the posterior longitudinal ligaments), multiple noncontiguous vertebral levels may be involved, manifested as skip lesions of vertebral body or posterior neural arch destruction (Figs. 7.7 and 7.11). Epidural inflammatory tissue, which may cause neural compromise, is demonstrated by thecal sac displacement and spinal cord distortion. The associated paraspinal abscesses may vary from small, single, rounded soft tissue swelling, often poorly delineated to extensive and often bilateral paraspinal masses (Fig. 7.9). In the lumbar spine, the sheaths of the psoas muscles may become visibly distended, while the abscesses associated with cervical lesions are evident as retropharyngeal swellings (Burrill et al. 2007). These lesions subsequently may undergo contraction and sometimes calcification as activity of the infection subsides. Calcification within a large paraspinal abscess is virtually diagnostic for TB, but MR imaging is less sensitive than radiography or CT for identifying paraspinal calcifications (Hong et al. 2009). Small and noncalcified paraspinal mass and paravertebral new bone formation in the early healing phase are strongly suggestive of a pyogenic lesion. The classical appearances of spinal TB are thoracic site, multiple levels of infection, presence of skip lesions, relative preservation of the intervertebral disk and subligamentous spread of infection, a large paraspinal mass containing calcifications, and the absence of reactive sclerosis. Etiological diagnosis is a more serious problem when a less typical pattern of TB is found. Erosions and destruction of pedicles or transverse processes may resemble metastatic carcinoma (Fig. 7.7) (Narlawar et al. 2002). Tuberculous involvement of a single vertebral body or of posterior elements, although occurring less frequently, has been well documented (Gillams et al. 1996). Finally, imaging (fluoroscopy, CT) may guide the collection of specimens from the infection focus. This might be indicated to select the appropriate antibiotics (Nagashima et al. 2010).
7.2.4.5 Nuclear Medicine
The role of scintigraphy in detection of bone pathology prior to detectable abnormality on radiography is well recognized. Technetium-99m-methylene diphosphonate (Tc-99m MDP) bone scintigraphy reveals areas of involvement as foci of increased activity. Its sensitivity is very high (>90 %), but the specificity is low (78 %), also causing false-positive in patients affected by other active diseases, including neoplastic and degenerative spine disorders and osteoporotic fracture (Duszynski et al. 1975; Palestro and Love 2007). Furthermore, Tc-99m MDP scan remains active until complete healing takes place and may remain active after infection has become quiescent due to its sensitivity to bone remodeling and repair. Gallium-67 scintigraphy is a valuable adjunct to bone scan, and when combined, they have a sensitivity of 90 %, a specificity of 100 %, and accuracy of 94 %. The use of indium-111-leucocyte scan is not recommended due to poor sensitivity, with spondylodiskitis lesions often displaying nonspecific photopenic regions (Palestro et al. 2009). Fluorine-18-fluorodeoxyglucose positron emission tomography (FDG-PET), possibly combined with CT, has been suggested as an adjunct to imaging in patients with spondylodiskitis (Gemmel et al. 2010; Ohtori et al. 2010). The sensitivity and specificity of FDG-PET in detecting spondylodiskitis is very high when used in addition to conventional tests and imaging. The main limitation remains the differentiation between infection and tumor, since FDG is also a nonspecific tracer.
7.3 Peripheral Osteomyelitis
Osteomyelitis, which infers suppurative inflammation of bone and marrow, can result from the hematogenous spread of organisms from remote sources or by a direct spread of organisms locally (Waldvogel et al. 1970). Hematogenous osteomyelitis typically affects the metaphyses of tubular bones in children (Mader and Calhoun 2000; Resnick 2002). Osteomyelitis in adults is usually caused by direct spread of organisms locally. In adults, the growth plate has resorbed, and the infection may extend to the joint spaces, as in infants, but the periosteum is firmly attached to the underlying bone, so that subperiosteal abscess formation and intense periosteal proliferation are less frequent. The infection, however, can erode through the periosteum forming a draining sinus tract (Mader et al. 2003). Direct spread to the bone can occur from a contiguous source of infection (e.g., skin, soft tissue, joint) or direct implantation of pathogens (e.g., penetrating wound, surgery). This route is likely in patients with peripheral vascular disease, in diabetic patients with neurotrophic plantar ulcers, in bedridden patients with decubitus ulcers, and after trauma. Posttraumatic osteomyelitis can also result from nosocomial infection from the treatment of trauma that allows organisms to enter bone, proliferate in traumatized tissue, and cause subsequent bone infection (Mader et al. 1999).
The elderly are predisposed to osteomyelitis because of surgical procedures that are frequently performed in the elderly population (e.g., dental extractions, open-heart surgery, and prosthetic joint replacement) or because of an increased incidence of systemic disorders that predispose to osteomyelitis (e.g., peripheral vascular disease and diabetes mellitus) (Yoshikawa 1992; Cunha 2002). Depending on both the virulence of the organism and the immune response mounted by the host, osteomyelitis may be acute, subacute, or chronic, but there is no consensual agreement on the temporal scale and no clear distinctions between the three stages are possible (Pineda et al. 2009). Acute osteomyelitis is usually acquired hematogenously, and the most frequently offending organism is Staphylococcus aureus. Subacute osteomyelitis is felt to be a result of a more indolent infection. In the elderly population, it is most commonly due to vertebral osteomyelitis or osteomyelitis associated with prosthetic joint replacement. Chronic osteomyelitis may be defined as osteomyelitis that has a duration of more than 6 weeks or as osteomyelitis that occurs when there is a residual nidus of infection and a refractory clinical course (Resnick 2002). The most common problem in elderly persons is chronic osteomyelitis due to peripheral vascular disease or diabetes mellitus. The pathogenesis of osteomyelitis is multifactorial. Some important factors include virulence of the infecting organism(s), underlying disease and the immune status of the host, and the type, location, and vascularity of the bone (Mader et al. 2003).
The infection arises as the organisms spread into the perivascular interstitial structures. At this stage, microscopy reveals an infiltration of neutrophils and edema. The infiltration leads to vascular compression and impaired blood supply; together with bacterial toxins, the infiltration ultimately causes tissue necrosis and breakdown of the bone trabeculae. If the area of dead bone is small, the necrotic tissue may be resorbed by the action of granulation tissue developing at its surface and replaced with new bone. Necrotic cancellous bone, in localized osteomyelitis, is usually absorbed. Some of the dead cortical bone is gradually detached from living bone to form a sequestrum that acts as a foreign body, and the infection develops from an acute condition into a chronic one. In this case, a periosteal reaction acts to circumscribe the sequestrum producing an encasing sheath of new bone (involucrum) surrounding the dead bone under the periosteum (Jain et al. 1995). Involucrum is often perforated by openings (cloacae) through which pus may track into the surrounding soft tissues and eventually drain to the skin, forming a draining sinus tract. Cloacae are caused by rupture of the periosteum due to pus under pressure (Mader et al. 2003). The presence of necrotic bone is the hallmark of chronic osteomyelitis; common features of this condition include involucrum, local bone loss, and sinus tracts. Occasionally, the disease does not form sequestra; acute osteomyelitis may be contained to a localized, purulent, and necrotic area and surrounded by fibrous tissue and granulation tissue. This specific form of osteomyelitis that can occur in either subacute or chronic infection is termed Brodie abscess. Typically, it is seen at the extremities of the long bones, in young men, with 75 % of patients under 25 years old (David et al. 1987; Bancroft 2007). The diagnosis of osteomyelitis is made on the basis of clinical, laboratory, and imaging examinations. A history of past medical illness, treatment, and any physical evidence of trauma or previous surgery is valuable. Clinically, the patient complains of pain, fever, local swelling, and sometimes hotness and redness at the site of infection. Certain laboratory tests, such as the white blood cell count, ESR, and C-reactive protein, may be helpful as a baseline during treatment. Imaging is essential to confirm the presumed clinical diagnosis and to provide information regarding the exact site and extent of the infectious process. The reference standard is biopsy for histopathology and microbiological evaluation.
7.3.1 Imaging of Peripheral Osteomyelitis
If osteomyelitis is suspected clinically, radiography remains the initial imaging procedure. Although radiographs often appear normal during the first 1–2 weeks, they provide an anatomic overview of the region of interest and are valuable for the exclusion of other diagnostic possibilities such as tumors. The first radiographic sign of osteomyelitis is often soft tissue swelling causing obliteration and blurring of the adjacent fat planes. Approximately 10 days after the onset of infection, radiographs may demonstrate lysis of medullary trabeculae. Type and extent of medullary lysis vary from a solitary radiolucency to irregular multiple radiolucencies (mottling) and homogeneous osteopenia (Fig. 7.12). The lesions are generally indistinct and irregularly outlined. Cortical destruction, associated with lamellated periosteal reactions, appears later as small holes that coalesce into lesions and finally progress to completely involve a region of cortex. The reparative phase under therapy is characterized by endosteal and periosteal new bone formation, development of a surrounding sclerosis, and even large osteosclerotic areas (Fig. 7.13) (Palestro 1994). The specificity of radiography for the detection of osteomyelitis (from 75 to 83 %) is higher than its sensitivity (from 43 to 75 %); thus, a negative study does not exclude osteomyelitis (Boutin et al. 1998). For this reason, radiography should be routinely supplemented by MR imaging in all patients suspected of having osteomyelitis. The standard MR imaging protocol should routinely include T1-weighted and fluid-sensitive sequences which are highly sensitive for early inflammatory edema. Contrast-enhanced MR images are least useful in cases in which no evidence of inflammation is present on unenhanced MR images. MR imaging has a 100 % negative predictive value for excluding osteomyelitis; thus, infection can be reliably excluded if the marrow is completely normal on T1-weighted and fluid-sensitive images. Contrast-enhanced imaging is most useful in determining the presence or absence of abscesses (enhancement of the granulation tissue in the abscess wall but not of the fluid within it) (Fig. 7.14), sinus tracts (lined by granulation tissue) (Fig. 7.15
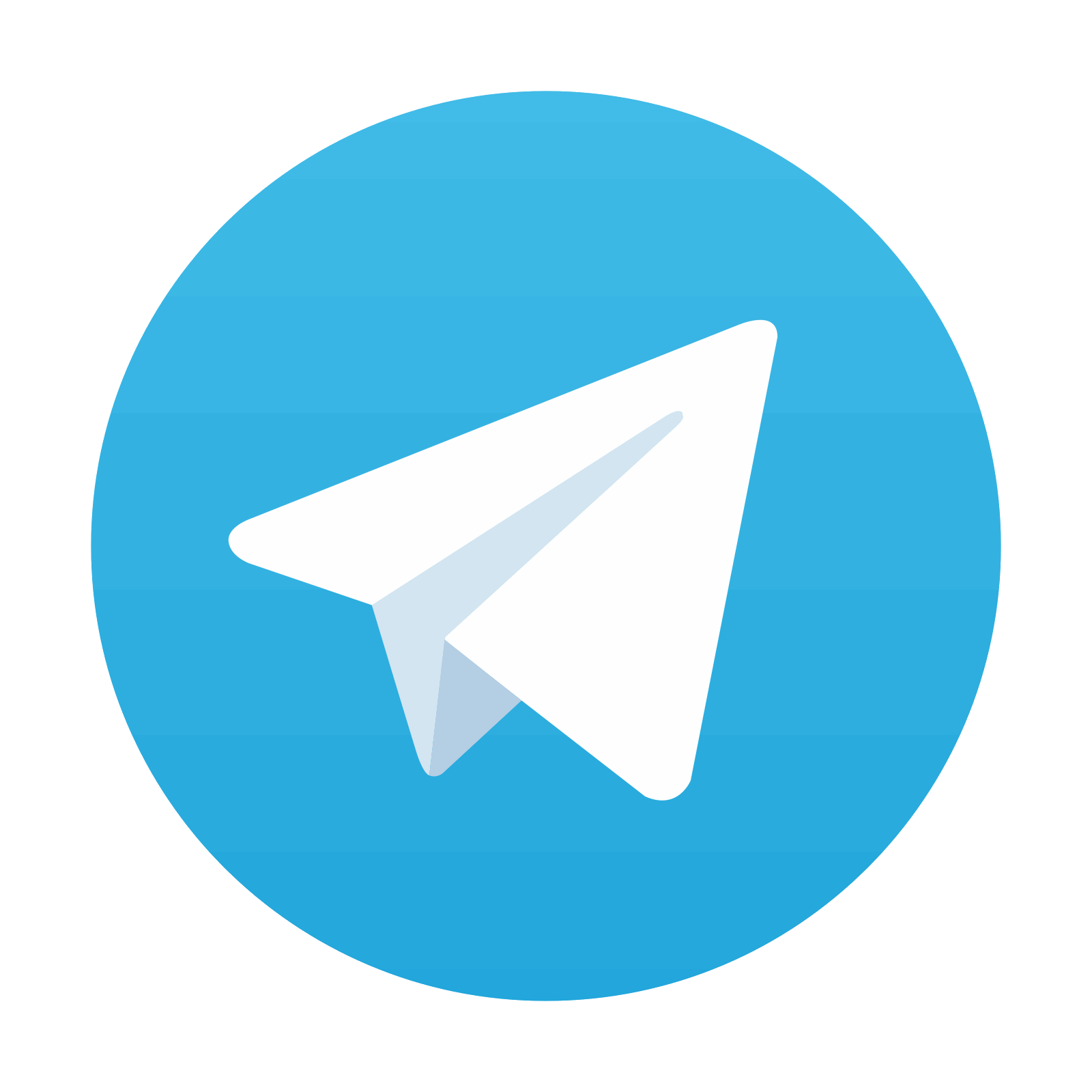
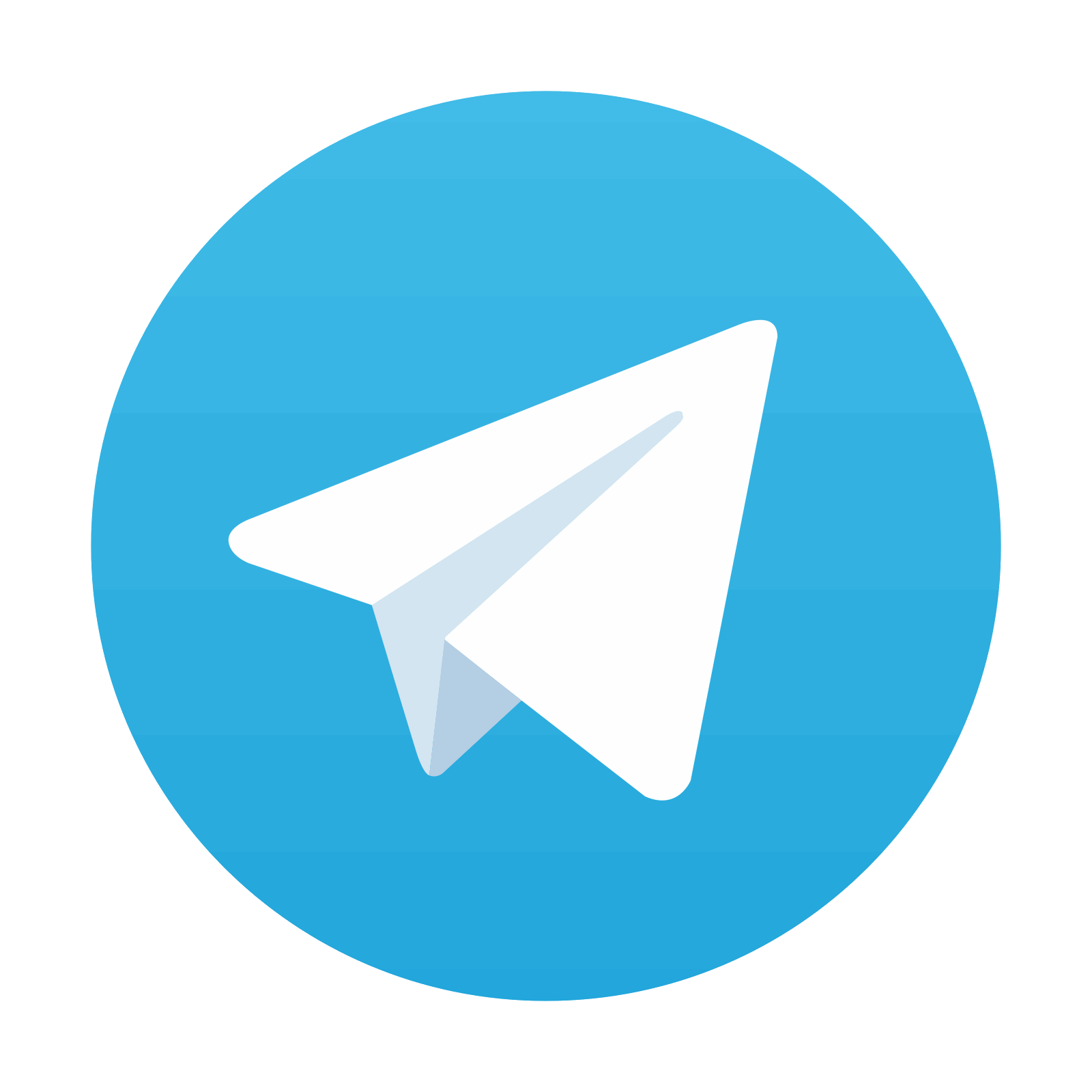
Stay updated, free articles. Join our Telegram channel

Full access? Get Clinical Tree
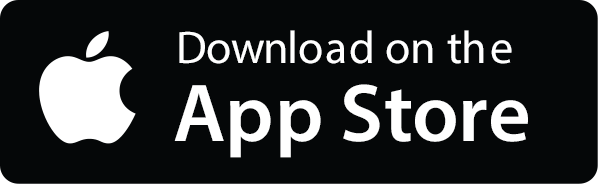
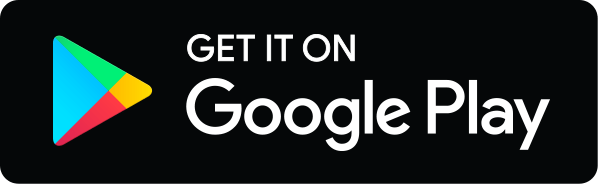