

Table 11-1 Selected Congenital and Perinatal Infections | |||||||||||||||||||||||||||||||||
---|---|---|---|---|---|---|---|---|---|---|---|---|---|---|---|---|---|---|---|---|---|---|---|---|---|---|---|---|---|---|---|---|---|
|
studies can show thrombocytopenia, direct hyperbilirubinemia, and elevated serum transaminases (16). The majority of infants with congenital CMV disease have permanent neurodevelopmental sequelae, including microcephaly, cerebral palsy, cognitive impairment, sensorineural hearing loss, visual abnormalities, and seizures. Sensorineural hearing loss can progress after birth, in both infants with congenital CMV disease and those who were without symptoms at birth (17). By contrast, the vast majority of CMV-infected infants who lack signs of infection, either at birth or subsequently, have normal neurodevelopmental outcomes. Steinlin et al. (18) have described a characteristic clinical syndrome in patients who are infected with CMV during the third trimester. Characteristics of the syndrome include microcephaly with sensorineural hearing loss, hyperactivity and associated behavioral problems, reduced apprehension for pain, and, sometimes, ataxia and hypotonia.
|
described in children with trisomy 13, trisomy 21, prenatal drug exposure, congenital heart disease, and a variety of anoxic and toxic injuries to the developing brain (31,32). Postmortem studies have described evidence of a mineralizing vasculopathy as the cause, whereas other studies suggest altered perfusion, perhaps the result of impaired autoregulation (33,34). Lenticulostriate vasculopathy is a nonspecific finding, and the diagnosis of congenital infection should not be strongly considered unless other supportive findings (parenchymal regions of hyperechogenicity, intraventricular septations, periventricular necrosis [germinolytic cysts], anterior temporal white matter lesions, and/or abnormalities of sulcation) are also seen by fetal imaging (US or MRI) or postnatal US, CT, or MRI (Fig. 11-4) (35,36,37). Antenatal cranial sonography may offer predictive information for the fetus infected with CMV but may underestimate the presence of anterior temporal lobe or temporal polar abnormalities (38). On CT and MR, some patients, presumably infected during the first half of the second trimester, demonstrate agyria or lissencephaly with a thin cortex, cerebellar hypoplasia, delayed myelination, marked ventriculomegaly, germinal zone cysts, anterior temporal lobe cysts, and periventricular calcification (Figs. 11-3, 11-4, 11-5 and 11-6). Those injured later, presumably in the middle of the second trimester, have more typical polymicrogyria, less ventricular dilatation, and less consistent cerebellar hypoplasia; schizencephaly and infoldings of cortical dysplasia can be seen (Figs. 11-6, 11-7 and 11-8) (39,40). Patients infected near the end of gestation or in the early postnatal period have normal gyral patterns, mild ventricular and sulcal prominence, and damaged periventricular or subcortical white matter with scattered periventricular calcification or hemorrhage (Figs. 11-7, 11-8 and 11-9) (12,18,24,28,41,42). FLAIR images, particularly in the first year of life, provide relatively poor contrast between gray and white matter; therefore, T2-weighted images are essential to identify
cortical malformations (Figs. 11-6 and 11-7), the detection of which helps establish the diagnosis of congenital CMV.
detected on CT than on MR in older infants and children. Although dogma has suggested that congenital CMV CNS infection is predictably associated with cerebral calcifications, the prevalence of calcification may be less than 70%. Differentiation of calcification from hemorrhage may be difficult by either CT or MRI (24). Including susceptibility-weighted imaging (SWI) and SWI filtered phase maps into the routine MR examination aids in the differentiation of SWI hypointensities of blood products (paramagnetic) from calcifications
(diamagnetic). The SWI filtered phase map of calcifications is hyperintense (Fig. 11-10) (44). Clinicians should recognize that calcification is not uniquely specific for congenital infection, as any injury to the brain, including those caused by ischemia, genetic syndromes, metabolic errors, and nonspecified neurodegeneration, can cause dystrophic calcification (45,46,47). MR is the imaging examination of choice for the detection of cortical malformations, myelin abnormalities (delay and demyelination), germinal zone cysts, and cerebellar hypoplasia/dysplasia. When these features are present in a child with microcephaly, developmental delay, sensorineural hearing loss, and seizures, a diagnosis of congenital CMV should be considered (24).
of infants with neonatal HSV encephalitis will have negative HSV PCR studies (52). Therefore, a negative result should be interpreted with caution. If there is a clinical suspicion of HSV meningoencephalitis, a second lumbar puncture should be performed and acyclovir administered empirically until the results of the second PCR are known. Therapy of neonatal HSV CNS infections consists of high-dose (60 mg/kg/day) acyclovir for 28 days (54). Infants who survive perinatal HSV infections are at high risk for cerebral palsy, epilepsy, and developmental delay despite aggressive acyclovir therapy (49,52). Suppressive antiviral therapy for 6 months after the initial treatment substantially improves the long-term outcome of neonatal HSV infections (55).
elevated lactate and, often, reduction of N-acetyl aspartate (NAA) in affected regions (Fig. 11-12) (61,63). After 1 or 2 days, CT and MR show patchy, multifocal areas of injury (low attenuation on CT, T1 hypointensity, T2 hyperintensity, and regions of reduced diffusion), affecting gray and white matter (Figs. 11-11, 11-12 and 11-13), which progresses in prominence and extent of involvement during the course of the next several days. Hemorrhage is a common finding in neonatal herpes encephalitis, seen in up approximately two-thirds of patients (Fig. 11-14) (63). Contrast enhancement, although minimal, occurs early in a meningeal pattern (Fig. 11-14) (63). Near the end of the first week of disease, there are often regions of cortical gray matter injury (increased attenuation on CT, T1 hyperintensity, and T2 hypointensity on MR) that persists for weeks to months(61,63,67). Loss of brain substance occurs rapidly, often as early as the second week. Eventually, severe, diffuse cerebral atrophy ensues with profound cortical thinning and encephalomalacia; in the end stage, the brain often appears multicystic (Fig. 11-15E). Punctate or curvilinear gyral calcifications may also be seen as a late finding. The cerebellum is injured in about half of the affected patients (61,63,67).
(see Chapter 5) and toxoplasmosis. Hydrocephalus, seen in more than 50% of affected neonates, results from necrotizing ependymitis and aqueductal obstruction (70,71). Other infants with congenital LCMV virus infections can have microcephaly and intracranial calcifications (70,71). Seizures commonly develop during the first year of life. Longterm outcome is generally poor, with mortality as high as 35% and severe neurologic sequelae in more than 60% of survivors (71).
of the world, with more than 100,000 cases estimated by the Centers for Disease Control and Prevention (CDC) to have occurred worldwide annually during the first decade of the current millennium. Humans represent the only reservoir of rubella virus, and transmission results from contact with virus-contaminated respiratory secretions (76).
to a more variable outcome; on CT, sparse (Fig. 11-21) or multiple periventricular calcifications and ventricular dilation are typical findings (Fig. 11-20). Infection after the 30th week of gestation is generally associated with mild clinical and imaging abnormalities, with CT findings of small periventricular and intracerebral calcifications that are only rarely accompanied by ventricular dilatation (104). An important differentiating feature between congenital toxoplasmosis and congenital CMV infection is the absence of cortical malformations in toxoplasmosis (a common occurrence in congenital CMV). The brain calcifications of congenital toxoplasmosis may resolve slowly in some infants after antitoxoplasma therapy (106). Therefore, the disappearance of calcification over time should not cause confusion if the diagnosis was established at birth on the basis of characteristic ocular findings and serologic testing.
opportunistic infections with T. gondii, cytomegalovirus, varicellazoster virus (VZV), Mycobacterium tuberculosis, fungi, and JC virus, the cause of progressive multifocal leukoencephalopathy (PML) (131).
associated encephalopathy; therefore, imaging studies of the spine in children with HIV myelopathy are uncommon.
Unlike previously discussed infections such as CMV, which reflect a static pattern of brain injury and/or malformation, the infant and young child with ACS exhibit progressive neurodegenerative clinical and neuroimaging findings.

of all ages in the United States and other regions with widespread use of the Hib vaccine and newer vaccines for both S. pneumoniae and N. meningitidis (167). Nontypeable Haemophilus influenzae or non-b subtypes have emerged as clinically important agents causing meningitis among healthy or immunocompromised children in many regions, including those with compulsory immunization against H. influenzae (Table 11-4) (4,174,175,176). Subtypes of H. influenzae remain important causes of bacterial meningitis with subtype F representing an aggressive, regional, circum-polar infection with reports often originating in remote northern latitudes. Subtype A and nontypeable H. influenzae are often associated with milder cases of meningitis. Among unimmunized children, H. influenzae meningitis occurs more commonly in persons with sickle cell anemia, asplenia, and HIV infection, and generally in older infants. Other risk factors for S. pneumoniae meningitis also include nephrotic syndrome, cochlear implantation, and CSF leaks. Unimmunized college students and persons with inherited complement deficiencies have an increased risk of contracting N. meningitides meningitis. Children or adolescents with congenital or acquired immunodeficiencies, including disorders of cell-mediated immunity or granulocyte function, can experience meningitis due to Pseudomonas aeruginosa, Staphylococcus epidermidis, Staphylococcus aureus, Listeria monocytogenes, and enteric organisms (174). Meningitis also represents one of the most common extrapulmonary manifestations of infection with Mycobacterium tuberculosis (177).
Table 11-3 Selected Causes and Imaging Features of Neonatal Bacterial Meningitis | ||||||||||||
---|---|---|---|---|---|---|---|---|---|---|---|---|
|
Table 11-4 Common Causes of Bacterial Meningitis in Infants, Children, and Adolescents | ||||||||||||||||||||||||||||||||
---|---|---|---|---|---|---|---|---|---|---|---|---|---|---|---|---|---|---|---|---|---|---|---|---|---|---|---|---|---|---|---|---|
|
controls, neonates with meningitis have increased FA values in the LCSWM, likely reflecting the presence of inflammatory adhesion molecules within the subarachnoid space (192).
is uncertain, or a complication of meningitis is suspected (Fig. 11-37). Hydrocephalus complicating meningitis indicates altered CSF circulation and/or resorption, which may occur at several levels (Fig. 11-38) (4,6,194), reflecting the intense inflammatory reaction and purulent exudates within the interventricular communications pathways, basal cisterns, sulci, and perivascular spaces (Figs. 11-37 and 11-38).
partition size (158) (see discussion on the following page). This observation is most easily made on midline sagittal images encompassing the sagittal sinuses and straight sinus/vein of Galen complex and on parasagittal images for the transverse and sigmoid sinuses. Other than in the subacute phase, the MR diagnosis of sinus thrombosis is difficult. Pronounced hypointensity seen in a venous sinus on sagittal T1-weighted images or in the superior sagittal sinus on coronal FLAIR images mitigates against thrombosis. However, in the absence of pronounced hypointensity on T1-weighted images, the patency of the sinus remains uncertain on routine MR images (Figs. 11-39B and 11-40A). The acutely thrombosed sinus is isointense to the brain on T1-weighted images and hypointense to the brain on T2-weighted images. This appearance cannot be distinguished from slow flow or pseudogating (in which the sinus is imaged during diastole). In this situation, evaluating the SWI images and reformations is very helpful to look for the hypointensity (paramagnetic effect) of thrombosis. Overall, as mentioned above, intravenous contrast-enhanced 3D MRV techniques are superior in the detection of cerebral sinovenous thrombosis as well as cortical and bridging vein thromboses (Figs. 11-40E and 11-41B) (158). In the clinical setting where contrast is contraindicated, robust noncontrast time-of-flight (TOF) 3D MRV contributes useful information.
from sagittal sinus thrombosis are parasagittal (Fig. 11-40); infarcts from thrombosed internal cerebral veins, straight sinus, and vein of Galen involve the thalami. Infarcts from vein of Labbé, transverse sinus, or sigmoid sinus thrombosis involve the temporal lobe. Smaller cortical/subcortical infarcts are often seen over the cerebral convexities (Fig. 11-41). On CT, venous infarcts are usually poorly delimited, hypoattenuating, or mixed attenuation areas involving the subcortical white matter and producing a slight mass effect on ventricular structures. The low attenuation is probably due to localized cerebral edema (vasogenic and cytotoxic), whereas high attenuation areas within the brain parenchyma usually represent hemorrhage. Following intravenous contrast administration, a lack of expected venous opacification is seen (venous clot may be detected), adjacent sulcal/gyral enhancement is noted, and adjacent parenchymal hypoattenuation (edema) is appreciated (209). On MR, early venous infarcts may be identified by visualization of prolonged T1 and T2 relaxation times in characteristic regions of the brain parenchyma, SWI hypointensity within the adjacent venous structure(s), and low ADC values in regional parenchyma (Fig. 11-41). Another early and important imaging sign of venous infarction is visualization of thrombus in the deep medullary veins with surrounding cavitation. Of note, reduced parenchymal diffusivity in the setting of venous thrombosis lacks sensitivity in predicting venous infarction. With venous ischemia and infarcts, diffusion appears to be heterogeneous, with areas of increased, normal, and decreased diffusion (Fig. 11-41) (210). The variable diffusion characteristics are likely related to the combination of interstitial and cytotoxic edema found in venous infarcts. In addition, part of this heterogeneity may be due to the frequent presence of hemorrhagic tissue, which makes diffusivity values unreliable. Twenty-five percent of venous infarcts are hemorrhagic and have an imaging appearance that varies from large subcortical hematomas to petechial hemorrhages admixed with edematous brain parenchyma (Fig. 11-40) (207,209). The hemorrhages are generally subcortical and often multifocal with irregular margins. They are occasionally linear in nature, indicating hematoma in and around the vein; this appearance is quite specific.
or predominant lymphocytic pleocytosis, elevated protein (usually between 100 and 500 mg/dL), and mildly reduced glucose (234). The diagnosis of tuberculous meningitis can be established by PCR detection of mycobacteria in the CSF and supported by a positive tuberculin skin test or detection of interferon-gamma release in whole blood samples exposed to M. tuberculosis antigens (232,234). Treatment consists of combined antituberculous therapy with a four-drug regimen and administration of corticosteroids; even with therapy, death or significant morbidity is possible, especially when treatment is delayed.
causing infarction of surrounding brain tissue. Outcome is directly related to the location and extent of these infarctions (238). The basal ganglia and thalami are affected in almost half of cases (239,240); suprasellar cisternal inflammation and involvement of the posterior hypophysis may lead to the syndrome of inappropriate antidiuretic hormone secretion (241). The thick exudate blocks the subarachnoid spaces, causing hydrocephalus. Infiltration of the perineurium of the cranial nerves causes neuropathies, particularly of cranial nerves II, VI, and VII. Small tubercles over the convexity of the brain may involve the leptomeninges, while deeper lesions may infiltrate the periventricular area.
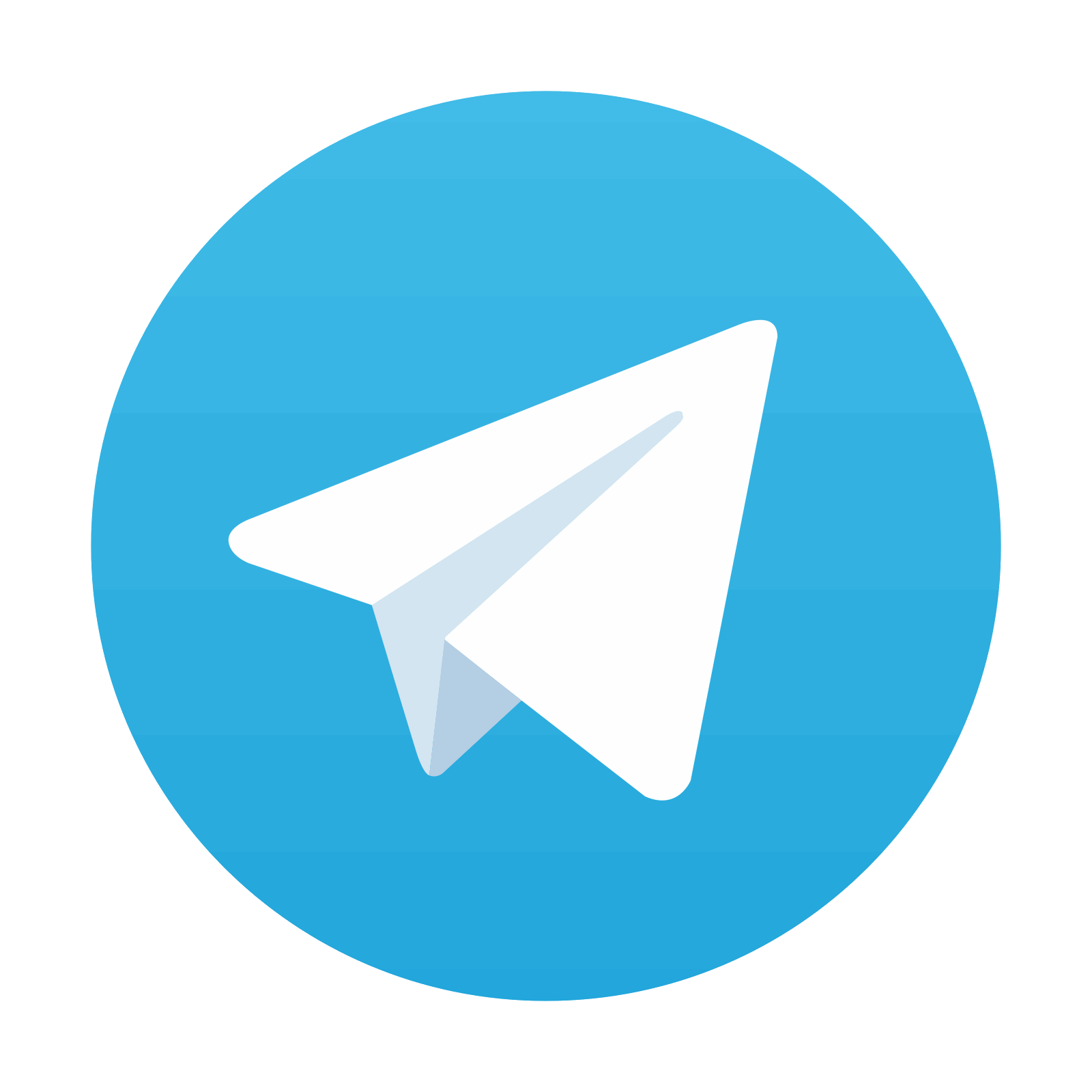
Stay updated, free articles. Join our Telegram channel

Full access? Get Clinical Tree
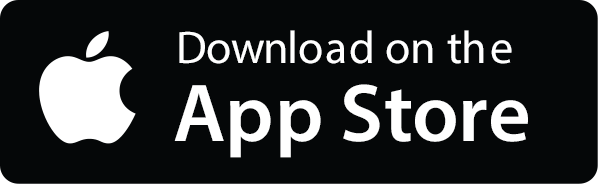
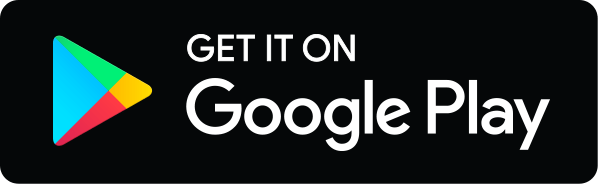
