Cardiac computed tomography (CT), the most highly developed application of x-ray imaging, is currently experiencing more advancement per year than ever in its history—probably more than any other imaging modality in the history of x-ray–based medical imaging.
The Road to the Present
X-Rays
Wilhelm Conrad Roentgen was awarded the first Nobel Prize in Physics in 1901 for the first intentional generation and use of x-rays, in 1895. He was a firm academic traditionalist, and, intending to preserve his scientific integrity, he signed away all commercial rights to his invention. His name lives on as one of many units of (medical) radiation. His descriptions of the physics of his novel form of “rays” were so definitive that they still constitute a large proportion of the theory of x-ray radiation.
Angiography and Catheterization
The first cardiac catheterization performed may be the one done by Claude Bernard in 1844 on a horse. The first human cardiac catheterization was performed in 1929 by a surgery resident, Werner Forssmann, on himself. Using his right hand, Forssmann blindly passed a urethral catheter up the basilar vein in his left arm. He then walked down to the floor below and used, by himself, an x-ray fluoroscopy unit, which enabled him to determine that the catheter was not within the heart. He then advanced the catheter further until he confirmed that it was indeed within the right ventricle, thereby affirming Law Number Six from Samuel Shem’s The House of God that “there is no body cavity that cannot be reached with a #14G needle and a good strong arm.” Forssmann then documented the position of the tip of the catheter using radiographs. He was rewarded for his feat by being fired from the training program, but he ultimately was awarded a Nobel Prize in Medicine, nearly three decades later, in 1956.
The first human angiogram probably was performed on the excised hand of a cadaver, with injection of an empiric contrast-enhancing formula of heavy metals and petroleum jelly into the hand vessels.
The first coronary angiogram was inadvertent. In 1958, Mason Sones, the great pioneer of coronary angiography, was performing a cardiac catheterization on a 26-year-old man with rheumatic aortic and mitral valve disease. The aortic root catheter inadvertently fell into the right coronary ostium, resulting in a powered injection of 50 mL of contrast dye, intended for the aortic root, into the right coronary artery. The patient was asystolic for 5 seconds, but recovered with bradycardia. Purely by chance, angiography was shown to be feasible and tolerable and to yield clinically useful images ( Fig. 1-1 ).
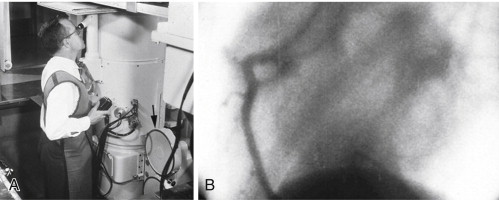
Sones, Seldinger, Judkins, and others pioneered many developments to enable peripheral arterial access, selective angiography, adequate image intensifiers, and development of rotating gantries that allowed first left-right rotation and later craniocaudal rotation.
In 2012, approximately 600,000 angiograms were performed in Germany, approximately 150,000 in Canada, and nearly 2 million in the United States, with an overall complication rate of 3.6% and a mortality rate of 0.1%. The average annual cost of coronary angiography in the United States exceeds $9 billion. The yearly worldwide number of coronary angiograms performed is unknown. Angiography remains the current standard for imaging of coronary artery stenosis, delivery of percutaneous coronary intervention, and determination of surgical bypass amenability. Cardiac CT is vying to offer accurate noninvasive angiography; it is a bold prerogative.
Cross-Sectional Imaging and Computed Tomography
Conventional x-ray imaging, planar or angiographic, is superimposition-based imaging and often provides a challenge to the interpretation of radiographs and the performance of angiograms.
The development of cross-sectional two-dimensional imaging began more than four decades ago. The first CT scanner, the brainchild of Sir Godfrey Hounsfield, became available in 1971. The first patient was scanned during the following year. This CT scanner system was able to scan only a small structure, such as a head, but within a decade, enlargement of the scanner gantry would allow whole-body scanning. Limited by the computer processing speed available at that time, generation of an image after scanning required almost an entire day. Within a year commercial development of the device began. The system launched a new era in cross-sectional diagnostic imaging that has helped in the management of innumerable patients with medical and surgical diseases and trauma. Hounsfield was awarded the Nobel Prize in medicine in 1972. His name lives on in the field of CT as a unit of attenuation of CT scanning—the Hounsfield unit (HU).
In 1973, at the Mayo Clinic, Erik L. Ritman developed the dynamic spatial reconstructor (DSR), a device that could generate a large number of image slices with improved temporal resolution. Standing more than a story tall and using 14 x-ray tubes that took 3 seconds to rotate all the way around the patient, the DSR’s enormous size is often cited as a turning point in the development of engineering advances of CT technology, not the least of which was achieving economies of space and radiation.
Early development wrestled with both the basics of the engineering suitable to this novel form of imaging and the processing of the data obtained to generate images. CT scanner designs have gone through four generations thus far, with each generation obtaining images by different positioning and sequencing of the beam-forming and beam-detecting units.
Electron beam CT (EBCT) scanning is heavily represented in medical literature, but it has largely outlived its clinical use. EBCT systems did not use any parts that moved around the patient. An electron beam from an x-ray source (“gun”) was focused by coils, then deflected by magnetic fields generated by other coils, and deflected again off a crescentic array that directed the beam across the patient’s body at different angles, resulting in “slices,” typically 3 mm thick. The system was unique for having no moving parts (no gantry), in contrast to current mechanical CT systems, which have an enormous amount of sophisticated equipment rotating at high speed around the patient during scanning. Few companies manufactured EBCT systems, and few systems have been produced in the last decade, but many machines are still operational. Despite its outdated qualities, EBCT had relatively good temporal resolution and very good spatial resolution and constituted a notable development and advancement in image quality. EBCT is most significant for its contribution to the medical research in noninvasive imaging and cardiac disease by coronary artery calcium scoring. However, EBCT yielded large slices (1.5–3 mm), was quite susceptible to image noise and artifacts, and could acquire only one (or two at the most) slices per breath-hold. Scan times were problematically long, taking 40 to 50 cardiac cycles. Although it briefly offered imaging superior to that of mechanical scanning, which was in its infancy at the time, EBCT soon was technologically surpassed for cardiac imaging by mechanical scanners. The ongoing representation of EBCT in the medical literature (generally in the form of well-performed clinical series at one to two decades of follow-up to calcium studies performed in the early 1990s) has led to some confusion about its current standing.
By the early 1990s, CT scanners had evolved from acquiring a sequence of scan slices through the field of interest (i.e., sequential scanners) to rotating around the patient while he or she was simultaneously passed through the scanner. CT systems defined as rotational, spiral, or helical became, and remain, the norm.
The basic components of CT systems developed steadily over three decades. Single-detector single-slice CT (SSCT) evolved to multiple-detector CT (MDCT) and multislice CT (MSCT) by 2000 or, simply, cardiac CT (CCT). Other key developments include:
- □
X-ray tubes that could produce large volumes of optimal uniform quality output with very good temporal responsiveness
- □
Collimeters to adequately control beam width and reduce the radiation emission to the minimum essential for image acquisition
- □
A gantry housing the x-ray tube and the detectors that could be moved with sufficient control and variable speed and had the means to offload the large and progressively increasing amount of information from the gantry at extreme speed
- □
Tables that could feed the patient into the gantry at precisely controllable speeds, thereby rendering image acquisition helical or spiral as a Z-plane was added to the X- and Y-planes of images to render acquisition volumetric
- □
Detectors of sufficient width to enable imaging of the heart in one rotation (256- and 320-MDCT) and detectors of superior quality (garnet-based rather than gadolinium-based)
- □
Software advances made it possible to:
- •
ECG “ gate” image acquisition to the cardiac cycle
- •
Allow image display along non-planar lines to follow curving cardiovascular structures along their own long axis
- •
Generate different perspectives on structures by means such as 3D volume rendering and endoluminal displays
- •
Process hundreds to thousands of images for morphologic and functional evaluation of cardiac chambers and valves
- •
- □
Workstations to facilitate the interface of all of this new technology.
Although much CT technology developed steadily, the last decade has seen markedly accelerated development. Within a period of just 5 years, CT developed from single-slice to 2-, 4-, 8-, 16-, 32-, 40-, and then 64-slice imaging, which is the current standard. Subsequent development yielded 128- (briefly), 256- (128 × 2) and 320- (128 × 2 + 64 to give 10 cm of coverage) slice scanners, to begin an era of single cardiac cycle acquisition (in optimal circumstances).
Numerous improvements have lowered radiation exposure by more than a logarithm. Now that MDCT has acquired greater spatial and temporal resolution, it has become capable of cardiac imaging and more capable of coronary imaging. Interest and involvement by cardiologists in participation and partnership with radiology in cardiovascular CT (CVCT) in the last half decade has increased significantly, and CVCT also has resonated with the media and population at large as a noninvasive means to identify coronary disease.
The novelty of the field of cardiac and coronary CT and its attraction for cardiologists have led to the following, all since 2005:
- □
Formalization and standardization of training requirements and examination certification
- □
CT training within the context of multimodality imaging, clinical competence, and laboratory operational standards
- □
Appropriateness of use of cardiac CT and of integration of structured reporting across cardiovascular imaging
Given the inherent challenges of imaging coronary arteries, because of their small size, considerable motion, tendency to develop atherosclerosis and to attract calcium, and stents—both of which produce problematic artifacts—coronary evaluation is understandably one of the last applications of MDCT to reach fruition. It is not there yet, in terms of having anything nearly equal to conventional coronary angiography across the breadth of clinical permutations of coronary disease, comorbidities, cardiac rhythm, heart rate, body size, and provision of a platform for intervention ( Table 1-1 ).
