Fig. 1.1
Face radiographs. Waters (a) and lateral (b) projection radiographs show multiple radiopaque metallic bullet fragments in the bilateral periorbital soft tissues, which remained after orbital and facial reconstructive surgery
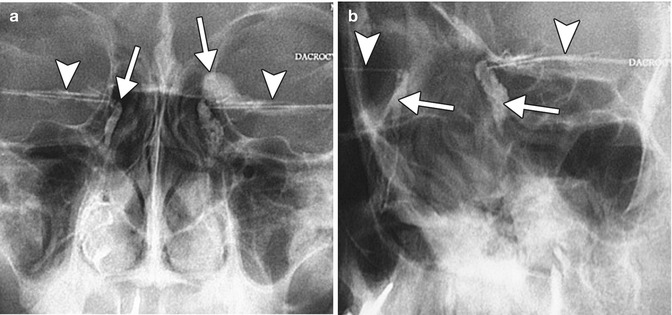
Fig. 1.2
Fluoroscopic dacryocystography. Frontal (a) and oblique (b) radiographic images show bilateral canalicular catheters (arrowheads) and contrast material within the bilateral nasolacrimal system (arrows), but no free spillage into the nasal cavity, suggestive of obstruction
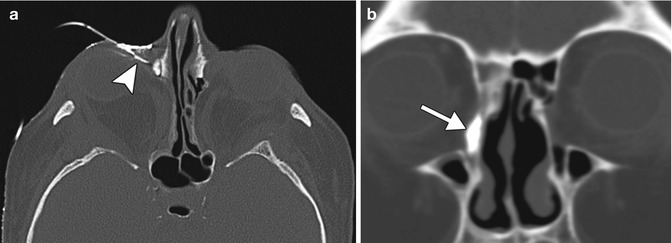
Fig. 1.3
CT dacryocystography. Axial CT image (a) shows the catheter and contrast material within the right inferior canaliculus (arrowhead). Coronal CT image (b) shows contrast within the right lacrimal sac (arrow)
1.3 Computed Tomography
Orbital CT is typically acquired with thin axial sections (0.6–1.0 mm) from which coronal and sagittal reformatted images are generated and displayed in both soft tissue and bone algorithms (Fig. 1.4). Thus, CT is well suited for delineating the osseous structures and the positioning of many types of orbital implants in fine detail. 3D surface renderings can be a useful adjunct for visualization of the implants. Except for stainless steel, tantalum, and certain embolization materials, such as Onyx, orbital implants generally do not produce significant streak artifact. In addition, CT is often obtained in emergency situations due to its rapidity, widespread availability, and ability to safely screen for metallic foreign bodies. Administration of iodinated contrast can be useful for evaluating suspected infectious and inflammatory processes and tumors. Contrast is also necessary for performing CTA and CTV, which may be useful for evaluating the status of carotid cavernous fistulas and other vascular lesions after treatment. However, contrast is not necessary for assessing the positioning of implants. Furthermore, in patients with thyroid eye disease, iodinated contrast can aggravate thyroid orbitopathy.
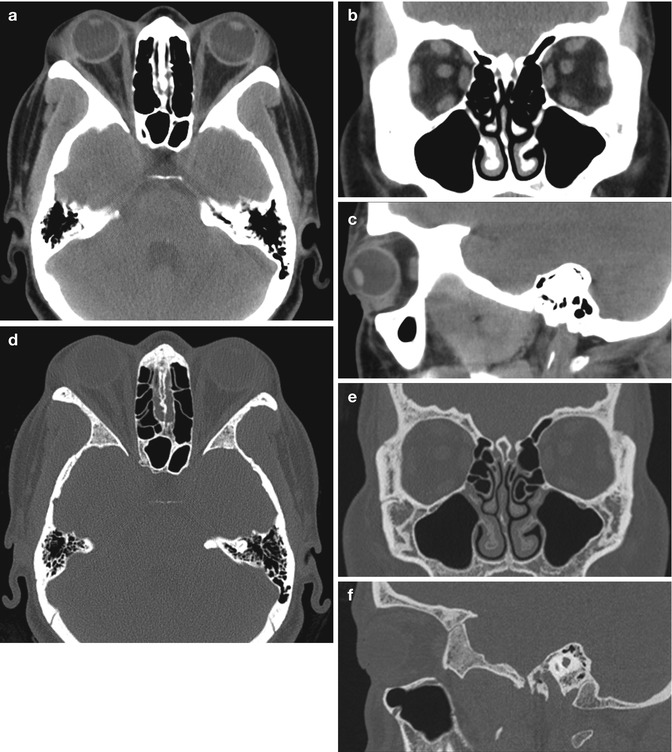
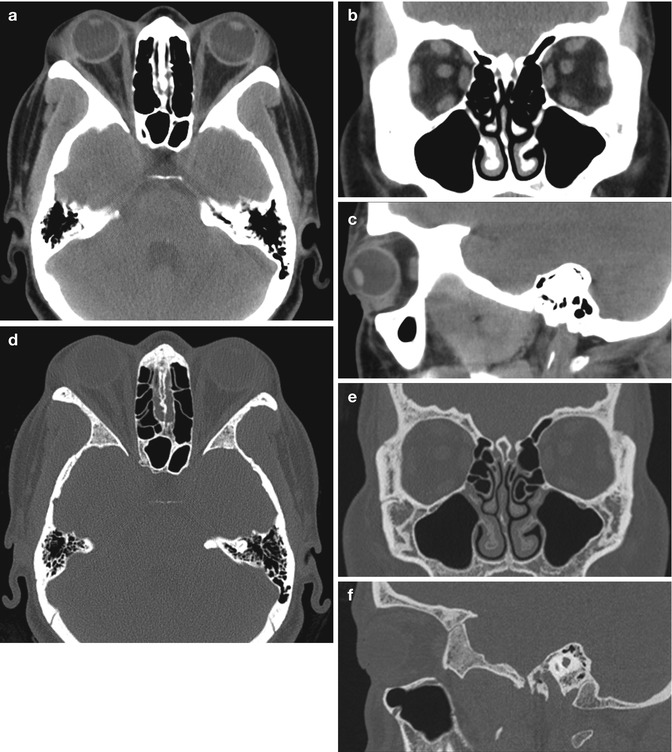
Fig. 1.4
Standard orbit CT images. Selected axial (a), coronal (b), and sagittal (c) soft window images and axial (d), coronal (e), and sagittal (f) bone window images of normal orbits
1.4 Magnetic Resonance Imaging
Compared with CT, MRI offers superior soft tissue delineation and no exposure to ionizing radiation, which is particularly important in the pediatric population. Prior to obtaining a scan, it is important that patients are screened for MRI compatibility. A useful reference for determining whether certain implants are MRI compatible can be found at http://www.mrisafety.com/. Other factors that may limit the application of MRI include severe claustrophobia and obesity exceeding table weight limit. The main sequences obtained in orbital MRI are T1-weighted and T2-weighted sequences in multiple planes, including axial, coronal, and sagittal (Fig. 1.5). Administration of gadolinium-based contrast for T1-weighted sequences is useful for defining infectious and inflammatory processes, as well as residual tumors (Fig. 1.6). Implementing fat-suppression techniques with T2 and post-contrast T1-weighted sequences is also helpful for better defining lesions against the hyperintense background of the orbital fat. Many fat-suppression techniques are prone to failure near the air-filled sinonasal cavities due to susceptibility effects, which may lead to misinterpretation. However, certain techniques such as Dixon and inversion recovery are less prone to such artifacts. The use of high-resolution microscopy surface coils can yield in-plane resolution of 312 μm and the display pixel dimensions of 156 μm even at 1.5 T. As a result, it is possible to obtain a detailed view of the orbital structures and globe, including Tenon’s capsule, tarsal plate, ciliary body, lens zonules, and components of the superior rectus-levator complex. The use of diffusion-weighted imaging in orbital imaging for indications such as monitoring treatment response in tumors remains investigational at the time of this writing. Likewise, the utility of cine (oculodynamic) MRI techniques for depicting the motion of the extraocular muscles in the postoperative setting is promising, but requires further validation. MRA can be used as a noninvasive method for evaluating patients after stenting and/or embolization of vascular lesions. Time-resolved MRA provides a higher quality imaging of the treated parent vessels compared with time-of-flight imaging.
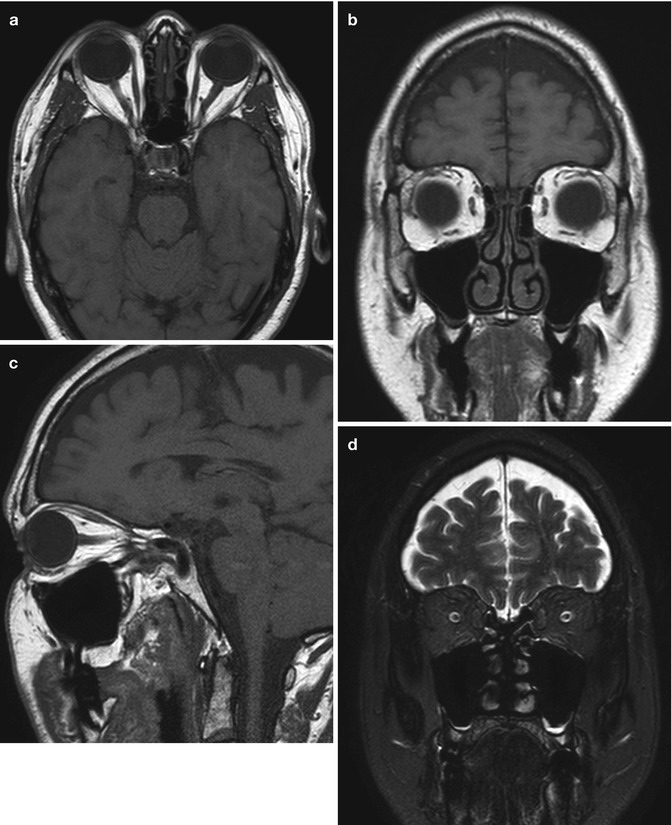
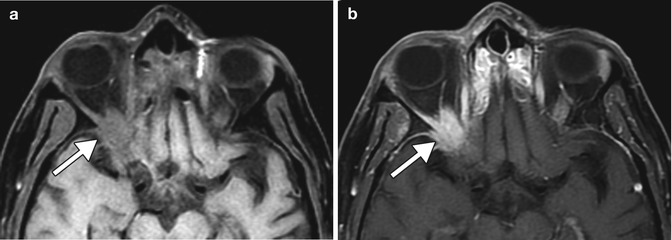
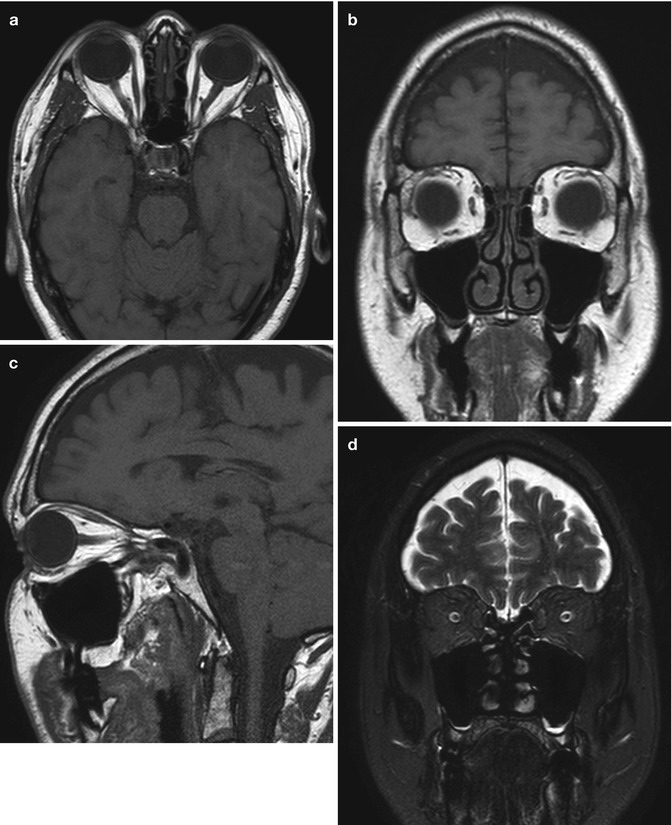
Fig. 1.5
Basic orbit MRI sequences without contrast include axial (a), coronal (b), and oblique sagittal (c) T1-weighted and coronal fat-suppressed T2-weighted (d) images
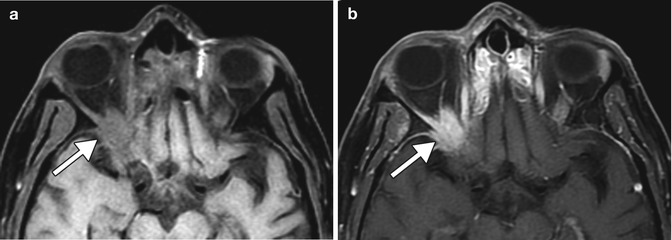
Fig. 1.6
Contrast-enhanced MRI for recurrent tumor assessment. Axial fat-suppressed T1-weighted image (a) and post-contrast axial fat-suppressed T1-weighted image (b) show an ill-defined, avidly enhancing tumor (squamous cell carcinoma) in the orbital apex (arrows)
1.5 Ultrasound
Ultrasound is an important imaging modality that is used for the initial clinical work-up and follow-up of many ophthalmic diseases, as it is relatively inexpensive to perform and readily available to ophthalmologists. The examination entails applying the surface of the probe or transducer to closed eyelids in contact with a gel or immersion fluid. Since a mild amount of pressure is applied to the globe, ultrasound examinations are contraindicated in patients with a possible open globe. Nevertheless, ultrasound is a versatile modality that is particularly well suited toward the evaluation of the intraocular contents, which is a relative limitation of CT and MRI. B-mode ultrasound is commonly performed, which provides a cross-sectional grayscale representation of the globe and surrounding structures in different planes, such as transverse and longitudinal (Fig. 1.7). The aqueous and vitreous are normally nearly anechoic on ultrasound and readily allow passage of the sound waves, while interfaces, such as the eye wall, tend to appear hyperechoic and may even cause shading in some cases. The in-depth spatial resolution that can be achieved with sonography is primarily dependent upon the frequency emitted by the transducer. The use of frequencies of 50 MHz and higher is known as ultrasound biomicroscopy, which can depict anatomic structures in exquisite detail, particularly in the anterior chamber and angle (Fig. 1.8). Another ultrasound modality is color Doppler imaging, which consists of simultaneous grayscale imaging of structure and color-coded imaging of blood velocity. This technique enables depiction of moderately small-caliber blood vessels, such as the central retinal artery, from which measures of blood velocity and vascular resistance can be obtained. The use of intravascular contrast agents, such as microbubbles, in orbital imaging is mainly investigational at the time of this writing, although these may have promising and intriguing applications in the realm of molecular diagnosis, therapy, and theranostics.
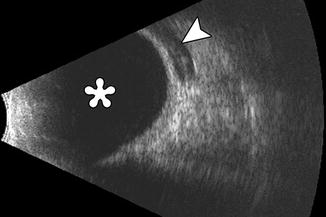
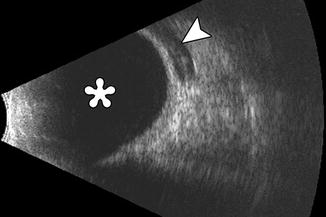
Fig. 1.7
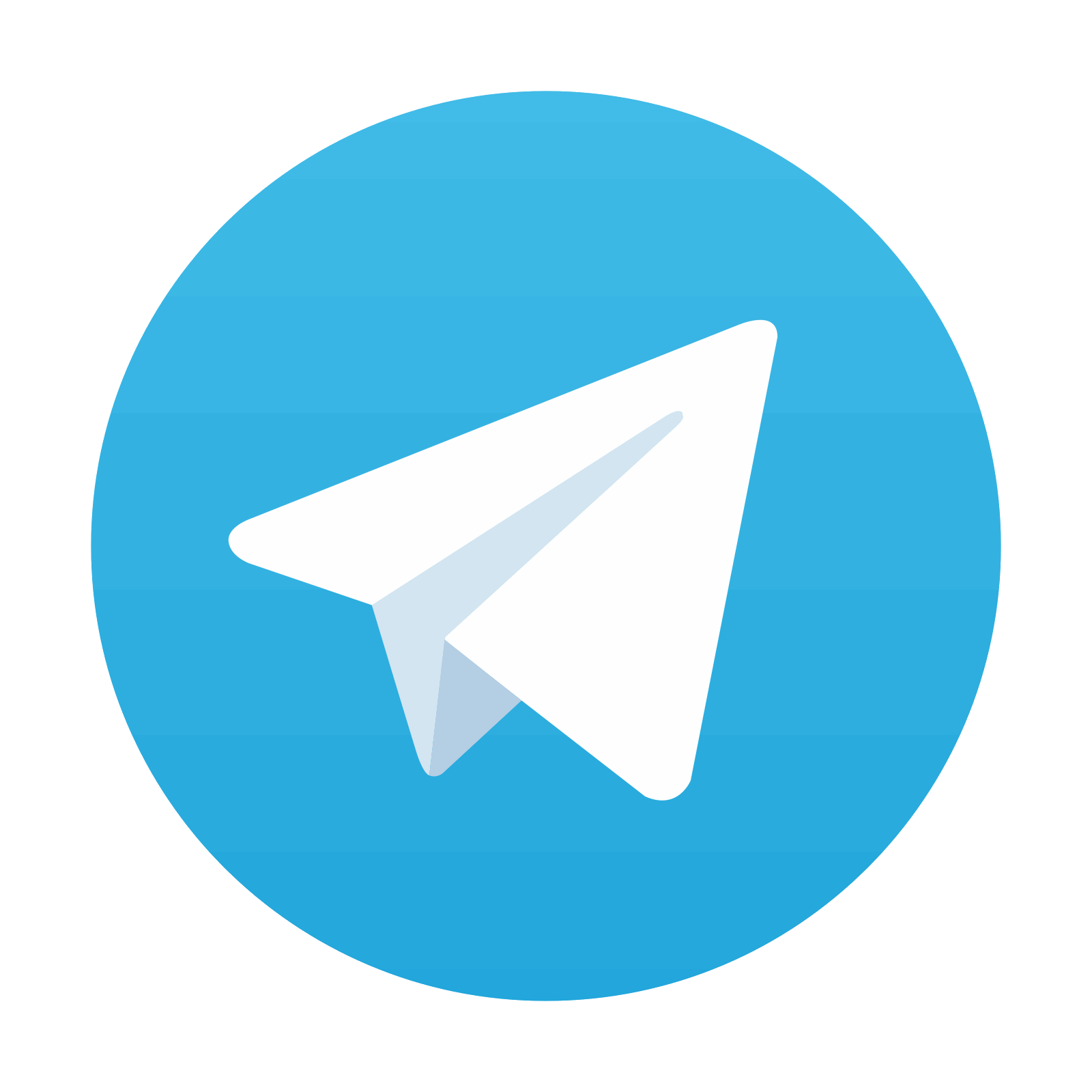
B-mode ultrasound image of a patient with glaucoma drainage device (arrow). The vitreous body is anechoic
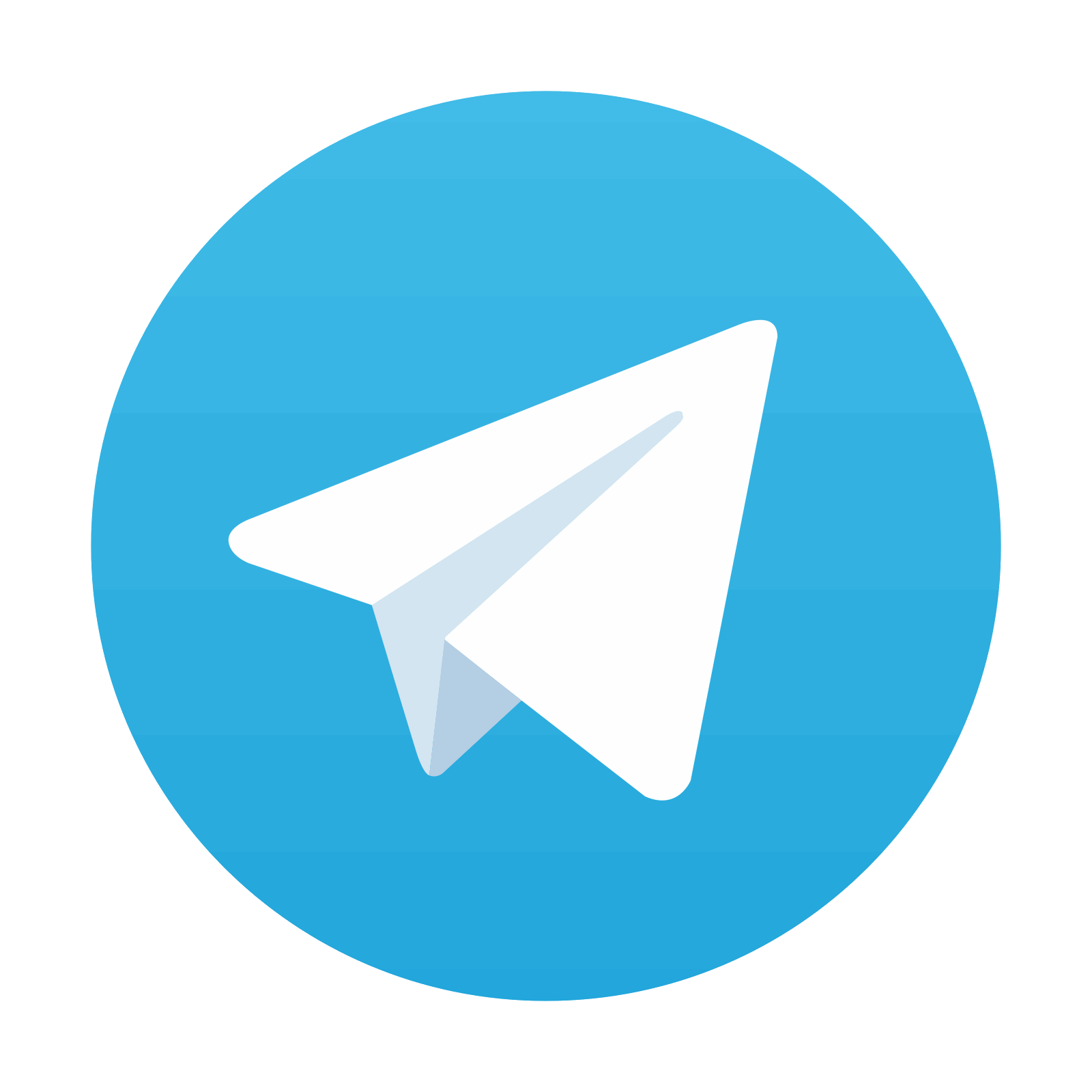
Stay updated, free articles. Join our Telegram channel

Full access? Get Clinical Tree
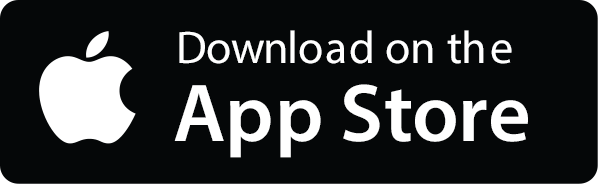
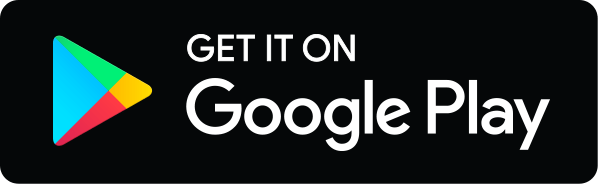
