Introduction to Imaging Methodology and Airway Anatomy
With the introduction and widespread availability of multidetector computed tomography (CT) scanners capable of providing continuous and/or overlapping high-resolution, near-isotropic thin sections throughout the lungs in a single breath hold, virtually all the lobar, segmental, and subsegmental bronchi can now be routinely identified in nearly all cases. In combination with advanced image processing techniques, including multiplanar reconstructions (MPRs), 3D surface renderings, and virtual endoscopic views, CT is now well established as the noninvasive imaging procedure of choice to evaluate virtually the entire gamut of diseases affecting the airways (1). Clinically, this includes evaluating patients presenting with chronic cough, dyspnea, chest pain, and hemoptysis (2, 3, 4). To date, CT has proved especially valuable in identification of occult airway pathology, including in patients with suspected airway malignancy with normal or nonlocalizing chest radiographs (5); evaluation of airway patency in patients in whom either partial or complete airway obstruction from a variety of causes is suspected (6,7); and identification of inflammatory airway disease, especially the diagnosis of bronchiectasis (8, 9, 10). In addition to these indications, CT has also become an invaluable tool for diagnosing diseases involving the small airways (11, 12, 13, 14, 15, 16, 17). As discussed in greater detail in Chapter 2, CT has also become an indispensable tool for assessing potential interventional techniques including, among others, the selection of appropriate patients for transbronchial needle aspirations (18) as well as patients with airway obstruction requiring stents (19).
IMAGING TECHNIQUES
The introduction of multidetector CT scanners capable of both prospectively and retrospectively providing high-resolution near-isotropic images throughout the entire thorax in a single breath hold has vastly simplified the task of imaging the airways. This is because it is now possible to use a single “generic” acquisition protocol for essentially all cases, including those specifically referred for evaluation of potential airway pathology. Although technical parameters vary depending on the type of scanner used, a few basic principles apply to nearly all current multidetector scanners. These include use of the thinnest possible collimators (typically 0.75 to 1.5 mm) and the shortest available scan times (i.e., subsecond image acquisition to minimize respiratory motion), with z-axis coverage to include the entire thorax in a single breath-hold period (typically a pitch of between 1 and 2). These parameters allow routine prospective reconstruction of both contiguous 5-mm sections (imaged with appropriate mediastinal and lung reconstruction algorithms, respectively) and high-resolution 1- to 1.5-mm sections reconstructed every 10 mm using an appropriate edge-enhancing algorithm. In effect, current-generation multidetector CT scanners maximize imaging flexibility by turning every study into both a routine and a high-resolution examination. Depending on the individual case, additional retrospective thin sections can then be reconstructed through appropriate regions of interest to clarify potential endobronchial abnormalities. In those cases for which MPRs or virtual bronchoscopic images are desired,
contiguous and/or overlapping high-resolution 0.75- to 1.5-mm images also can be prospectively and/or retrospectively reconstructed. These techniques represent a considerable improvement over conventional CT in the era before multidetector CT in which the sensitivity and specificity of CT for detecting obstructive lesions of the respiratory tract, for example, were reported to be as low as 60%, leading some investigators to consider CT inadequate, for example, as a method for initial evaluation of the airways in patients with suspected lung cancer or hemoptysis, (20,21).
contiguous and/or overlapping high-resolution 0.75- to 1.5-mm images also can be prospectively and/or retrospectively reconstructed. These techniques represent a considerable improvement over conventional CT in the era before multidetector CT in which the sensitivity and specificity of CT for detecting obstructive lesions of the respiratory tract, for example, were reported to be as low as 60%, leading some investigators to consider CT inadequate, for example, as a method for initial evaluation of the airways in patients with suspected lung cancer or hemoptysis, (20,21).
In addition to the imaging parameters mentioned previously, other considerations include dose as well as the use of contrast enhancement. In patients for whom the primary indication is visualization of the central airways, in particular, low-dose (40 to 80 mA) technique should be used to minimize radiation exposure (22). Boiselle et al. (23) conducted a combined phantom and clinical study of the effect of radiation dose on multiplanar and 3D image quality using both single-detector and multidetector CT scanners. They found that, although image quality was consistently superior with multidetector CT, no distinguishable differences in image quality could be identified comparing images obtained at 40, 120, and 240 mA, respectively. Similar considerations apply when the primary indication for performing CT is to visualize the peripheral airways and lungs. Low-dose techniques should also be standard for evaluating airway pathology in pediatric patients as well as younger individuals, especially women of child-bearing age (24).
Although there are no definitive guidelines regarding intravenous contrast administration, use of iodinated contrast media allows improved visualization of peribronchial and parenchymal abnormalities. It should be routinely used, in particular in patients in whom there is suspicion of mediastinal or hilar disease or radiographic evidence of parenchymal consolidation or collapse
(Fig. 1-1). Rarely, precontrast- and postcontrast-enhanced images may allow a more precise characterization of abnormalities such as hypervascular tumors (Fig. 1-2) or hypovascular lesions such as bronchogenic or esophageal duplication cysts.
(Fig. 1-1). Rarely, precontrast- and postcontrast-enhanced images may allow a more precise characterization of abnormalities such as hypervascular tumors (Fig. 1-2) or hypovascular lesions such as bronchogenic or esophageal duplication cysts.
Another technique of considerable value is the addition of select expiratory high-resolution images. As discussed and illustrated throughout this text, high-resolution expiratory images are essential to the diagnosis of a wide range of abnormalities, including, among others, tracheobronchomalacia and obstructive small airway diseases (Fig. 1-3) (25). Although some advocate the routine acquisition of select expiratory images as part of a standard CT examination of the thorax, in our experience this has not proved worthwhile in the vast majority of cases. It should be emphasized that in those cases for which paired inspiratory and forced-expiratory images are required, they should be obtained with a low-dose (40 to 80 mA) technique. As documented by Zhang et al. (22), in a study of tracheal lumen measurements in 10 patients with tracheomalacia, no discernible differences in diagnosis could be identified comparing standard and low-dose techniques.
With the introduction of advanced multidetector CT scanners, interest has also been directed at the potential use of both respiratory and electrocardiogram (ECG) gating to enhance visualization of the tracheobronchial tree. In a recent retrospective study of 25 patients in whom the effect of ECG gating on virtual bronchoscopic images was evaluated, surprisingly image quality proved superior on nongated versus gated images, regardless of the time interval used for reconstructing ECG-gated images (26). Coupled with the substantially higher radiation dose needed to perform ECG gating, this technique should not be used preferentially to study the airways.
Advanced Reconstruction Techniques
Although routine axial images remain the standard for identifying airway disease, as discussed in Chapter 2, this method has important limitations, especially in comparison with flexible bronchoscopy. First, CT is limited in its ability to accurately differentiate among mucosal, submucosal, and extrinsic causes of airway narrowing (see Figs. 2-2 and 2-3). Axial CT images also are of notoriously limited value in estimating the length of airway stenoses.
With the recent introduction of multidetector CT scanners capable of generating hundreds of contiguous and/or overlapping high-resolution thin sections throughout the thorax, there has been growing impetus to rely on alternative methods of reconstruction to evaluate the airways and lungs (Fig. 1-4) (27). These include MPRs, maximum intensity projections (MIPs) and minimum intensity projections (MinIPs), external rendering with either 3D shaded-surface displays (SSDs) or volumetric rendering, and internal rendering, or so-called virtual bronchoscopy (VB). Although currently MPRs alone have proved of routine clinical value (23,28), there is still considerable interest in the potential use of the other techniques, especially VB.
Multiplanar Reconstructions
MPRs are typically 1-voxel-thick two-dimensional “tomographic” sections interpolated along an arbitrary plane or, less commonly, a curved surface. Compared with other imaging techniques, MPRs have the advantages of taking little time to reconstruct and requiring no skill. With modern scanners, coronal and sagittal MPRs may be routinely prospectively reconstructed, provided initial volumetric data acquisition is available. The main advantage of MPRs is the ability to allow individual airways to be displayed to best advantage along their nearest long axes. Although variations have been proposed, including doubleobliquity MPRs perpendicular to previously reconstructed 3D images (29,30) and curved MPRs employing a userdefined trace to render complex 3D structures in a single flat tomographic plane, these techniques are time-consuming and have proved of only limited clinical value. Unfortunately, even with volumetric acquisition, MPRs are susceptible to stair-step artifacts, which limit interpretation. Typically, these artifacts manifest as irregularities along the surfaces of airways that course obliquely. They are directly related to pitch and are independent of collimation or reconstruction algorithm (31).
Despite these potential limitations, MPRs have consistently been shown to be of considerable clinical value, especially for assessing focal airways stenoses such as those that occur following prolonged intubation or lung transplantation (27,32, 33, 34, 35). Following lung transplantation, MPRs have been shown to be more accurate than axial images in differentiating normal postoperative changes, such as diverticula resulting from redundant mucosal folds and endoluminal flaps and linear air collections resulting from bronchial invagination, from the more ominous findings of dehiscence and bronchial fistulization (36).
Maximum and Minimum Projection Images
MIP and MinIP images are 2D representations of 3D data in which either the highest or lowest individual voxel values are projected through a given subvolume along parallel (orthogonal) rays. Although this allows specific density data to be extracted and evaluated independently, this technique sacrifices 3D depth information. Use of the lowest attenuation values within the slab (MinIP imaging) yields images in which the bronchial lumen is accentuated at the expense of the bronchial wall (Figs. 1-4, 3-3, and 3-30A). In distinction, use of the highest available attenuation values within the slab (MIP imaging) accentuates the bronchial wall at the expense of the bronchial lumen (Fig. 1-4). In both cases, the result is to distort the normal cross-sectional appearance of the airways by accentuating only a portion of airway anatomy (37). First introduced by Napel et al. (38) as a technique for improving visualization of blood vessels and airways in the parenchyma of the lung, sliding MIP images have found limited use especially in identifying foci of small airway inflammation (Fig. 1-5). In distinction, although of limited value for evaluating the central airways, MinIP images have proved of use for evaluating the lung parenchyma, both in patients with emphysema and in those with small airway disease (39, 40, 41, 42).
External Rendering
External rendering enables visualization of structures from an external vantage point, most often using a simulated light source (see, for example, Figs. 3-5C, 3-10B, and 3-30B). This can be accomplished using either surface thresholding or volume-rendering techniques. In the former, a single binary threshold is applied, rendering only a few voxels visible along the surface of selected structures. These are given the appearance of depth by illuminating the surface with an imaginary source of light. In distinction, volume rendering uses linear or continuous scaling techniques in which every voxel within the original dataset is assigned a proportional value based on the full range of tissue densities represented (43). Although each technique has both advantages and disadvantages, to date, neither has gained widespread routine clinical utility (44). Despite the striking visual appearance of these images, external rendering techniques usually require time-consuming editing to create and rarely allow visualization of airways beyond the mainstem and proximal lobar bronchi. With the shaded-surface technique, in particular, the use of a single threshold also makes these images susceptible to numerous artifacts. When inappropriately selected the result is surface discontinuities or “holes,” jagged edges, and “floating pixels.” Focal areas of high density including bronchial stents and bronchial cartilage calcifications also may be completely obscured (31).
Analogous to MPRs, 3D surface-rendering techniques have most often been used to identify central bronchial stenoses. Three-dimensional images also have been anecdotally
reported of value in a wide range of disparate conditions, including tracheal compression resulting from kyphosis (45), congenital airway abnormalities such as cardiac bronchus (46) or those associated with vascular malformations or rings (24), following stent placement (19), even identification of bronchopleural fistulas (47), among others (1,35,48).
reported of value in a wide range of disparate conditions, including tracheal compression resulting from kyphosis (45), congenital airway abnormalities such as cardiac bronchus (46) or those associated with vascular malformations or rings (24), following stent placement (19), even identification of bronchopleural fistulas (47), among others (1,35,48).
Virtual Bronchoscopy
Internal rendering, or VB, allows visualization of the airways from a point source at a finite distance, simulating human perspective as seen through a bronchoscope. Identical to external imaging of the airways, virtual bronchoscopic images can be obtained using either 3D SSDs or volumetric rendering (30,49, 50, 51, 52). Although a detailed description of these techniques is outside the scope of this chapter, a few pertinent aspects of these techniques need to be addressed. With 3D surface rendering, a specific attenuation coefficient is chosen as a threshold to define the air-lumen interface. This renders all voxels as representing either air or soft tissue, without an identifiable transition zone. Unfortunately, this technique has the disadvantage of greater susceptibility to noise and partial volume averaging, resulting in surface discontinuities, jagged edges, and floating pixels. Furthermore, no optimal threshold has been described; instead, thresholds vary, in particular, as a function of the density of the bronchial wall. Using a bronchial phantom, Maniatis et al. (53) have proposed a threshold value of −520 Hounsfield units (HU) as appropriate for visualizing the main central airways, whereas −720 HU has been suggested as requisite for accurate visualization of segmental and subsegmental bronchi.
![]() Figure 1-6 Virtual bronchoscopy. A and B: Virtual bronchoscopic views of the carina in the same patient as Fig. 1-5 reconstructed using shaded-surface (A) and volumetric rendering (B) techniques. Note that for purposes of routine endoscopic viewing, there is little difference between these techniques. |
In distinction, volume rendering makes use of the entire volume of data in which each voxel is classified on the basis of its attenuation value. This allows not only visualization of the endoluminal surface of the airways but real-time visualization of structures through the airway wall, as well. Although this is an advantage over SSTs, it should be noted that this difference has not proved clinically significant. When viewed endoscopically, little distinguishes these techniques (Fig. 1-6). In a recent study comparing the use of ECG gating on evaluation of the bronchial tree, Schertler et al. (26) found no difference in the ability of VB images to visualize all bronchial segments regardless of whether they were reconstructed using an SST or volumetric rendering. It is also noteworthy that nearly all currently available workstations allow simultaneous evaluation of peribronchial tissues through simultaneous annotated displays of corresponding axial, coronal, and sagittal images oriented to conform to any given plane identified from the endoluminal display, further minimizing differences between these techniques. Finally, although it has been suggested that volumetric rendering allows depiction of the bronchial mucosa by including a separate transition zone between air within the lumen and the bronchial wall, it has yet to be shown that this transition zone actually correlates with the appearance of the mucosa at bronchoscopy (54). In this regard, in
a study of 44 consecutive patients with thoracic malignancies, although HRCT including VB correctly identified 90% of endoluminal abnormalities (including 100% of obstructive lesions), only 16% of mucosal lesions identified bronchoscopically were correctly identified prospectively (55). It is also worth emphasizing that in this study, although all normal patients were correctly identified by CT, CT demonstrated two false-positive obstructive lesions as well (55).
a study of 44 consecutive patients with thoracic malignancies, although HRCT including VB correctly identified 90% of endoluminal abnormalities (including 100% of obstructive lesions), only 16% of mucosal lesions identified bronchoscopically were correctly identified prospectively (55). It is also worth emphasizing that in this study, although all normal patients were correctly identified by CT, CT demonstrated two false-positive obstructive lesions as well (55).
Other generally available VB tools include orthogonal axes to indicate anterior, posterior, or superior direction and “rearview” imaging, which allows simultaneous depiction of a virtual endoscopic image pointing backward from any given location. This latter technique allows inspection of endobronchial lesions from a vantage point not accessible via routine bronchoscopy (52). It is also possible to measure distances between any fixed points within the airways using “trail markers,” which are analogous to the distance markers present on fiberoptic bronchoscopes (52). More recently, attempts have been reported of correlating virtual bronchoscopic images with flexible bronchoscopy in real time, using either sensors attached to the tip of the bronchoscope or external fiduciary markers (56). It has also been shown that augmented endoscopic images combining both real and virtual bronchoscopic information can be created in real time, as the bronchoscopy is being performed, even in the absence of sensors or fiduciary markers (57).
It should be emphasized that the principal parameter in determining the quality of virtual bronchoscopic images is use of thin collimation. In an early study using a single-detector CT scanner with 3-mm sections and a 1-mm reconstruction interval, for example, Summers et al. (30) were only able to identify 82% of lobar and 76% of segmental airways. Similar data using 3-mm collimation have been reported by Lacasse et al. (58), who found that the sensitivity of VB for detecting lobar and segmental bronchial lesions was only 76% and 48%, respectively. Multidetector CT scanners substantially improve the capacity to image the peripheral airways (59). With retrospective target reconstruction using fields-of-view between 16 and 18 cm, spatial resolution now approximates 0.5 mm, allowing routine identification of fifth- to eighth-generation bronchi that were not previously identifiable. The degree to which near-isotropic imaging enhances VB has recently been documented (60). In a comparison of 1.5-mm sections reconstructed q 0.75 mm with 0.75-mm sections reconstructed q 0.4 mm using a 16-row multidetector CT, the use of thinner sections improved visualization of the mean generation of bronchial bifurcations from 4.8 to 6.5 mm (p <.0003) (Fig. 2-18); similarly, the diameters of recognizable bronchi improved from a mean of 7.5 to 4.6 mm (p <.0001) (60).
To date, indications for VB to evaluate the central airways (extending from the trachea to the proximal lobar bronchi) have been extensively reported. These include assessing tracheal and proximal bronchial stenoses (61, 62, 63, 64), including in association with esophageal atresia in neonates and infants (65), as well as in identifying central airway tumors (48,54,58,66, 67, 68). VB has been used to evaluate benign bronchial tumors (69), Wegener’s granulomatosis (70), suspected foreign body aspiration in children (71), and esophageal carcinoma infiltrating the tracheobronchial tree (72), among others. Unfortunately, none of these indications has received widespread clinical acceptance, largely because nearly identical information regarding the central airways has been available from routine axial and multiplanar reconstructions (54,73,74). Although at least one report has shown VB to be more accurate than MPRs or MIPs for accurately assessing the length of bronchial stenoses, frequent false-positive endobronchial abnormalities have been reported in most series (55).
However, VB does have important potential uses. As discussed in detail in Chapter 2, VB may be of use in determining optimal sites for performing transbronchial needle aspirations and biopsies (see Fig. 2-16) (75). VB has also been successfully used as a means for teaching optimal methods for performing these techniques to inexperienced bronchoscopists (76,77). This potential use of VB is especially promising because, despite excellent results in the reported literature, transbronchial needle biopsy remains generally underutilized (78,79). Another potential area in which VB may come to fill an essential role is in providing a road map for ultrathin bronchoscopic evaluation of peripheral lung lesions (Fig. 1-7). Currently only limited attempts have been made to expand VB to include the peripheral airways (27,80,81).
Advanced Imaging Techniques: Airway Segmentation and CT Bronchography
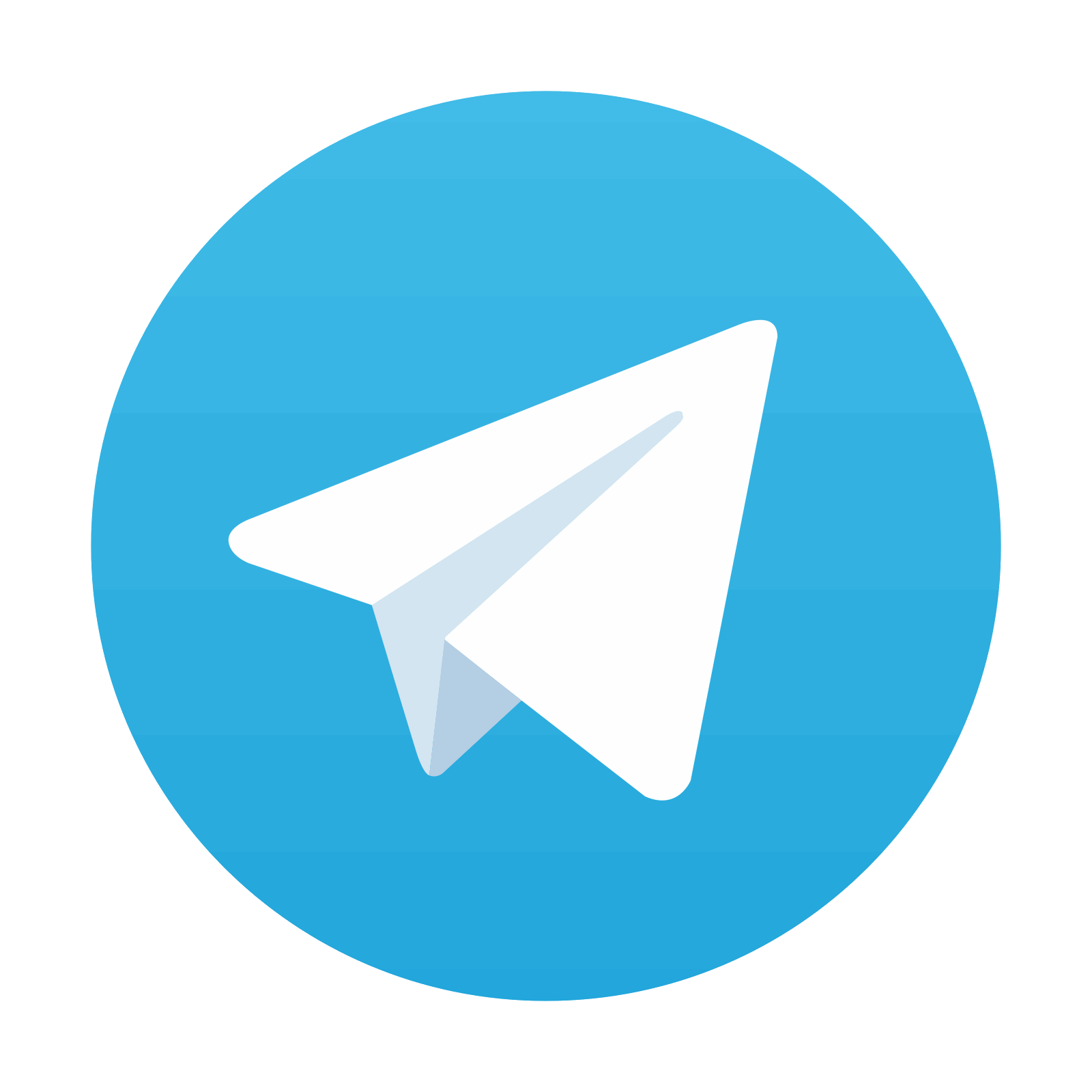
Stay updated, free articles. Join our Telegram channel

Full access? Get Clinical Tree
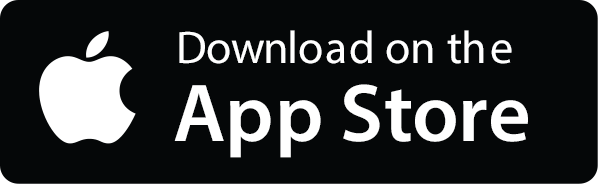
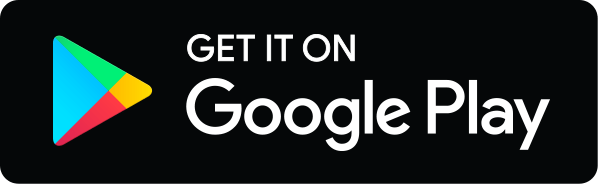