Fig. 1.1
Atrial pressure waveforms. The dotted line represents the “c-v”-wave seen in the right atrium from severe tricuspid regurgitation
Table 1.1
Atrial waveforms and their etiology
a | Atrial contraction |
x | Right atrial rela(x)ation |
c | Early ventricular contraction resulting in upward tricuspid valve movement |
v | Atrial filling |
y | Right atrial empt(y)ing |
Table 1.2
Right atrial pressure in specific scenarios
Atrial septal defect (large) | Equalization of a and v amplitudes |
Severe tricuspid regurgitation | Large “c-v”-waves |
Atrial fibrillation or flutter | Absence of a-waves |
AV dissociation or dyssynchrony (e.g., complete AV block) | Cannon a-waves |
Pericardial tamponade | Loss of y (and possibly x) descent; equalization of right and left atrial (wedge) pressure |
Constriction | Rapid y descent – more pronounced with inspiration |
Restriction | Rapid x and y descent |
Left Atrium
Mean pressures (5–10 mmHg) tend to be higher than in the right atrium with v-waves being more prominent than a-waves. Left atrial pressures can reflect left ventricular preload as well as compliance. They can be estimated invasively by obtaining the pulmonary artery wedge pressure (PAWP). In the setting of mitral stenosis, there will be a pressure gradient between the left atrium and left ventricle. Throughout diastole, the PAWP will be higher than the LV pressure and will remain higher at end-diastole. The area under the curve of the pressure gradient can be utilized to calculate mitral valve area (discussed later).
Right and Left Ventricle
The ventricular waveform includes a brisk early systolic rise and a brisk fall in early diastole (typical values 25/5 mmHg in the right, 120/10 mmHg in the left). There can be an end-diastolic peak corresponding to atrial contraction, sometimes referred to as the “post-a-wave” left ventricular end-diastolic pressure. Elevated LVEDP (greater than 12 mmHg) can be associated with abnormal left ventricular filling or compliance. A thorough evaluation should mention both pre- and post-a-wave values for LVEDP.
Aortic and Pulmonic Artery Pressures
In the aorta and pulmonary artery, there is a pronounced systolic upstroke, followed by a downstroke with a brief positive deflection due to aortic valve closure (dicrotic notch) (Fig. 1.2). The mean aortic pressure is somewhat lower distally than proximally, although there may be a slight rise in the systolic pressure. In the setting of significant aortic regurgitation, there can be end-diastolic pressure equalization between the LV and aorta (Fig. 1.3).
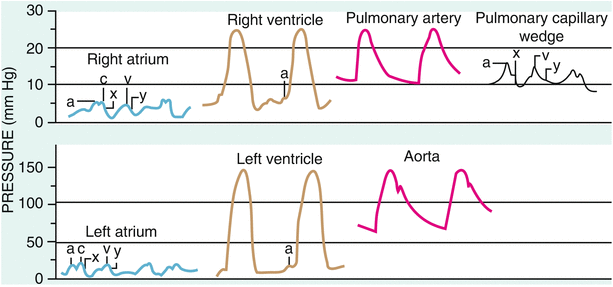
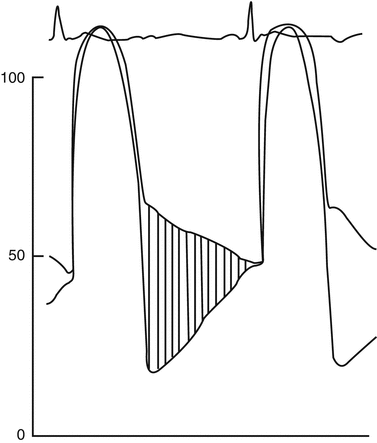
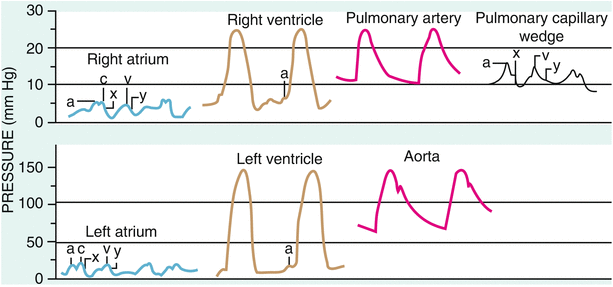
Fig. 1.2
Classic atrial, ventricular, and pulmonary artery pressure tracings
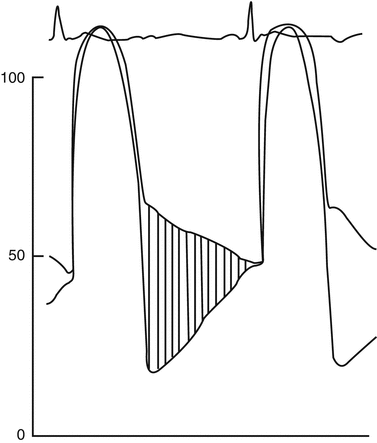
Fig. 1.3
Left ventricular and aortic pressure tracings in severe aortic regurgitation. Note the wide aortic pulse pressure and the end-diastolic equalization of pressures
Mean pulmonary artery pressures less than 21 mmHg are considered normal. Mean values above 25 mmHg suggest pulmonary hypertension.
Pulmonary arterial hypertension is a more specific diagnosis and carries four criteria:
1.
Resting mean pulmonary artery pressure >25 mmHg
2.
Pulmonary vascular resistance >3 Wood units
3.
Pulmonary artery wedge pressure <15 mmHg
4.
Absence of other causes of pulmonary hypertension
Echocardiography has largely replaced right heart catheterization as the modality of choice for evaluating pulmonary artery pressures. In certain circumstances, both modalities are used. For instance, heart transplant recipient candidates are typically referred for both procedures. While some studies show a good correlation between pulmonary pressures as estimated by both modalities, results from the clinical arena show both poor correlation and modest agreement, due to a number of factors including patient hydration and sedation status during invasive studies as well as echocardiographic technique.
Pulmonary Artery Wedge Pressure (PAWP)
The PAWP estimates left atrial pressure (normal range 8–12 mmHg). The careful advancement of a pulmonary artery catheter, with its balloon tip inflated, will normally “wedge” the balloon such that arterial flow is interrupted beyond that point and the pressure tracing changes. In case of uncertainty, an arterial sample obtained from this position should yield an oxygen saturation very similar to aortic values.
Wedge pressure waveforms follow those of the left atrium, with an a-wave corresponding to atrial contraction followed by an x descent due to atrial rela(x)ation, a v-wave corresponding to atrial filling, followed by a y descent due to atrial empt(y)ing. The x descent corresponds with the QRS complex on ECG. The v-wave has a larger amplitude than the a-wave, reflecting left atrial pressure characteristics.
PAWP, as with the PA pressure, should typically be measured at the end of expiration, just before the inspiratory decline. With mechanically ventilated patients, PAWP is measured just before the inspiratory rise in pressure. While the PAWP reflects left atrial pressure, it can be higher in the presence of mitral stenosis or pulmonary venous hypertension, for example (Fig. 1.4). If suspected, a simultaneous LVEDP should be measured.
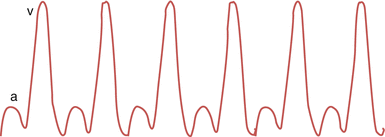
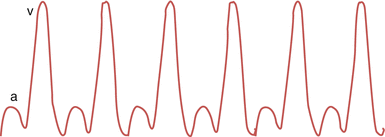
Fig. 1.4
Pulmonary artery wedge pressure waveform with “giant v-waves” that can be seen in severe mitral regurgitation, ventricular septal defect, as well as mitral stenosis and with a stiffened left ventricle
Cardiac Output
Indicator dilution and Fick equation are the principal invasive means in present use to estimate cardiac output. Indicator dilution is based on the principle that if a known quantity of a tracer is introduced into the circulation, its concentration over time can be used to estimate circulating volume. One example of this principle, thermodilution, measures iced or room temperature saline, injected from a more proximal port within the RA and measured by a thermistor in the PA. The area under curve provides an estimate of cardiac output. Usually 3–5 separate measurements are made and outlier values eliminated. This technique can be less reliable in the setting of severe tricuspid and pulmonic regurgitation, as well as intracardiac shunting.
German physiologist and inventor of the contact lens, Adolf Fick (1829–1901) determined that regional uptake of a substance by an organ, in this case oxygen by the lungs, is the product of blood flow to the lungs and the arteriovenous oxygen concentration difference across the lungs. To determine intravascular oxygen content, oxygen saturation samples obtained from the pulmonary artery and aorta are essential for this calculation. In clinical practice the femoral artery sheath is often used to draw an aortic blood sample surrogate. The utilization of pulmonary and aortic blood to estimate pulmonary oxygen uptake assumes that there is no R-to-L shunting at the intracardiac level. The Fick equation also requires estimation of lung oxygen uptake. While some labs measure this directly, for example, using a Douglas bag or polarography, another approach is to estimate it. The normal oxygen consumption rate is approximately 125 ml/m2 of body surface area, or 3 ml O2/min/kg, but this value differs with age and sex:
![$$ \begin{gathered} \mathrm{Cardiac}\;\mathrm{Output} = {{\mathrm{O}}_2}\;\mathrm{consumption}\;\left( {\mathrm{m}\mathrm{l}/ \min } \right)/\mathrm{arteriovenous}\;{{\mathrm{O}}_2}\;\mathrm{difference} \times \left[ {\mathrm{Hb}} \right] \times 10 \hfill \\ \left( {\mathrm{Normal}\;\mathrm{cardiac}\;\mathrm{output}\;4-8\;\mathrm{L}/ \min,\;\mathrm{normal}\;\mathrm{cardiac}\;\mathrm{index}\;2.5-4\;\mathrm{L}/ \min /{{\mathrm{m}}^2}} \right) \hfill \\ \end{gathered} $$](/wp-content/uploads/2016/03/A307485_1_En_1_Chapter_Equ00011.gif)
![$$ \begin{gathered} \mathrm{Cardiac}\;\mathrm{Output} = {{\mathrm{O}}_2}\;\mathrm{consumption}\;\left( {\mathrm{m}\mathrm{l}/ \min } \right)/\mathrm{arteriovenous}\;{{\mathrm{O}}_2}\;\mathrm{difference} \times \left[ {\mathrm{Hb}} \right] \times 10 \hfill \\ \left( {\mathrm{Normal}\;\mathrm{cardiac}\;\mathrm{output}\;4-8\;\mathrm{L}/ \min,\;\mathrm{normal}\;\mathrm{cardiac}\;\mathrm{index}\;2.5-4\;\mathrm{L}/ \min /{{\mathrm{m}}^2}} \right) \hfill \\ \end{gathered} $$](/wp-content/uploads/2016/03/A307485_1_En_1_Chapter_Equ00011.gif)
[Hb] = serum hemoglobin concentration
Vascular Resistance
Derived from Ohm’s law (R = ΔP/Q), vascular resistance is calculated by dividing the pressure gradient across a vascular bed by the flow through it:


mPAP = mean pulmonary artery pressure, mPAWP = mean pulmonary artery wedge pressure, mSBP = mean systolic blood pressure, mRAP = mean right atrial pressure
Resistance is measured in hybrid reference units or Wood units (mmHg/L/min). By multiplying by 80, the value is converted into dynes.s.cm−5. Pulmonary vascular resistance (PVR) is normally less than 1.7 Wood units, about one tenth that of systemic vascular resistance (SVR). PVR is increased by various factors including blood viscosity, hypoxia, and acidosis. Pulmonary hypertension is said to be reversible with vasodilators if mean PAP falls by ≥10 mmHg to below 40 mmHg, or PVR must fall below 5 Wood units, without loss of cardiac output.
Calculations of Valvular Area
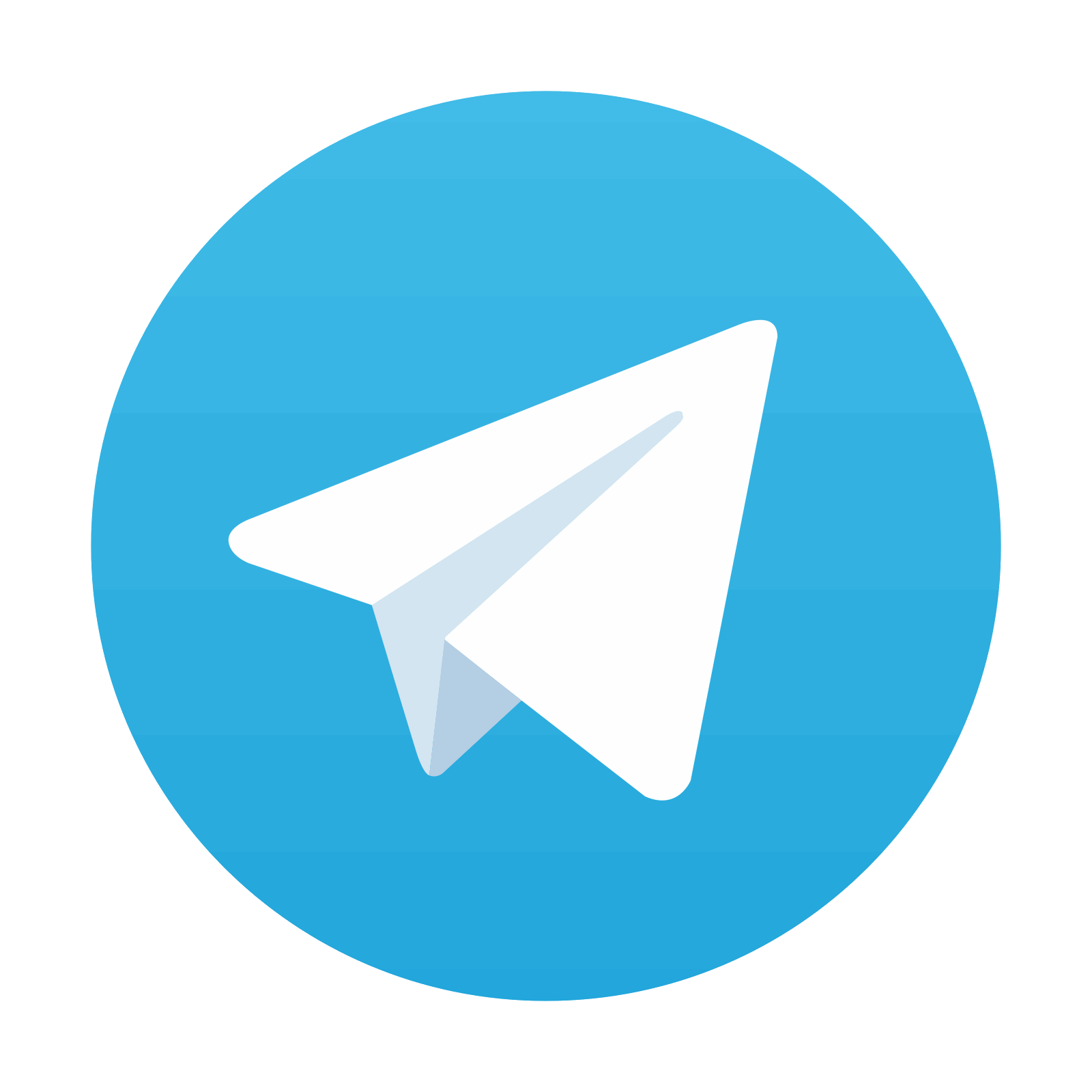
Stay updated, free articles. Join our Telegram channel

Full access? Get Clinical Tree
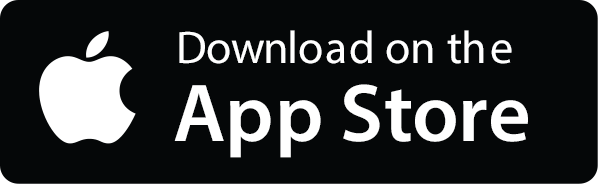
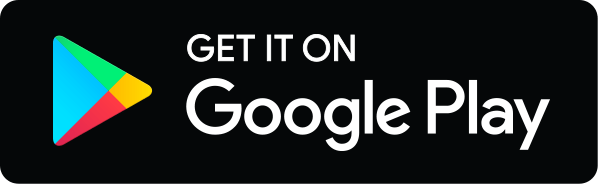