IVIM MRI: A Window to the Pathophysiology Underlying Cerebral Small Vessel Disease
To investigate the pathophysiology underlying cerebral small vessel disease (cSVD), novel advanced functional MRI techniques, such as intravoxel incoherent motion (IVIM), are very promising. Thus far, in vivo imaging of cSVD has been extensively performed with a focus on morphological abnormalities and blood perfusion. MRI has the advantage that it can reveal various types of small morphological abnormalities using various image contrasts together with information on blood perfusion and other physiological properties in one imaging session. IVIM, in particular, can provide physiological information in terms of quantitative measures on the microvascular and parenchymal integrity. This chapter reviews some (early) applications of IVIM in cSVD (and its related condition, stroke) and provides a methodological and clinical context.
4.1 Cerebral Small Vessel Disease
Cerebral small vessel disease (cSVD) is an umbrella term for pathologies with various etiologies that affect small arteries, arterioles, venules, and capillaries of the brain [1, 2]. This disease is strongly associated with ageing and cardiovascular risk factors. It contributes to up to 25% of all ischemic strokes and 45% of all dementias, causing cognitive, physical, and psychiatric disabilities [1]. Various pathologies underlie this disease, among which the most prevailing pathologies are cerebral amyloid angiopathy and arteriolosclerosis. A common form is arteriolosclerotic cSVD, which clinically manifests mostly as lacunar stroke or vascular cognitive impairment [1]. Employing conventional magnetic resonance imaging (MRI) methods to visualize structural abnormalities of the brain (i.e., T1w and T2w; e.g., fluid attenuated inversion recovery (FLAIR) imaging), morphological abnormalities of the brain can be visualized in cSVD [3] (Fig 4.1). In the clinic, the motivation to perform imaging in the acute situation is often to evaluate early infarcts and to differentiate between irreversibly affected brain tissue and reversibly impaired tissue, whereas imaging in the chronic phase (i.e., cSVD) is more aimed at helping elucidate the mechanisms underlying cognitive decline, gait disturbances, and mobility difficulties. Commonly observed abnormalities include white matter hyperintensities (WMHs), visible perivascular spaces (PVSs), small subcortical infarcts, lacunae, microbleeds, and brain atrophy. The visualization and assessment of these morphological abnormalities have significantly improved the characterization of the disease over the last years [3]. Until recently, the typical application of MRI in cSVD was to evaluate morphological abnormalities, which typically constitute the end state of damage to the microvessels. However, with advancement in functional MRI techniques, subtle abnormalities beyond the scope of macrostructure become visible, as functional MRI techniques potentially allow for earlier detection of abnormalities. Noninvasive imaging plays an important role in the management of cSVD, as it can provide in vivo pathophysiological information, without your having to rely on histopathology. The pathophysiological sequence leading to these abnormalities remains largely unknown. A better understanding of the pathophysiological mechanisms is crucial for developing effective treatment for patients with cSVD and risk assessment for cerebrovascular events.
Figure 4.1 Morphological abnormalities in cSVD visible in an MRI, such as white matter hyperintensities (A), lacunae (B), and enlarged perivascular spaces (C), which are indicated by the white arrows.
4.2 Impairment of Cerebral Blood Flow
An important mechanism in the pathophysiology of cSVD is the impairment of cerebral blood flow (CBF). The autoregulation of CBF is carried out by mural cells (i.e., pericytes and vascular smooth muscle cells) [4]. Through interactions with other elements of the neurovascular unit (NVU), they regionally regulate CBF by means of vessel contraction and dilation. For example, interneurons and endothelial cells have been shown to release nitric oxide, which relaxes the vascular smooth muscle cells and leads to vessel dilation [5]. In addition, astrocytes have also shown to be involved in the contraction and relaxation of pericytes [6]. Several changes in cSVD can contribute to the impairment of CBF. For example, cSVD is associated with the loss of smooth muscle cells and also the degeneration of the vessel wall composition [1]. Furthermore, pericytes have been shown to be affected [7]. The resulting malfunction in CBF autoregulation can cause persisting hypoperfusion [8]. This results in the depletion of nutrients and ultimately deterioration of brain tissue. However, hypoperfusion in itself is not a unique feature of cSVD, as many other neuronal and (systemic) cardiovascular disorders can contribute to impairment of CBF. Therefore, characterization of cSVD should focus on the properties of the vasculature and its interrelationship with parenchyma and not solely on blood flow.
4.3 Intravoxel Incoherent Motion Imaging Metrics as a Marker of Pathophysiology
IVIM imaging is a diffusion-weighted imaging (DWI) technique that has the potential to quantitatively describe the microstructural tissue environment and has received great attention in recent years [9]. DWI measures the diffusion properties of water molecules in living tissue. From these properties, structural information can be derived of the tissue microstructure in which the water molecules move. DWI has already shown its credible contribution by identifying tissue that might be salvaged after ischemic stroke [10]. IVIM imaging is characterized by the inclusion of low diffusion- sensitizing b values (i.e., <200 s/mm2), where the b value represents the amount of diffusion sensitization (i.e., a combination of applied field gradient strength and duration) during image acquisition [11, 12]. As the MRI measurement for the IVIM contrast is largely derived from attenuation measurements with relatively low b values, it is sensitive to water molecules that diffuse with no or relatively little hindrance. The IVIM signal was initially assumed to arise from water movements from two compartments: a microvascular compartment and a parenchymal one. In the microvascular compartment, water molecules flowing through a randomly orientated microvasculature give rise to the intravascular pseudodiffusivity D*, which is relatively large compared to parenchymal diffusivity D and has diffusion restrictions of the cell and matrix structures. Furthermore, the perfusion volume fraction f represents the volume fraction that gives rise to the intravascular diffusivity. Finally, the composite measure f·D* is sometimes used to represent blood flow. These quantitative measures can concurrently provide surrogate markers of both microvascular blood perfusion and parenchymal microstructure without the use of a (exogeneous) contrast agent. This technique seems ideal for studying the pathophysiology of cSVD, since both microvasculature and brain parenchyma are impaired in this disease. Therefore, IVIM imaging can be used to investigate affected microvascular blood perfusion and the extent to which the surrounding brain parenchyma is disintegrated.
4.4 Applications of IVIM in cSVD
In 17 patients with acute stroke, Federau et al. investigated the feasibility of IVIM perfusion imaging [13]. Patients presenting with symptoms of acute hemispheric stroke, which constitutes a rather critical form of cSVD as the blood flow to an area of the brain is cut off, were included. IVIM perfusion maps showed an area of decreased perfusion fraction f in the region of decreased diffusion coefficient D (indicative of cell swelling) and that both f and D were significantly lower compared with the contralateral side. As they observed a significantly reduced perfusion fraction (i.e., hypoperfusion) in the visible infarct, they concluded that IVIM perfusion imaging is feasible in acute stroke.
In a population of 15 patients with acute ischemic stroke, Hu et al. compared CBF metrics, obtained using arterial spin labeling, with IVIM metrics D and D* [14]. They found that D*, though not D, in the ischemic penumbras was significantly lower than those of the contralateral normal brain regions. Furthermore, a significant correlation was detected between D* and ASL-derived CBF. It was concluded that D* might be indicative of perfusion.
Yao et al. set out to investigate the feasibility of using IVIM to measure variations in diffusion and perfusion metrics in 38 stroke patients [15]. They reported positive correlations between f and CBF (as obtained through arterial spin labeling) as well as fD* and CBF. Quantitative analysis showed a significant decrease in D, D*, f, fD*, and CBF of the lesions compared with the contralateral side. It was concluded that IVIM analysis allowed separation of the perfusion contribution from parenchymal diffusion and thus provided an evaluation of both variations in perfusion and diffusion metrics during stroke, which might further elucidate the mechanisms of ischemic stroke.
Van Bussel et al. investigated hippocampal microvascular and microstructural changes in relationship with verbal memory performance in a population with diabetes mellitus type 2 (T2DM), a known risk factor for cSVD [16]. For the microvasculature, the blood perfusion volume (f) was larger in participants with T2DM, f and blood flow (fD*) increased with higher fasting blood glucose (FBG) levels, and microvascular pseudodiffusion (D*) and fD*, which are indicative of altered (vasodilated) microvasculature, were higher in participants with both relatively high FBG levels and low memory performance. For the parenchyma, the diffusion (D) was higher in T2DM, indicative of an injured microstructure, with reduced memory performance. It was concluded that the microvascular properties of the hippocampus were altered in participants with both T2DM and memory decrements and possibly hint at an underlying vascular mechanism, which complies with the notion that T2DM is a risk factor for cSVD, in addition to other cardiovascular morbidities.
Sun et al. used IVIM imaging in 32 subjects with visible WMHs to study the microvasculature and microstructure integrity and their association with cognitive performance [17]. They reported a decreased D* (indicative of a decreased blood flow) and an increased D (indicative of parenchymal degeneration) and f (showing increased microvascular volume) in both periventricular and deep WMH. Poorer cognitive performance was related to a decrease in f. With the latter finding, it was proposed that an elevated f could serve as a protective (i.e., compensatory) mechanism against cognitive decline, though this bold statement was not backed up with solid evidence.
Wong et al. performed a study in which IVIM imaging at 3T was used to measure both microvascular perfusion and parenchymal diffusivity in 73 patients with cSVD and 39 controls [18] (Fig 4.2). The authors used inversion recovery IVIM [19], which suppresses contamination of the CSF signal using inversion recovery preparation. Patients had a higher D (indicating microstructural tissue disintegration) and a larger f in normal appearing white matter (NAWM) compared to controls. Both f and D in NAWM and gray matter increased with disease severity, that is, the WMH load, suggesting that IVIM imaging might be able to provide a surrogate marker for the progression of cSVD. Zhang et al. studied the same group as Wong et al. but related IVIM measures D and fD* with cognitive performance [20]. They found in the patients that a lower fD* was associated with lower overall cognition, executive function, and processing speed. The relationship of higher D with lower cognitive scores disappeared after careful correction of covariates. It was concluded that perfusion in both cortical gray matter and NAWM may play a role in the pathophysiology of cognitive dysfunction in cSVD.
Figure 4.2 Example of a FLAIR image (A, D) and IVIM maps of the perfusion volume fraction f (B, E) and parenchymal diffusivity D (C, F) for a patient with cSVD and a control, respectively. The white arrows in the images in the upper row indicate tissue with high values of perfusion volume fraction f and parenchymal diffusivity D, which appears normal in the FLAIR image (A). Figure reprinted from Ref. [18] with permission from Elsevier, copyright 2017.
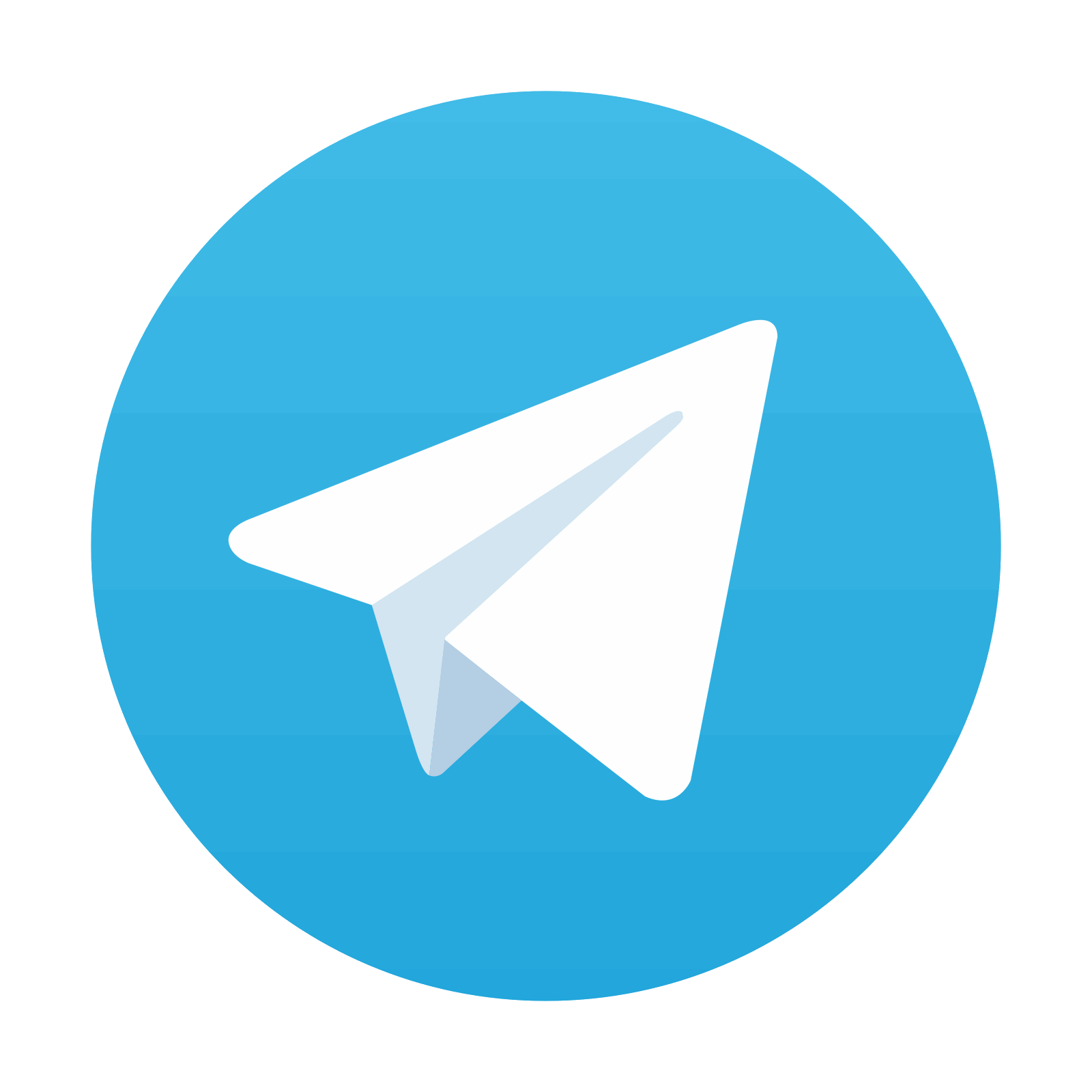
Stay updated, free articles. Join our Telegram channel

Full access? Get Clinical Tree
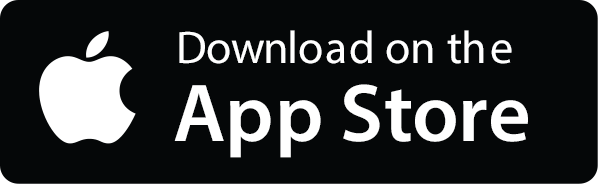
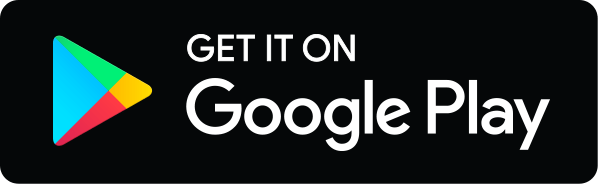