Liver, Bile Ducts, and Gallbladder
Andrew T. Trout
Daniel B. Wallihan
Alexander J. Towbin
Daniel J. Podberesky
INTRODUCTION
Imaging plays a crucial role in the assessment of disorders of the pediatric liver and biliary system. Radiologic studies not only can provide anatomic and morphologic information but can characterize tissues and assess hepatobiliary function. In this chapter, the most commonly employed imaging modalities, including state-of-the-art techniques are reviewed. Disorders of the liver are presented first, followed by disorders of the biliary tree and gallbladder. Normal anatomy of each of these systems is discussed because anatomic understanding is critical for accurate interpretation of imaging findings and effective communication with clinicians. Subsequently, the imaging appearances of common as well as unusual but important hepatobiliary abnormalities in the pediatric population are reviewed.
IMAGING TECHNIQUES
Radiography
Radiography is of little value in the primary assessment of the liver and biliary system. Findings that are visible by radiography (such as right upper quadrant calcification or gas) can suggest an underlying hepatic or biliary abnormality and may require further workup.
Ultrasound
The liver and biliary system are ideally suited for evaluation by ultrasound because of their position in the abdomen, the relative homogeneity of the hepatic parenchyma, and the high contrast between the hepatic parenchyma and biliary and vascular structures. As such, ultrasound is the initial modality of choice in the assessment of most hepatic and biliary abnormalities. Ultrasound provides primarily anatomic information but is ideally suited for the real-time assessment of the hepatic vasculature. Ultrasound elastography, including acoustic radiation force imaging (ARFI), is an emerging technique for noninvasive assessment of liver stiffness (Fig. 14.1).
Computed Tomography and Magnetic Resonance Imaging
Computed tomography (CT) and magnetic resonance imaging (MRI) provide high-resolution anatomic images of the liver and biliary tree. They are commonly employed following identification of an abnormality by ultrasound. In general, MRI is favored over CT in the pediatric population because of the lack of ionizing radiation and because it provides high soft tissue contrast. Additionally, advanced MR techniques, including diffusion-weighted imaging, chemical shift imaging, spectroscopy, elastography, and use of hepatobiliary-specific contrast agents, allow assessment of specific tissue characteristics. However, CT remains of particular value in the acute setting.
Nuclear Medicine
Hepatobiliary scintigraphy with iminodiacetic acid (HIDA) derivatives is the dominant nuclear medicine study employed for the assessment of the liver and biliary systems. HIDA allows dynamic assessment of hepatic function and evaluation of biliary system integrity and function. Today, sulfur colloid imaging is only rarely used to assess the liver in the pediatric population. 18F-fluorodeoxyglucose positron emission tomography (18F-FDG-PET) plays a minor but important role in evaluating primary and secondary liver tumors.
Interventional Diagnostic Imaging
Catheter angiography and percutaneous transhepatic cholangiography are typically employed in children when an abnormality has been identified with noninvasive imaging (e.g., ultrasound or MRI) and additional evaluation is required or intervention is likely to be performed. These techniques are particularly valuable in the management of transplanted livers.
NORMAL ANATOMY
Normal Hepatic Anatomy and Vascular Variants
Knowledge of hepatic segmental anatomy is important to the accurate interpretation of hepatic imaging in the child, as this often guides surgical and therapeutic planning. The liver consists of left and right hepatic lobes, separated by the middle
hepatic vein. The right and left lobes can be divided into a total of four sections or eight segments based on the Anglo-Saxon or Couinaud systems respectively (Fig. 14.2). These divisions are based on typical vascular and biliary boundaries that determine planes of hepatic resection. The most typical and thus the standard pattern of vasculature is as follows:
hepatic vein. The right and left lobes can be divided into a total of four sections or eight segments based on the Anglo-Saxon or Couinaud systems respectively (Fig. 14.2). These divisions are based on typical vascular and biliary boundaries that determine planes of hepatic resection. The most typical and thus the standard pattern of vasculature is as follows:
Portal vein: The main portal vein branches in the porta hepatis into the right and left portal veins, which supply their respective lobes. The right portal vein subsequently branches into anterior and posterior segmental branches.
Hepatic artery: The common hepatic artery arises as a branch of the celiac axis and branches into the gastroduodenal and proper hepatic arteries. In the porta hepatis, the proper hepatic artery branches into the right and left hepatic arteries, which supply their respective lobes.
Hepatic vein: The bulk of the liver is drained to the inferior vena cava (IVC) by the right, middle, and left hepatic veins. Often the middle and left hepatic veins form a common trunk prior to draining into the IVC. The caudate has separate venous drainage via multiple perforating caudate veins to the IVC.
SPECTRUM OF HEPATIC DISORDERS
Congenital and Developmental Anomalies
Preduodenal Portal Vein, Abernethy Malformation, and Other Congenital Portosystemic Shunts
The normal development of the portal vein involves a process of selective involution of a network of interconnections between paired embryonic vitelline veins (Fig. 14.3). This process results in the development of a portal vein that receives splanchnic inflow and courses posterior to the second portion of the duodenum to enter the liver hilum. When this process occurs abnormally, the position and drainage of the portal vein can become abnormal. The most extensively described of these portal venous developmental anomalies are the preduodenal portal vein and Abernethy malformation.
Preduodenal Portal Vein
A preduodenal portal vein receives normal inflow from the splenic vein (SV) and superior mesenteric vein (SMV). Instead of taking its normal course posterior to the second portion of the duodenum, the preduodenal portal vein passes anterior to the second portion of the duodenum. The radiologic and clinical significance of the preduodenal portal vein mostly relates not to the vein itself but to associated developmental anomalies. These include abnormal situs (including heterotaxy syndromes), intestinal malrotation, duodenal webs, biliary atresia, preduodenal common bile duct (CBD), annular pancreas, and congenital heart disease.6,7 An association has also been described with Down syndrome.8 Preduodenal portal veins can be diagnosed preor postnatally with the majority diagnosed in childhood.6 Partial duodenal obstruction is a possible clinical presentation. Importantly, the observed obstruction commonly relates to an associated anomaly (e.g., duodenal web) and not to the abnormally positioned portal vein.7
The abnormally positioned preduodenal portal vein can be identified by ultrasound, CT, or MRI based on its location relative to the second portion of the duodenum (Fig. 14.4). The aberrant portal vein may cause an impression on the anterior surface of the duodenum that is visible on a fluoroscopic upper GI examination, but sequelae of partial duodenal obstruction related to associated anomalies, such as duodenal webs, are more commonly observed manifesting as dilatation of the first portion of the duodenum (the so-called megacap).7
Abernethy Malformation and Other Congenital Portosystemic Shunts
The term “Abernethy malformation” is often used to describe any extrahepatic portosystemic shunt with an associated portal venous anomaly. Congenital extrahepatic portosystemic shunts (CEPS) is a more appropriate term for this broader group, which can be classified as detailed in Table 14.1.9 True Abernethy malformations relate to shunts between the splanchnic or portal venous system and the systemic venous system that arise during abnormal vitelline venous development. Shunts typically develop to the IVC, azygos vein, or right atrium.10,11 Although the effect is the same, extrahepatic portosystemic shunts via the renal and iliac veins are likely of a distinct origin.11
The effect of CEPS is to allow compounds that would normally be metabolized by the liver to enter the systemic system. Affected pediatric patients are variably symptomatic with clinical presentations ranging from no symptoms to mild hepatic dysfunction, hyperammonemia/hepatic encephalopathy, pulmonary hypertension, and/or hepatopulmonary syndrome.10 CEPS may be identified prenatally but can also go undiagnosed into adulthood.
CEPS may exist in coincidence with other vascular anomalies, including the spectrum of IVC anomalies. Additionally, the type I Abernethy malformations are known to be associated with other congenital anomalies of the heart, spleen, biliary and genitourinary systems.10,12 Aberrations in hepatic vascular flow are known to predispose to the development of focal hepatic abnormalities; regenerative nodules, nodular regenerative hyperplasia, focal nodular hyperplasia (FNH), hepatic adenoma, hepatocellular carcinoma (HCC), and hepatoblastoma have all been described in patients with CEPS.10,12,13
The key imaging finding in extrahepatic portosystemic shunts is an absent or diminutive portal vein at the level of the hepatic hilum with a venous collateral connecting the splanchnic/portal venous and system venous systems (Fig. 14.5). There is often associated enlargement of the hepatic artery. These findings can be identified by ultrasound,
CT, MRI, or catheter angiography. CT and MRI can help map the overall vascular anatomy and provide the broadest field of view for identification of the shunt and associated anomalies. Differentiation of CEPS from acquired portosystemic shunts can be difficult. Imaging findings of portal or splanchnic hypertension, including ascites, splenomegaly, and gastroesophageal varices, are rarely seen in CEPS because of low pressure gradients across the shunt.12,13 Cavernous transformation of the main portal vein also suggests an acquired portosystemic shunt.
CT, MRI, or catheter angiography. CT and MRI can help map the overall vascular anatomy and provide the broadest field of view for identification of the shunt and associated anomalies. Differentiation of CEPS from acquired portosystemic shunts can be difficult. Imaging findings of portal or splanchnic hypertension, including ascites, splenomegaly, and gastroesophageal varices, are rarely seen in CEPS because of low pressure gradients across the shunt.12,13 Cavernous transformation of the main portal vein also suggests an acquired portosystemic shunt.
Management of congenital portosystemic shunts depends on the identification of intrahepatic portal venous branches. The presence of any identifiable portal venous blood flow allows staged closure of the portosystemic shunt, thereby rerouting blood flow through the portal venous system.14,15 Catheter angiography, including direct shunt interrogation, is considered the imaging test of choice in this assessment, although biopsy may ultimately be required.12,14 Screening, diagnosis, and follow-up of hepatic masses in the setting of CEPS is an important function of imaging. Ultrasound can be used to screen for and follow up known lesions, whereas MRI is ideal for characterization of identified lesions. Of note, caution should be exercised in the diagnosis of specific lesions based on dynamic contrast enhancement characteristics, as
the effect of absent portal venous blood flow on enhancement patterns has not been well characterized. Enhancement patterns in the hepatocyte phase with hepatocyte-specific contrast agents are likely unaffected by the altered blood flow.
the effect of absent portal venous blood flow on enhancement patterns has not been well characterized. Enhancement patterns in the hepatocyte phase with hepatocyte-specific contrast agents are likely unaffected by the altered blood flow.
TABLE 14.1 Classification Scheme for Congenital Extrahepatic Portosystemic Shunts | ||
---|---|---|
|
Infectious and Inflammatory Disorders
Infectious Disorders
Infectious diseases of the liver in children consist of viral hepatitis, pyogenic (bacterial) and amebic abscesses, parasitic diseases (e.g., hydatid disease and schistosomiasis), as well as fungal and other granulomatous diseases (e.g., tuberculosis, cat-scratch disease).
Viral hepatitis outside of the neonatal period is most commonly caused by hepatitis A, B, and C viruses, but a vast array of other viruses can infect the liver in childhood, including coxsackie, rubella, Epstein-Barr, varicella-zoster, herpes simplex,
adenovirus, and cytomegalovirus. The diagnosis of viral hepatitis is typically clinical. Ultrasound may show hepatomegaly, heterogeneous parenchymal echotexture, “starry sky” appearance (diffuse decrease in hepatic echogenicity with resultant relative increase in echogenicity of the portal vein walls) (Fig. 14.6), gallbladder wall thickening (Fig. 14.7), and periportal lymph node enlargement. Chronic viral hepatitis conveys an increased risk of cirrhosis and resultant complications, such as portal hypertension and malignancy. Ultrasound is frequently used to screen for these complications.
adenovirus, and cytomegalovirus. The diagnosis of viral hepatitis is typically clinical. Ultrasound may show hepatomegaly, heterogeneous parenchymal echotexture, “starry sky” appearance (diffuse decrease in hepatic echogenicity with resultant relative increase in echogenicity of the portal vein walls) (Fig. 14.6), gallbladder wall thickening (Fig. 14.7), and periportal lymph node enlargement. Chronic viral hepatitis conveys an increased risk of cirrhosis and resultant complications, such as portal hypertension and malignancy. Ultrasound is frequently used to screen for these complications.
Pyogenic (bacterial) liver abscesses may occur secondary to ascending cholangitis, hematogenous spread, or direct spread from an adjacent organ. Immunocompromised children are at increased risk of pyogenic liver abscess formation. Escherichia coli is the most common offending bacterium.16 Abscesses <5 cm in diameter are generally treated with antibiotic therapy alone, whereas larger abscesses often require a combination of antibiotic therapy and percutaneous drainage.16 On ultrasound, pyogenic abscesses typically present as focal liver lesions with variable echogenicity, increased posterior acoustic through transmission, and lack of internal Doppler flow; a multiloculated appearance is common (Fig. 14.8). On contrast-enhanced CT and MR, pyogenic liver abscesses are typically well-defined, lobular, often multiseptated hypoattenuating/fluid signal lesions that may demonstrate rim enhancement and perilesional edema (Fig. 14.8).
Amebic liver abscesses are caused by Entamoeba histolytica, and are the most common extraintestinal complication of amebiasis, occurring in approximately 8.5% of cases.16 Infection is typically secondary to ingestion of contaminated food or water. Treatment of amebic abscesses with amebicidal therapy is highly effective, and percutaneous drainage is rarely required.16 On ultrasound, amebic abscesses are typically peripherally located, hypoechoic
lesions with increased posterior acoustic through transmission and no internal Doppler flow. On contrast-enhanced CT, amebic abscesses typically appear as unilocular, homogenous fluid attenuation masses with an enhancing rim and a surrounding halo of low-attenuation edema (Fig. 14.9).
lesions with increased posterior acoustic through transmission and no internal Doppler flow. On contrast-enhanced CT, amebic abscesses typically appear as unilocular, homogenous fluid attenuation masses with an enhancing rim and a surrounding halo of low-attenuation edema (Fig. 14.9).
Fungal infections of the liver in children most frequently occur in immunocompromised patients, often those with neutropenia, and in patients with lymphoma/leukemia. Candida, Aspergillus, Histoplasma, Cryptococcus, Coccidioides, Nocardia, and Mucor are all known causative agents. Several ultrasound patterns of fungal microabscesses have been described: “wheel-within-a-wheel” pattern (central hypoechoic focus surrounded by echogenic rim), target appearance (central echogenic focus surrounded by hypoechoic rim), diffuse uniform hypoechoic foci, and finally, calcified, hyperechoic foci in the healing/healed phase (Fig. 14.10). On CT, both with and without intravenous contrast material, fungal microabscesses present as multiple, small, hypoattenuating lesions scattered throughout the liver (Fig. 14.11). On MRI, fungal microabscesses appear as multiple, small lesions that are hypointense on T1-weighted MR images and hyperintense on T2-weighted MR images with variable enhancement following intravenous contrast material administration.
Inflammatory Disorders
Neonatal Hepatitis
Neonatal hepatitis refers to the clinical presentation of a neonate with conjugated hyperbilirubinemia related to underlying liver disease.19 Hepatic inflammation does not need to be present but frequently is. Causes of the underlying liver disease are numerous, including infection (e.g., toxoplasmosis, rubella, cytomegalovirus, herpes), metabolic (e.g., α-1-antitypsin deficiency), neoplastic, vascular (e.g., Budd-Chiari syndrome), toxic (e.g., total parenteral nutrition (TPN)-associated cholestasis, drug related), immune (i.e., gestational alloimmune liver disease/neonatal hemochromatosis), structural (e.g., choledochal cyst), and idiopathic.
The role of imaging in neonatal hepatitis is not necessarily to identify the exact etiology of the hepatitis but rather to confirm the diagnosis, exclude extrahepatic biliary atresia, and identify structural abnormalities. Ultrasound is typically the initial imaging modality of choice in this scenario. On ultrasound, the liver may be normal in appearance, although hepatomegaly and increased parenchymal echogenicity may be seen. It is important to identify features of biliary atresia, as this condition requires urgent surgical intervention.
Magnetic resonance cholangiopancreatography (MRCP) and hepatobiliary scintigraphy can also be used in the evaluation of a patient with neonatal hepatitis. Visualization of the extrahepatic bile ducts by MRCP has a sensitivity and specificity of 90% and 77%, respectively, for excluding biliary atresia.20 On hepatobiliary scintigraphy using a 99mTclabeled iminodiacetic acid (IDA) analogue, imaging features consistent with neonatal hepatitis include delayed hepatocyte uptake and washout of the radiotracer with identifiable bowel activity. Delayed images at 4 to 6 and 24 hours should be performed to confirm the presence of excreted radiotracer in the bowel (Fig. 14.12). Hepatobiliary scintigraphy with single photon emission computed tomography (SPECT) was recently shown to have an accuracy of 91.3% in this clinical scenario.21 Ultimately, liver biopsy is often required to add in differentiating neonatal hepatitis from biliary atresia.
Autoimmune Hepatitis
Autoimmune hepatitis is a rare chronic inflammatory disease of the liver that can lead to cirrhosis with a peak incidence in patients 10 to 30 years old.22 In children, the condition sometimes presents along with autoimmune cholangitis. The etiology is uncertain, but is thought to be a combination of genetic, immunologic, and environmental factors.
Imaging studies in general are not helpful in making the specific diagnosis of autoimmune hepatitis but are often helpful in excluding other underlying liver pathology that can result in similar clinical presentations. On ultrasound, the liver may demonstrate nonspecific heterogeneous increased echogenicity (Fig. 14.13), and on contrast-enhanced CT or MR, the liver may demonstrate heterogeneous enhancement. If the disease progresses to cirrhosis, the typical imaging features of cirrhosis and its complications can be seen on cross-sectional imaging modalities. MR elastography and ultrasound elastography may soon play a role in noninvasively detecting progression of fibrosis, thus limiting serial percutaneous liver biopsies (Fig. 14.14).
Neoplastic Disorders
The liver is the third most common site for primary solid abdominal tumors in the pediatric population after the kidneys and adrenal glands.23 Two-thirds of primary pediatric liver tumors are malignant; hepatoblastoma, HCC, and undifferentiated embryonal sarcoma of the liver are the most common.24,25,26 One-third of primary pediatric liver tumors are benign, the most common of which are infantile hepatic hemangioma, FNH, and mesenchymal hamartoma.23,24,26,27
Benign Primary Neoplasms
Infantile Hepatic Hemangiomas
Infantile hepatic hemangiomas (IHHs), sometimes inappropriately called hemangioendotheliomas, are the most common liver tumor in infancy and the third most common liver tumor in children.23,28,29 IHHs are true neoplasms, composed of a network of capillary-sized endothelium-lined vessels, and are distinct from the misnamed adult “hemangiomas,” which are venous malformations.28,29 Most IHHs are asymptomatic.30 Rare complications include high-output congestive heart failure, consumptive coagulopathy (Kasabach-Merritt syndrome), jaundice, hepatic failure, bleeding, abdominal compartment syndrome, and hypothyroidism.24,28
IHHs are classified based on distribution as focal, multifocal, or diffuse (Figs. 14.15, 14.16 and 14.17). Focal IHHs have many of the same characteristics of cutaneous rapidly involuting congenital hemangiomas (RICH). Unlike multifocal and diffuse IHHs that present in the first weeks of life, focal lesions can present in the prenatal period and are congenital. Focal lesions may regress without therapy within the first 12 to 14 months
of life.31 Multifocal and diffuse IHHs are frequently associated with cutaneous infantile hemangiomas.29 Like cutaneous infantile hemangiomas, multifocal and diffuse IHHs appear in the first weeks of life, grow rapidly over the first year, and then slowly involute.29
of life.31 Multifocal and diffuse IHHs are frequently associated with cutaneous infantile hemangiomas.29 Like cutaneous infantile hemangiomas, multifocal and diffuse IHHs appear in the first weeks of life, grow rapidly over the first year, and then slowly involute.29
Imaging can be used to distinguish the subtypes of IHHs and identify sequelae in symptomatic cases. In general, focal and multifocal IHHs appear as well-defined, spherical liver mass(es) (Figs. 14.15 and 14.16). Diffuse IHHs manifest as total or near-total replacement of the liver parenchyma (Fig. 14.17).31,32 Calcifications may be seen in up to 50% of lesions.24 Findings of vascular steal and shunting may be visible as enlarged supplying arteries (celiac and hepatic), tapering of the infraceliac abdominal aorta, enlarged portal veins, early draining hepatic veins, and sequelae of congestive heart failure (e.g., cardiomegaly and pulmonary edema).24,31
On ultrasound, IHHs appear hypoechoic or of mixed echogenicity reflecting central hemorrhage, necrosis or calcification (Fig. 14.15).24 Diffuse IHHs may present as an enlarged heterogeneous liver.24 Doppler interrogation typically demonstrates lesional hypervascularity with variable spectral waveforms.24 IHHs are typically hypoattenuating on nonenhanced CT. On noncontrast MR images, lesions are typically T1-weighted hypointense and T2-weighted
hyperintense (Fig. 14.15).24,31 Internal heterogeneity, including T1-weighted hyperintensity, may be present reflecting hemorrhage or necrosis. Flow voids in and around the IHH reflect high flow within feeding vessels. Postcontrast imaging with CT or MRI is characteristic with peripheral, continuous, or discontinuous arterial enhancement that centripetally fills the lesion over the portal venous phase and more delayed imaging (Figs. 14.15, 14.16, 14.17). Smaller lesions typically enhance homogenously, whereas larger lesions may never enhance completely because of central hemorrhage or necrosis.24 Rare reports of IHHs evaluated with hepatocyte-specific contrast agents have described the lesions to be variable in their retention of contrast on the hepatocyte phase of imaging.33
hyperintense (Fig. 14.15).24,31 Internal heterogeneity, including T1-weighted hyperintensity, may be present reflecting hemorrhage or necrosis. Flow voids in and around the IHH reflect high flow within feeding vessels. Postcontrast imaging with CT or MRI is characteristic with peripheral, continuous, or discontinuous arterial enhancement that centripetally fills the lesion over the portal venous phase and more delayed imaging (Figs. 14.15, 14.16, 14.17). Smaller lesions typically enhance homogenously, whereas larger lesions may never enhance completely because of central hemorrhage or necrosis.24 Rare reports of IHHs evaluated with hepatocyte-specific contrast agents have described the lesions to be variable in their retention of contrast on the hepatocyte phase of imaging.33
IHH is a benign tumor with a good prognosis. Asymptomatic pediatric patients should be followed with ultrasound to document regression.31 Symptomatic pediatric patients may be treated pharmacologically (e.g., propranolol) to promote lesion regression.31 Hepatic artery embolization, partial liver resection, or liver transplant may be needed in rare complicated cases.31
Focal Nodular Hyperplasia
Focal nodular hyperplasia (FNH) accounts for 2% of pediatric liver tumors.34 This tumor-like cellular proliferation occurs both sporadically and at an increased rate in long-term survivors of pediatric malignancies (e.g., neuroblastoma, leukemia).34,35,36,37,38,39,40 Sporadic FNH are typically solitary, whereas multiple lesions are common in cancer survivors.34 FNH is possibly induced by local abnormalities in blood flow. Grossly and microscopically, FNH resembles a localized form of cirrhosis with fibrous bands and nodular collections of hepatocytes accompanied by proliferating bile ductules. Sporadic FNH are generally larger and more likely to have the classic central stellate scar compared with those arising after treatment for a pediatric malignancy (Fig. 14.18).34
On ultrasound, FNH typically appears homogeneous with variable echogenicity (Fig. 14.19).24 The central scar, if present, generally appears hyperechoic compared to the mass. Doppler interrogation may show internal vessels with arterial waveforms, which has been described as a distinguishing feature versus hepatic (hepatocellular) adenoma.24 On non-contrast CT, FNH is typically iso- or slightly hypoattenuating compared to normal liver with the central scar appearing
hypoattenuating compared to the mass. On MRI, FNH is typically iso- to slightly hypointense on T1-weighted MR images and iso- to slightly hyperintense on T2-weighted MR images (Fig. 14.19). The central scar is typically hypointense to the mass on T1-weighted MR images and slightly hyperintense on T2-weighted MR images. This differs from the central scar seen in fibrolamellar HCC (FLH), which is commonly hypointense on T2-weighted MR images.
hypoattenuating compared to the mass. On MRI, FNH is typically iso- to slightly hypointense on T1-weighted MR images and iso- to slightly hyperintense on T2-weighted MR images (Fig. 14.19). The central scar is typically hypointense to the mass on T1-weighted MR images and slightly hyperintense on T2-weighted MR images. This differs from the central scar seen in fibrolamellar HCC (FLH), which is commonly hypointense on T2-weighted MR images.
![]() FIGURE 14.18 Focal nodular hyperplasia, manifesting as a 7 cm mass with a characteristic central white fibrous scar in an otherwise healthy adolescent girl. |
The enhancement pattern of FNH on CT and MRI is characteristic with early, intense arterial enhancement with the lesion becoming isointense to the liver in the portal venous and delayed phases (Fig. 14.19). The central scar typically enhances on the delayed phase of imaging (versus the non-enhancing scar of FLH). Hepatocyte-specific contrast agents provide specificity for the diagnosis of FNH as it is one of the few lesions to retain contrast material on the hepatocyte phase of imaging (Fig. 14.19).33 The central scar does not retain contrast during the hepatocyte phase, appearing
hypointense to the surrounding lesion.33 Large FNHs may demonstrate evidence of arteriovenous shunting on CT and MRI, including enlarged hepatic artery branches in and surrounding the lesion and early hepatic venous opacification.
hypointense to the surrounding lesion.33 Large FNHs may demonstrate evidence of arteriovenous shunting on CT and MRI, including enlarged hepatic artery branches in and surrounding the lesion and early hepatic venous opacification.
Asymptomatic lesions require no follow-up. Surgical resection or hepatic artery embolization of symptomatic lesions can be considered.
Mesenchymal Hamartoma
Mesenchymal hamartoma of the liver is the second most common benign pediatric liver tumor, accounting for 5% to 8% all liver masses.41 Eighty percent are diagnosed before 2 years of age and ninety-five percent before 5 years of age24,41; in utero development has been described. Affected pediatric patients typically present with a rapidly enlarging, painless abdominal mass.42 Ascites, jaundice, and congestive heart failure can occur if the mass compresses adjacent structures.41 Mild elevation of alpha fetoprotein (AFP) level has been described, but there is no specific laboratory marker for mesenchymal hamartoma.24
Imaging features depend on tumor composition. Lesions can range in appearance from predominantly cystic to predominantly solid with 80% to 85% of tumors having macroscopic cysts.24,42 These tumors are typically large at presentation, with an average diameter of 16 cm, and they are more likely to arise in the right lobe of the liver than the left.41,42 If identified on prenatal ultrasound, mesenchymal hamartomas typically appear anechoic to hypoechoic. There may be associated placental thickening, multicystic enlargement, or mesenchymal stem villous hyperplasia.41 Postnatally, the cystic portions of mesenchymal hamartomas appear anechoic with echogenic septations on ultrasound. Low-level echoes can be seen within the cysts, reflecting the gelatinous content.24 Solid portions of the mass are generally hyperechoic compared to background liver. Doppler ultrasound typically shows little blood flow.
On CT, mesenchymal hamartoma typically appears as a complex cystic mass with the cystic components similar in attenuation to water (10-20 HU) (Fig. 14.20).24 On noncontrast CT, the stromal components of the mass are generally hypoattenuating compared to normal liver, but hyperattenuating compared to the cystic portions. Calcifications are rare. Following intravenous contrast material administration, the septations and stromal components enhance (Fig. 14.20). On MRI, the cystic components of the lesion are usually hypointense on T1-weighted MR images and hyperintense on T2-weighted MR images (Fig. 14.20). Cysts may have variable signal intensity, particularly on T1-weighted MR images, depending on the proteinaceous content of the fluid.24 The solid component of the mass is typically hypointense on T1-weighted and T2-weighted MR images reflecting fibrous tissue24 and enhances on postcontrast imaging (Fig. 14.20).
Histologically, mesenchymal hamartoma is composed of primitive stellate mesenchymal cells in a myxoid matrix with admixed hepatocytes, ductular structures, and islands of hematopoietic cells; cysts are frequently seen (Fig. 14.21).41,42 Recurrent clonal cytogenetic abnormalities, in particular a t(11;19) chromosomal translocation, are thought to reflect a neoplastic biology.43,44 The natural history of mesenchymal hamartoma is to rapidly enlarge in the first several months of life before stabilizing or transitioning to a phase of slow growth.24 Spontaneous regression has been described, but observation is discouraged because of the small risk of “malignant transformation” to undifferentiated embryonal sarcoma.24,41 Surgical resection is considered definitive therapy.
Hepatic Adenoma
Hepatic (hepatocellular) adenomas are uncommon in the pediatric population. Lesions can be seen in young women on oral contraceptives and in obese patients. In addition, hepatic adenomas can present in association with altered hepatic blood flow and systemic diseases, such as Fanconi anemia (related to androgen therapy), glycogen storage disease types I and III, galactosemia, familial diabetes mellitus, and familial adenomatous polyposis.24 Patients with more than nine hepatic adenomas are said to have hepatic adenomatosis, which can be unilobar, massive, or multifocal.45 Hepatic adenomas are most often asymptomatic. Tumor rupture, however, occurs in 10% to 35% of affected patients manifesting as acute pain, intraperitoneal hemorrhage, or hypovolemic shock.24,45
Hepatic adenomas are composed of sheets of hepatocytes without portal tracts or normal bile ducts (Fig. 14.22), and they are supplied by the hepatic artery. Recent studies have subclas-sified hepatic adenomas based on the presence of genetic mutations (HNF-1α or β-catenin mutation) or histologically based on the presence or absence of inflammation. Inflammatory hepatic adenomas are the most common subtype (40% to 50%) and occur more commonly in association with oral
contraceptive use and obesity.46 Systemic symptoms of chronic anemia and a systemic inflammatory syndrome (fever, leukocytosis, and elevated serum levels of C-reactive protein) have been reported.46 HNF-1α mutated hepatic adenomas (30% to 35%) occur exclusively in girls, with many having a history of oral contraceptive use.46 A background of steatosis is common, and 50% are multiple.46 This mutation results in promotion of lipogenesis and accumulation of lesional intracellular lipid, which is apparent by imaging. β-catenin mutated hepatic adenomas (10% to 15%) occur more commonly in boys and are associated with male hormone administration, glycogen storage disease, and familial adenomatous polyposis.46 Unclassified adenomas have no specific associations.
contraceptive use and obesity.46 Systemic symptoms of chronic anemia and a systemic inflammatory syndrome (fever, leukocytosis, and elevated serum levels of C-reactive protein) have been reported.46 HNF-1α mutated hepatic adenomas (30% to 35%) occur exclusively in girls, with many having a history of oral contraceptive use.46 A background of steatosis is common, and 50% are multiple.46 This mutation results in promotion of lipogenesis and accumulation of lesional intracellular lipid, which is apparent by imaging. β-catenin mutated hepatic adenomas (10% to 15%) occur more commonly in boys and are associated with male hormone administration, glycogen storage disease, and familial adenomatous polyposis.46 Unclassified adenomas have no specific associations.
In general, hepatic adenomas appear well-defined by ultrasound with variable echogenicity depending on the amount of intralesional lipid, hemorrhage, and glycogen as well as the echogenicity of adjacent liver (Fig. 14.23).24 On noncontrast CT, hepatic adenomas appear as well-defined, homogeneously hypoattenuating lesions (Fig. 14.23). Lesions that have bled may show internal hyperattenuation (Fig. 14.24). Calcifications are uncommon (5% to 15%).24 On postcontrast imaging, hepatic adenomas show arterial enhancement with a variable appearance on delayed phase imaging. By MRI, the classic appearance of a hepatic adenoma is a lesion that is heterogeneously T1-weighted hyperintense and mildly T2-weighted hyperintense, and that shows loss of signal on T1-weighted gradient-recalled echo out-of-phase (opposed phase) imaging (Fig. 14.23). On post-contrast imaging, hepatic adenomas classically show arterial enhancement reflecting hepatic arterial supply and no uptake of hepatocyte-specific contrast agents during the hepatocyte phase of imaging (Fig. 14.23). Loss of signal on T1-weighted gradient-recalled echo opposed phase imaging can be seen in some subtypes of hepatic adenomas (HNF-1α) (Fig. 14.23)
and some hepatic adenomas can retain contrast (though generally less than normal background liver) on the hepatocyte phase of imaging (β-catenin mutated). Specific imaging features have not been well-defined for β-catenin mutated and unclassified hepatic adenomas; however, there is some evidence to suggest that MRI can distinguish the inflammatory and HNF-1α mutated hepatic adenoma subtypes.46
and some hepatic adenomas can retain contrast (though generally less than normal background liver) on the hepatocyte phase of imaging (β-catenin mutated). Specific imaging features have not been well-defined for β-catenin mutated and unclassified hepatic adenomas; however, there is some evidence to suggest that MRI can distinguish the inflammatory and HNF-1α mutated hepatic adenoma subtypes.46
The two main complications of hepatic adenomas are bleeding and degeneration to HCC (Fig. 14.24). The risk of these complications depends on the subtype. Inflammatory hepatic adenomas are the most likely to bleed, with the risk of hemorrhage increasing with the size of the tumor.46 HNF-1α mutated hepatic adenomas are the least aggressive and have the smallest risk of hemorrhage or malignant degeneration. β-catenin mutated hepatic adenomas have the highest risk of malignant transformation to HCC. Risk factors that increase the likelihood of malignant transformation include male gender, glycogen storage disease, anabolic steroid use, β-catenin subtype, and size larger than 5 cm.46
Hepatic adenomas are first treated with discontinuation of hormonal therapy (e.g., oral contraceptives).24 Lesions larger than 5 cm and those that enlarge or fail to regress are typically surgically resected. Radiofrequency ablation has been used to treat small hepatic adenomas.47 Depending on the size, location, and number of hepatic adenomas, orthotopic liver transplant may be performed.45
Malignant Neoplasms
Hepatic Metastasis
Metastases are the most common malignant tumor to involve the liver in children and are most commonly seen with neuroblastoma and Wilms tumor. Metastases can be solitary or multifocal, and their appearance on imaging is variable. On MRI, if hepatocyte-specific contrast agents are used, metastases show no uptake of contrast material during the hepatocyte phase because of the absence of functioning hepatocytes in the lesions (Fig. 14.25). Hepatic metastases also commonly restrict diffusion of water on diffusion-weighted imaging (Fig. 14.25). Although uncommon, lymphoma can also involve the pediatric liver (Fig. 14.26).
Hepatoblastoma
Hepatoblastoma is the most common primary hepatic malignancy in childhood, accounting for between 65% and 80% of all pediatric liver tumors.27,48,49 Median age at diagnosis is 18 months,48 and 90% to 95% of cases are diagnosed before 4 years of age.26,48 Risk factors for hepatoblastoma include male gender, genetic syndromes (familial adenomatous polyposis coli, Beckwith-Wiedemann, hemihypertrophy,
and trisomy 18), low birth weight, and prematurity.25,50,51 Of pediatric patients with hepatoblastoma, 6.4% have an associated congenital anomaly.50 Children with hepatoblastoma usually present with a palpable abdominal mass, and sometimes with nonspecific anorexia and weight loss.25,27 Rarely, affected pediatric patients present with precocious puberty/virilization due to secretion of human chorionic gonadotropin (β-HCG).27 Serum AFP is elevated in 90% of patients; tumors that do not express AFP have a worse prognosis.25,26
and trisomy 18), low birth weight, and prematurity.25,50,51 Of pediatric patients with hepatoblastoma, 6.4% have an associated congenital anomaly.50 Children with hepatoblastoma usually present with a palpable abdominal mass, and sometimes with nonspecific anorexia and weight loss.25,27 Rarely, affected pediatric patients present with precocious puberty/virilization due to secretion of human chorionic gonadotropin (β-HCG).27 Serum AFP is elevated in 90% of patients; tumors that do not express AFP have a worse prognosis.25,26
Histologically, hepatoblastoma is classified into two types, epithelial and mixed.25 The epithelial type of hepatoblastoma is the most common and consists of primitive hepatocytes mimicking those seen in embryonal or fetal life (Fig. 14.27). When mesenchymal elements, such as bone, cartilage, or adipose tissue, are admixed, the tumor is termed a mixed type. In general, tumor behavior is linked to the degree of hepatocyte differentiation, with the most “mature” tumors (pure fetal pattern) having a better prognosis and small cell undifferentiated pattern correlating with a worse prognosis.
The role of imaging in hepatoblastoma is to confirm the diagnosis, stage the disease, and assist in surgical planning. Attention should be paid to hepatic sectional and vascular anatomy as these determine disease stage and resectability. CT of the chest should be performed in all cases, as lung is the most common site of metastatic disease.52
On ultrasound, the appearance of hepatoblastoma is variable. The tumor is usually heterogeneously hyperechoic compared to background liver (Fig. 14.28).52 Calcifications and necrosis may be identifiable.52 Color Doppler can be useful to assess location and involvement of vascular structures. On noncontrast CT, hepatoblastoma typically appears as a well-defined hypoattenuating mass compared to normal background liver.25 Calcifications are visible in approximately 50% and are usually small and fine in epithelial tumors and
coarse in mixed mesenchymal-epithelial tumors.25,52 The MRI appearance of hepatoblastoma depends on the histologic subtype. Epithelial tumors typically appear homogeneously hypointense on T1-weighted MR images and hyperintense on T2-weighted MR images.52 Mixed epithelial-mesenchymal tumors are more often heterogeneous because of the presence of intralesional calcification, cartilage, and fibrous septa (Fig. 14.29).52
coarse in mixed mesenchymal-epithelial tumors.25,52 The MRI appearance of hepatoblastoma depends on the histologic subtype. Epithelial tumors typically appear homogeneously hypointense on T1-weighted MR images and hyperintense on T2-weighted MR images.52 Mixed epithelial-mesenchymal tumors are more often heterogeneous because of the presence of intralesional calcification, cartilage, and fibrous septa (Fig. 14.29).52
Following intravenous contrast material administration, rim and septal enhancement may be seen early on CT and MRI (Fig. 14.28), with the tumor appearing hypoenhancing relative to background liver during the delayed phases (Fig. 14.29).25,52 Attention should be paid to the hepatic vasculature and IVC to exclude bland or tumor thrombus. Hepatoblastoma can have a variable appearance on the hepatocyte phase of imaging if hepatocyte-specific contrast agents are used.33 The majority of tumors show decreased uptake with a small number showing variable retention of contrast.35 Intrahepatic satellite lesions can occur.
The most commonly used staging system is the pretreatment extent of disease (PRETEXT) system. Primary tumor staging under this system reflects the number of liver sections that need to be resected to completely excise the tumor. The PRETEXT number is obtained by subtracting the number of contiguous tumor-free sections of the liver from four.53,54 In addition to describing the anatomic extent of the tumor, the PRETEXT staging system includes other criteria defining involvement of the IVC or hepatic veins, portal vein, extrahepatic disease, and distant metastases.53
Pediatric patients with hepatoblastoma are treated with either up-front surgical resection or neoadjuvant chemotherapy followed by surgery.55 Patients treated with cisplatin-based chemotherapy and complete surgical resection have a 5-year survival rate of 80% to 90%.56 Ten to twenty percent of tumors are unresectable and are treated with neoadjuvant chemotherapy followed by liver transplantation.56 Overall survival in this group ranges from 82% to 100%.56
Hepatocellular Carcinoma
HCC is the second most common primary pediatric liver malignancy, accounting for <25% of hepatic malignancies.25 HCC generally occurs in older children and adolescents (>10 years of age).48 Known risk factors for developing HCC during childhood include cirrhosis, chronic hepatitis B or C infection, type 1 glycogen storage disease, tyrosinemia, hemochromatosis, alpha-1 antitrypsin deficiency, autoimmune hepatitis, primary sclerosing cholangitis (PSC), biliary atresia, familial cholestatic jaundice, Wilson disease, Alagille syndrome, neurofibromatosis, ataxia-telangiectasia, and Fanconi anemia.25,26,48 Preexisting liver disease, however, is present in only 30% to 50% of patients.25,57 Children with HCC usually present with a large abdominal mass and elevated serum AFP levels.25,58
Like in hepatoblastoma, the role of imaging in HCC is to confirm the diagnosis, stage the disease, and assist in surgical planning. In general, there are three patterns of primary tumor growth in HCC: a solitary mass, multinodular tumor, and diffuse infiltrative tumor [6]. Metastatic disease is present in 25% to 50% of patients at the time of diagnosis and most commonly occurs in the lungs.58,59
Small HCCs are generally hypoechoic by ultrasound, but can be variable in appearance.25 On noncontrast CT, hypoattenuation is typical. On MRI, lesions are typically predominantly hypo- to isointense on T1-weighted MR images and slightly hyperintense on T2-weighted MR images. However, large lesions can appear homogeneous or heterogeneous by any of these modalities depending on the presence of internal macro- and microscopic fat, hemorrhage, and/or necrosis.25
HCCs are supplied by the hepatic artery, and, thus, show arterial phase hyperenhancement.25,58 On the portal venous phase of imaging, HCC can be variable in appearance.25,58 With hepatocyte-specific contrast agents, HCC typically shows decreased uptake on the hepatocyte phase, although well-differentiated HCC may appear hyperintense relative
to background liver.33 Careful attention must be paid to the hepatic vasculature to exclude tumor invasion (Fig. 14.30).
to background liver.33 Careful attention must be paid to the hepatic vasculature to exclude tumor invasion (Fig. 14.30).
Children with HCC have a poor prognosis with an overall survival rate of 10% to 30%.25,59 Survival is largely dependent on tumor resectability, but two-thirds of patients have unresectable disease at diagnosis.25 HCC is relatively chemotherapy resistant with 30% to 50% of affected pediatric patients showing some response.57,60 Alternative treatment strategies include radiofrequency ablation, transarterial chemoembolization, 90Y radioembolization, and liver transplantation. The 5-year survival rate of childhood HCC treated with liver transplantation is 63% to 83%.61,62
Fibrolamellar Hepatocellular Carcinoma
Fibrolamellar hepatocellular carcinoma (FLH) is a rare (0.85% of all primary liver malignancies) variant of HCC with unique clinical, imaging, and histologic features. The tumor is more common in adolescents and young adults with a peak incidence near 25 years of age.63 Pediatric patients with FLH typically present with an abdominal mass with occasional gynecomastia or jaundice.25 Most affected pediatric patients do not have underlying liver disease.64 Serum AFP is usually not elevated, but serum B12, unsaturated B12-binding capacity, and serum neurotensin are elevated.64,65,66 FLH most often occurs as a solitary mass, more commonly in the left lobe of the liver.64 A central stellate or amorphous scar is present in 33% to 60%25,63,66 (Fig. 14.31). Lymph node metastases are common.
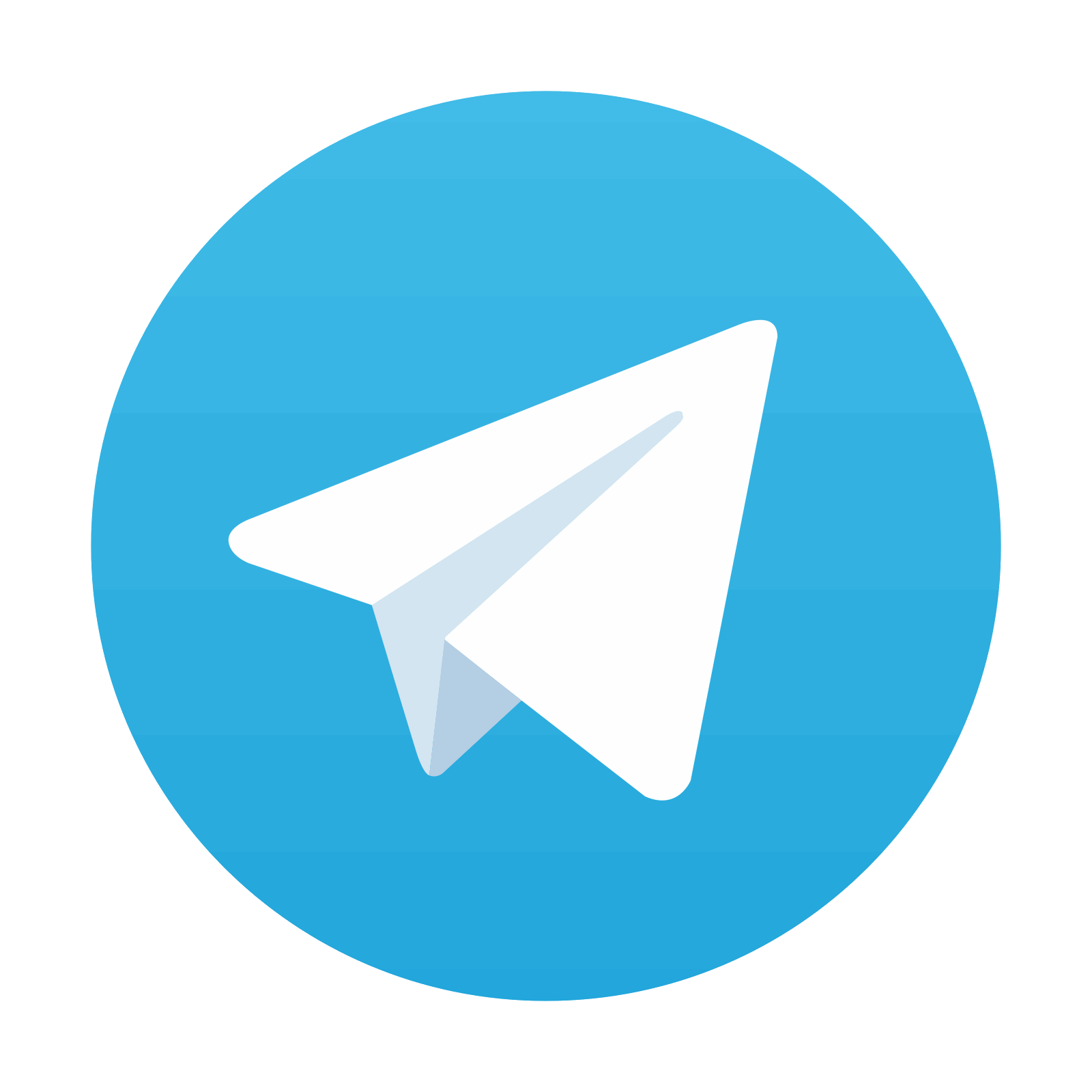
Stay updated, free articles. Join our Telegram channel

Full access? Get Clinical Tree
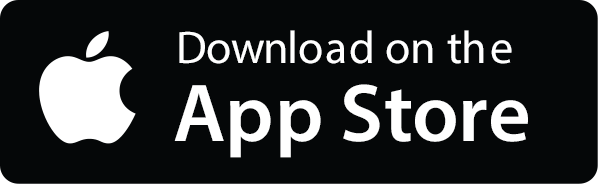
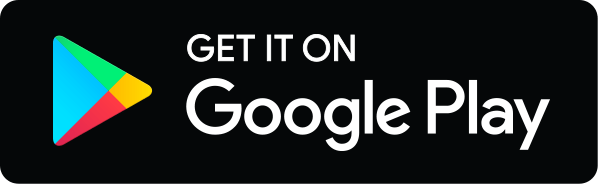
