, Valery Kornienko2 and Igor Pronin2
(1)
N.N. Blockhin Russian Cancer Research Center, Moscow, Russia
(2)
N.N. Burdenko National Scientific and Practical Center for Neurosurgery, Moscow, Russia
Lung cancer (LC) is the most common malignant neoplasm in the world’s population and, as a cause of cancer mortality, amounts to almost 1.6 million people annually. More than 1.8 million of new cases of lung cancer are registered each year worldwide. In Russia, lung cancer is diagnosed each year in more than 55,000 patients (43.4 per 100 thousand). According to Davydov and Axel (2014a, b) the incidence of lung cancer, taking into account all age groups, was 18.7% in 2012 and put lung cancer at the first place in the structure of prevalence of malignant neoplasms in Russia among men. There are two major forms of lung cancer—small cell (SCLC) and non-small cell lung cancer (NSCLC): the latter accounts for up to 80% of total cases. Both forms of lung cancer have different degrees of biological activity, which are reflected in the approaches to the selection of diagnostic and treatment methods (Spencer 1977; Trachtenberg 1987; Davydov and Polotsky 1994; Jänne et al. 2002; Ramazanova and Kislichko 2012; Peters et al. 2012; Rossi et al. 2014; Folkert and Timmerman 2015). The development of lung cancer is believed to be associated with smoking: approximately 90% of affected men and 80% of affected women. At the time of diagnosis, the disease in most patients has common forms.
By the incidence of metastases to the brain, lung cancer is on the first place among all malignant tumors: according to different data, it accounts for 30–60% of all metastases in the brain (to our knowledge, not less than 25%). Up to half of lung cancer patients have a high risk of developing metastatic brain lesions (Takakura et al. 1982; Sen et al. 1998). Based on the study of case records in 2410 patients with lung cancer, brain lesions were identified in 790 (32.9%) patients, with solitary metastases being more common in adenocarcinoma than in poorly differentiated cancer (Martynov and Idrisova 1981). Alperovich (1975) noted that poorly differentiated cancer metastasizes more often. The time interval from the diagnosis of lung cancer to the detection of MTSs in the brain is on average 2–9 months, which is slightly shorter than with cancers from other sites (Sundaresan and Galicich 1985; Nussbaum et al. 1996). The median survival rate of untreated patients with lung cancer MTSs in the brain ranges from 6 to 12 weeks (Carney 1999). Lung cancer metastases in the brain appear to be more common in men.
According Chute et al. (1985), SCLC accounts for 18–30% of all cases of lung cancer. This type of tumor is characterized by an early and rapid spread of metastases via intrathoracic lymph nodes, mediastinal lymph nodes, as well as to the brain, liver, skeletal bone, and adrenals. Jereczek et al. (1996) noted that an autopsy found distant metastases in 143 (82%) cases in the group of 174 patients who died from SCLC, including in the liver (49%), adrenal glands (25%), peripheral lymph nodes (21%), kidneys (18%), brain (17%), and pancreas (12%). This is why a brain MRI study with contrast enhancement in confirmed SCLC is included in the mandatory investigation protocol.
NSCLC comprises several groups: adenocarcinoma (40%), squamous cell carcinoma (30%), and large cell cancer, bronchoalveolar cancer, and other subtypes which account for up to 30% of cases. The proportion of cases of metastatic squamous cell carcinoma was found to increase to up to 80% with the 2-year survival of patients. Literature describes cases of simultaneous presence of different morphological NSCLC forms and neuroendocrine variants, which greatly complicate an accurate diagnosis. In stage IV NSCLC, the overall prognosis is unfavorable: a 1-year survival rate in such cases does not exceed 20% (Riquet et al. 1997). Metastases in the brain occur in one third of NSCLC patients and often manifest by multiple lesions, while the latter can be located both sub- and supratentorially.
Metastatic lesions in the meninges (carcinomatosis) in patients with lung cancer are less common than, for example, in breast cancer (Grossman et al. 1993; Grossman and Krabak 1999); however, according to Mahajan et al. (2002), the source of metastases was lung cancer in 50% of 101 patients with confirmed meningeal involvement.
Clinical symptoms in intracranial metastases of lung cancer include headache, vomiting, motor and sensory deficits, seizures, behavioral and mental disorders, and aphasia. However, these symptoms can often be “subdued,” which complicates the early clinical diagnosis of the lesion. Thus, according to Jena et al. (2008) in 46.7% of cases of 62 patients with lung cancer metastases in the brain, there were no neurological symptoms.
The method of choice for primary diagnosis of metastatic brain lesions of any etiology is MRI; if it cannot be performed, a viable alternative is CT.
Lung cancer metastases in a CT study performed without contrast enhancement often look like isodense lesions or lesions with a reduced (in the cystic form) density. The size of a perifocal edema area relative to the sizes of the lesion varies greatly up to the absence of edema as such.
Administration of a contrast agent in a CT study is a must, because a solid part of lung cancer metastases rapidly accumulates the contrast agent. Small, convexitally located lesions not always are visualized even after the CA administration.
A CT perfusion study in lung cancer metastases in the brain allows to obtain mean values (CBV = 11.27 ± 3.21 ml/100 g) of the blood flow volume similar to those in metastases of breast cancer, colon cancer , and uterine cancer in the brain. CBF values representing the blood flow velocity in the depth of a metastatic tumor in lung cancer have similar mean values (CBF = 85.03 ± 19.56 ml/100 g/min) as compared with the mean CBF values detected in patients with MTSs of other origin. A solid part of lung cancer metastases in the brain is characterized by relatively low (8.83 ± 2.85 s) MTT as compared to MTT in other metastatic lesions.
Despite the large number of conducted MRI studies, we were unable to establish any MR trends in manifestations of lung cancer metastases in the brain, although we identified a number of pathognomonic MRI manifestations in cases of metastatic brain lesions of another origin (melanoma , kidney cancer , colon cancer). The MR picture of metastatic brain lesions in lung cancer is similar to MRI manifestations of MTSs of tumors from other sites.
Metastatic brain lesions in lung cancer in most cases have a cystic nature with the presence of an area of moderate perifocal edema and are characterized by an increased signal on T2-weighted MRI. However, in our material, metastatic lesions with both a solid and cystic-solid structure with a small area of perifocal edema were identified. In cases where there is an acute hemorrhage in the metastasis stroma in T2-weighted images, there are small hypointense areas, and the tumor tissue becomes heterogeneous. In cases where the metastatic lesion has a solid structure, T2-weighted images show an iso-hypointense MR signal.
T1-weighted MRI allows to display manifestations typical for the active tumor growth. A hyperintense and heterogeneous MRI signal on T1-weighted images is generally caused by the presence of a hemorrhage in the metastasis.
In lung cancer, in cases of multiple metastatic lesions, tumor lesions can be located in a chaotic fashion throughout the brain, both sub- and supratentorially. We did not identify any site “characteristic” for solitary lung cancer metastases in our clinical material. At the same time, multiple microfoci were noted in the brain parenchyma with lung adenocarcinoma. In such cases, a perifocal edema is usually insignificant, which can be partly attributed to the typical manifestations of multiple parenchymal metastases in this morphological type of lung cancer.
Metastases to the skull bones and vertebral bodies can occur not only via the systemic blood flow but also along the vertebral venous plexuses, a valveless system of venous anastomoses running along the spine from the brain to the lesser pelvis. Particular attention in the analysis of diagnostic images should be paid to the occipital bone. The analysis of bone structures in a CT study is mandatory, as metastases to the skull bones in lung cancer occur in up to 30% of cases. In typical cases, a characteristic area of bone destruction in combination with the soft tissue component is visualized. In case of small lesions in the cranial bones, especially its vault, it is difficult to differentiate the metastases from emissary veins.
When the bony structures of the skull base are affected, there is typically a combination of a bone destruction focus with a soft tissue tumor component that is intensely enhanced after intravenous administration of a contrast agent. In such cases, T1-weighted MRI with fat suppression should be used before and after administration of gadolinium preparations. In dural dissemination of metastatic lesions, the symptom of a “dural tail” can be visualized. It is observed on MRI in case of local invasion of the dura mater on the periphery of the main lesion.
We successfully used proton MR spectroscopy (PMRS) as a supplementary option in protocols of differential diagnosis of brain metastatic lesions from primary lesions of tumor and non-tumor origin. However, the prevalence of the Lip-Lac complex characteristic of the tumor lesions, displaying the fact of necrotic changes in the tumor tissue, due to its resemblance to chronic post-radiation changes also accompanied by necrosis and an increased Lip-Lac complex does not allow to consider MR spectroscopy as a method of reliable differential diagnosis of metastases in the brain from formed radiation necrosis after radiotherapy. This statement is fully true for lung cancer metastases in the brain.
SWI (SWAN) images in lung cancer metastases showed a small or moderate number of abnormal vessels, which generally did not result in a significant decrease in the MR signal in this sequence.
In patients diagnosed with primary metastatic tumor in the brain, a one-study whole-body DW MRI provided information on the primary tumor site and extent of the disease in general. Thus, in our studies, whole-body DW MRI in patients with peripheral lung cancer usually clearly detected a primary site of the tumor (both on PET and DWI). Furthermore, besides the primary lesion in the lungs, small metastases with sizes of about 7 mm were identified in some cases. This demonstrates the high diagnostic sensitivity of DW technology.
When analyzing the results of these studies, we observed that the size and shape of lesions on DWI MRI and whole-body PET were often not the same, differing both in the direction of an increase and a decrease. A decrease in the size of lesions may be associated with chest excursions during respiration and a partial loss of changes on MRI. From our point of view, an enlargement in high-signal areas on MRI may be caused by concomitant atelectasis, the presence of cysts and hemorrhages in the lesion stroma.
The whole-body DW MRI has high sensitivity in malignant lung lesions—85% of lesions detected with PET. However, this method has insufficient (76%) specificity due to a large number (24% in our material) of false-positive results.
A whole-body FDG-PET study by Yap et al. (2002) in patients with bronchoalveolar lung cancer showed a fairly high (95%) specificity of the method as compared to CT. However, it was noted that the use of PET with the superficial location of the tumor in the bronchus lumen (without any signs of significant invasion) was less effective. Similar results, confirming the superior specificity of PET, were shown by Vansteenkiste and Stroobants (1998) in a comparative study of the diagnostic efficiency of PET and CT in relation to distant NSCLC metastases in the lymph nodes.
According to our data, the use of PET/CT with 18F-FDG in lung cancer is justified only to assess extracranial lesions. Due to mostly cystic and microfocal brain lesions, the drug accumulation foci in the solid part of lung cancer metastases merge with the physiological accumulation of 18F-FDG. Products with low accumulation in the brain parenchyma, such as 18F-thymidine, 18F-Tyrosine, and 11C-methionine, are significantly superior to 18F-FDG in investigation of intracranial lesions. Hara and Inagaki (2000) investigated the possibility of PET diagnostics with 11C-choline and 18FDG in patients with NSCLC. 11C-choline sensitivity appeared to be 100%, while that of FDG was 75%, which indicates higher efficiency of 11C-choline in the diagnosis of NSCLC and its distant metastases.
12.1 Clinical Observations
See Figs. 12.1, 12.2, 12.3, 12.4, 12.5, 12.6, 12.7, 12.8, 12.9, 12.10, 12.11, 12.12, 12.13, 12.14, 12.15, 12.16, 12.17, 12.18, 12.19, 12.20, 12.21, 12.22, 12.23, 12.24, 12.25, 12.26, 12.27, 12.28, 12.29, 12.30, 12.31, 12.32, 12.33, 12.34, 12.35, 12.36, 12.37, 12.38, 12.39, 12.40, 12.41, 12.42, 12.43, and 12.44.
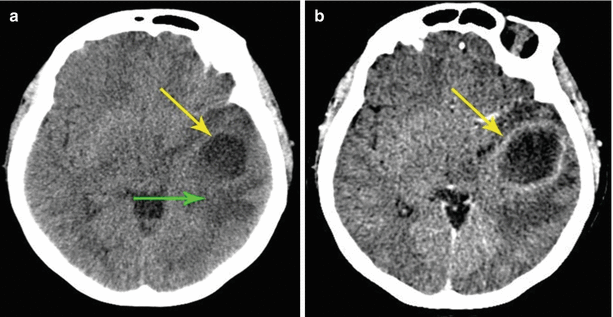
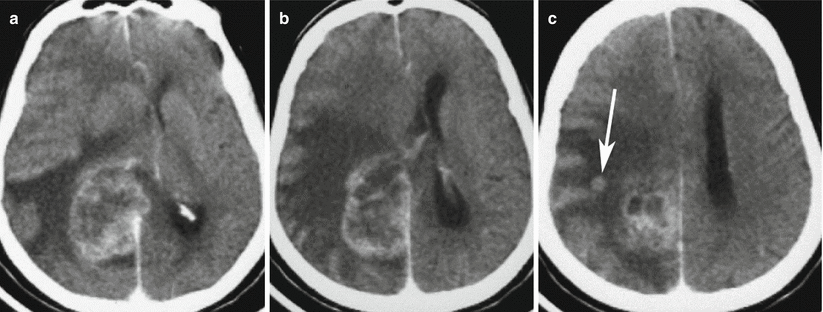
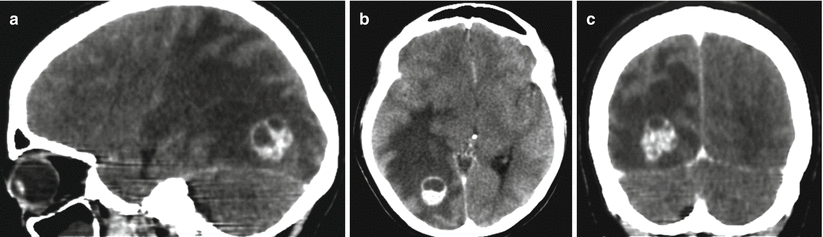
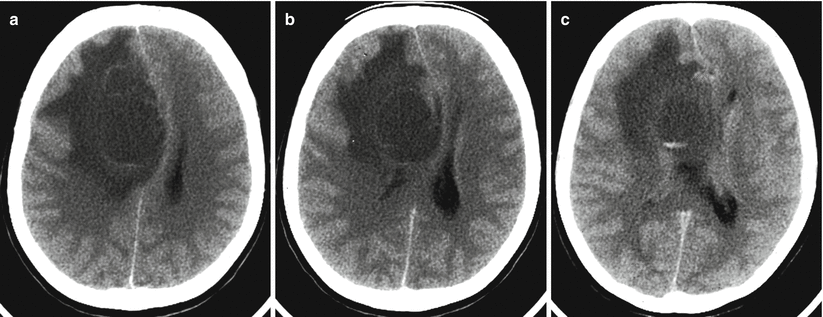
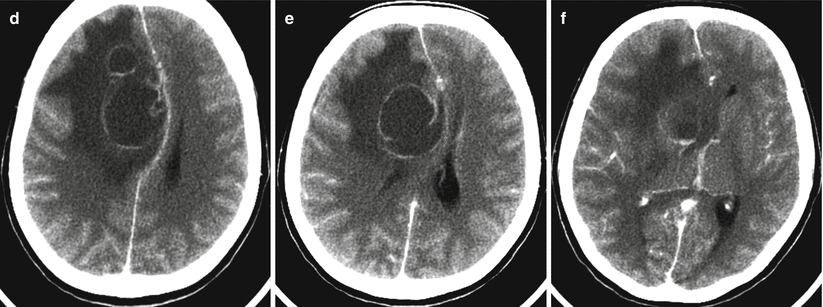
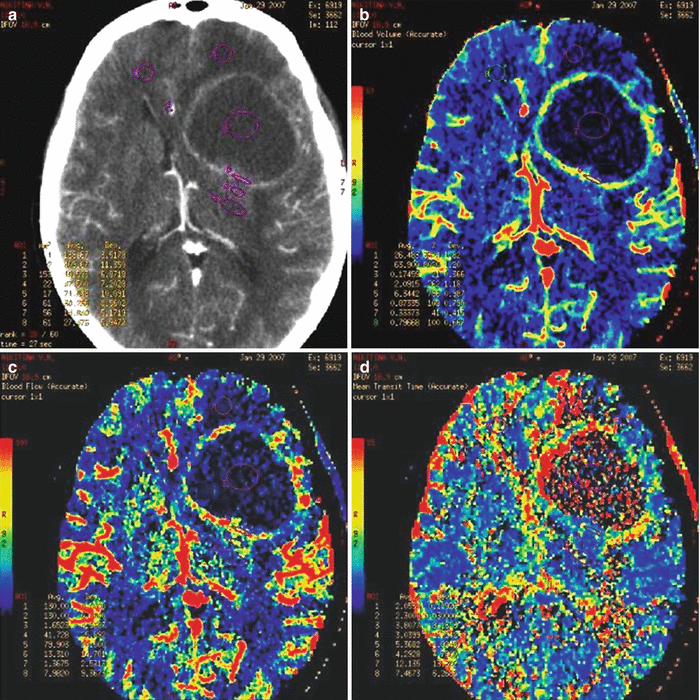
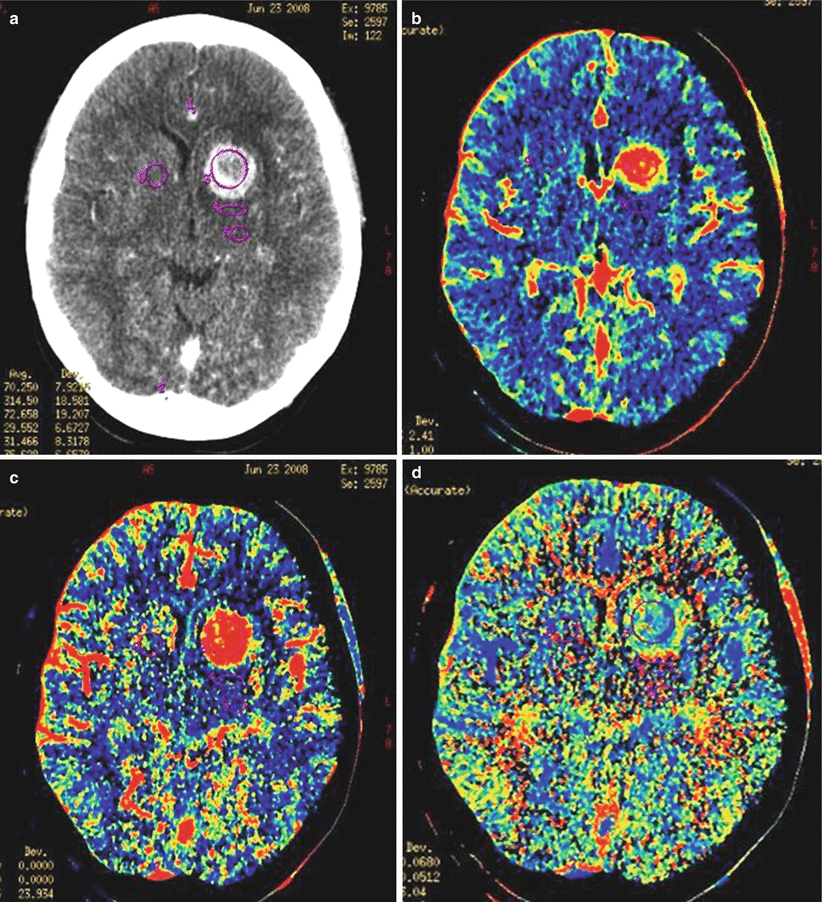
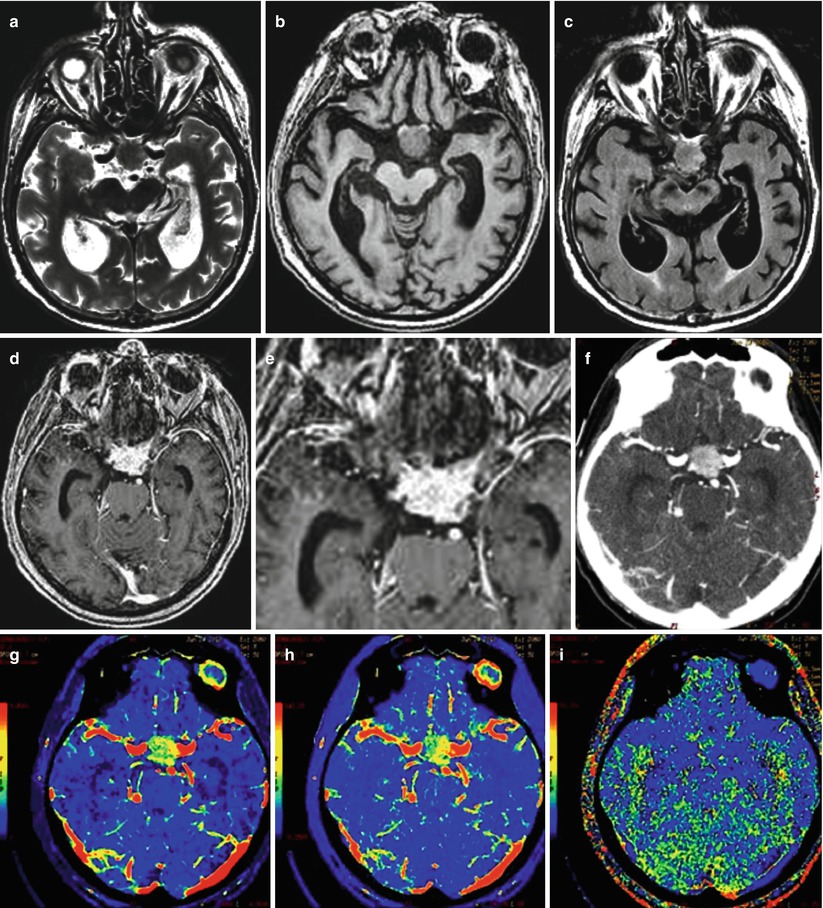
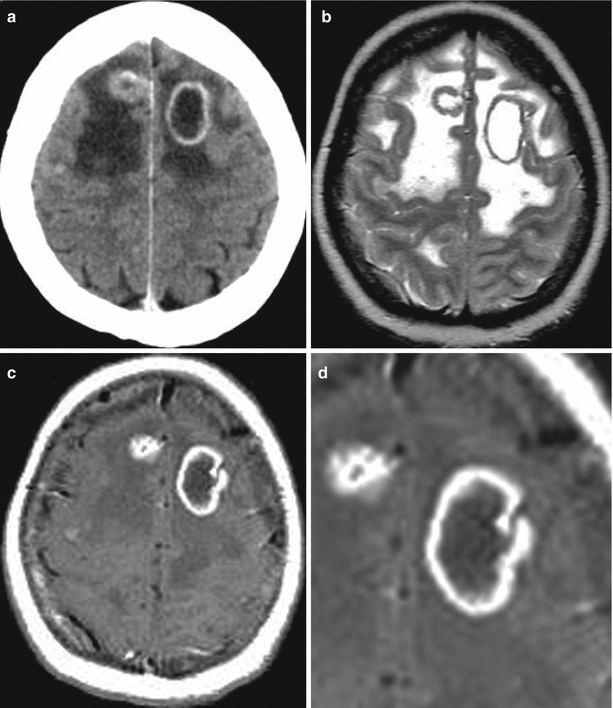
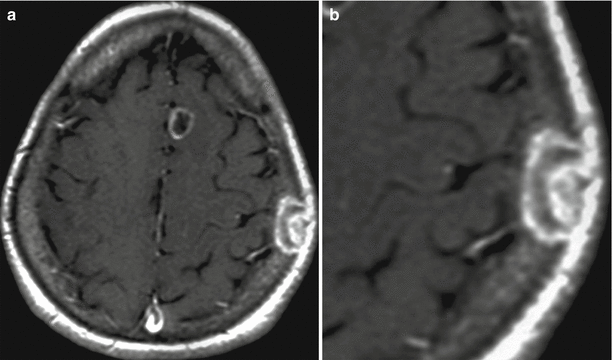
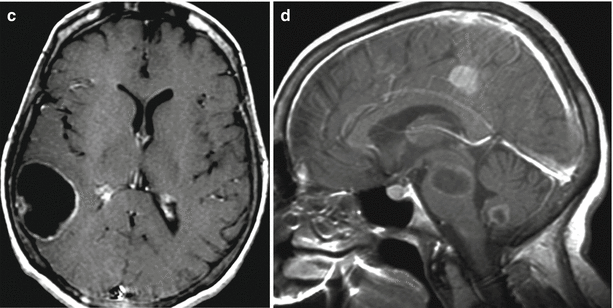
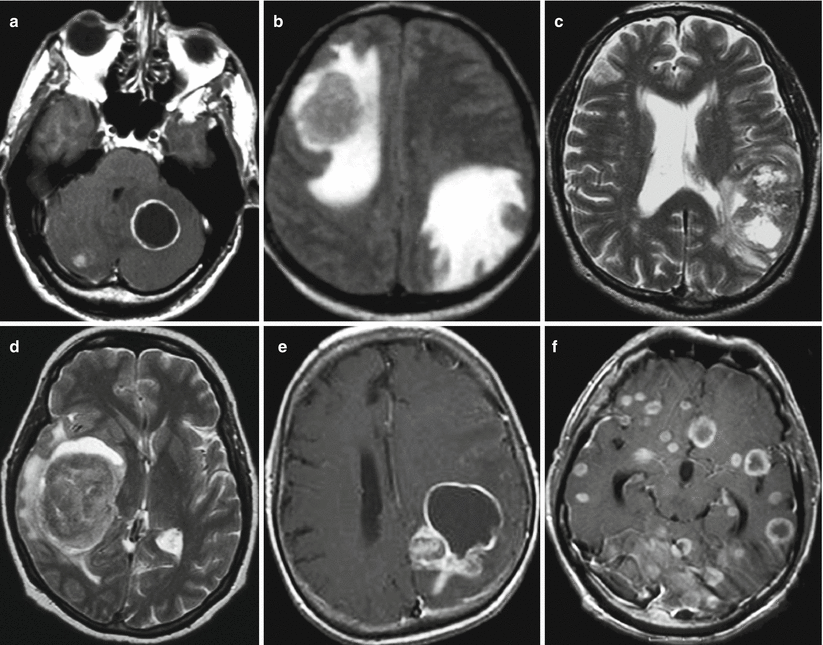
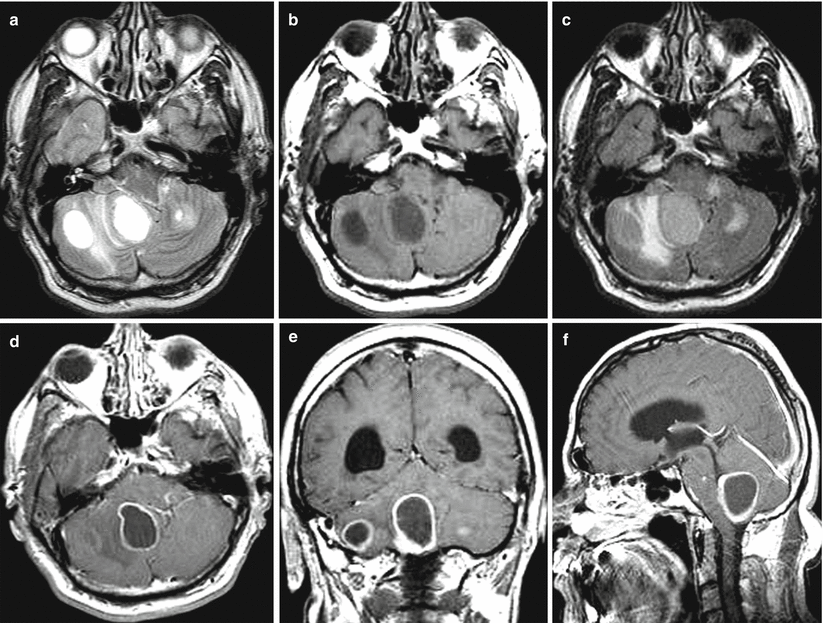
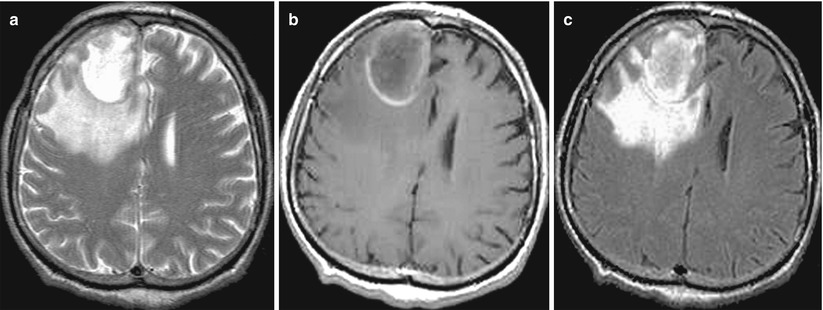
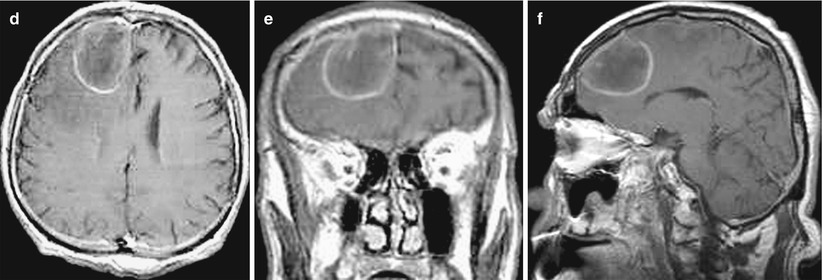
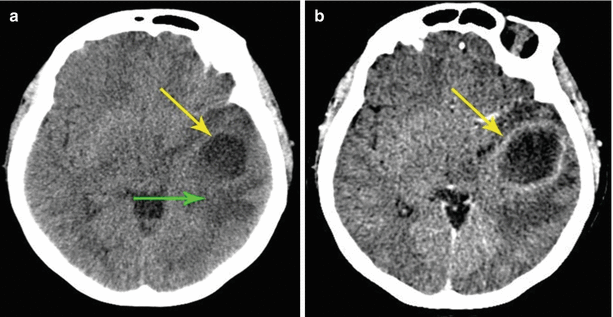
Fig. 12.1
(a, b) A lung cancer metastasis in the brain. Brain CT before (a) and after (b) the administration of the contrast agent. There is a rounded hypodense area (bottom arrow a) in the projection of the left temporal region before the administration of the contrast agent (a) on the background of edema. After the administration of the contrast agent (b), a thin ring-shaped accumulation of the contrast agent in the solid part of the tumor (upper arrows a, b) is identified
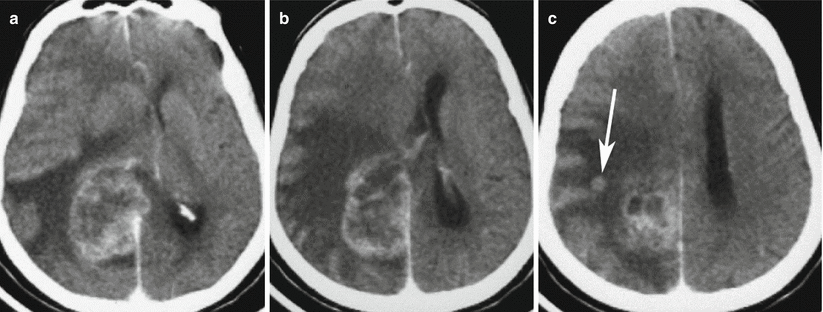
Fig. 12.2
Multiple lung cancer metastases in the brain. On a CT scan after intravenous contrast enhancement (a–c), there is a large lesion with marked, heterogeneous contrast enhancement in the right parietal region and the second lesion (arrow) anteriorly to it (b)
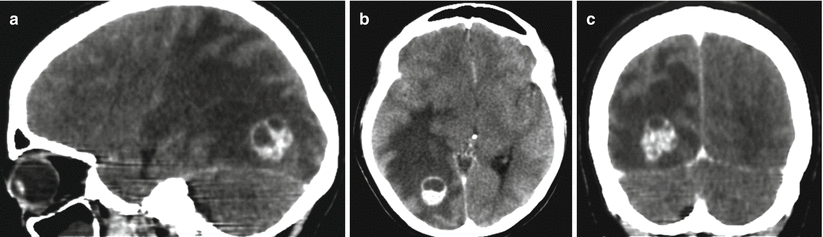
Fig. 12.3
A metastasis of lung cancer in the right occipital region of the brain. On a CT scan after intravenous contrast enhancement (a–c), a small-sized tumor site surrounded by an area of pronounced perifocal edema is visualized
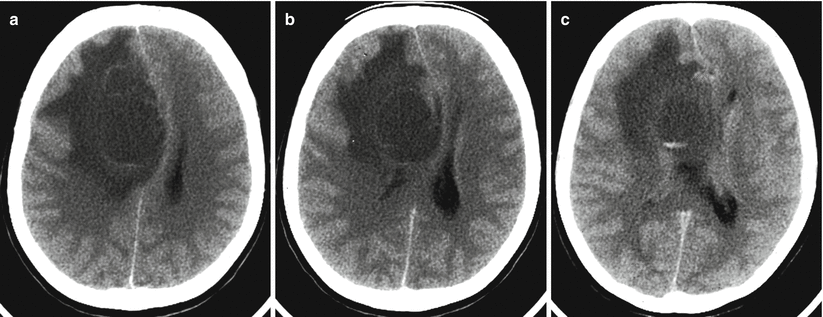
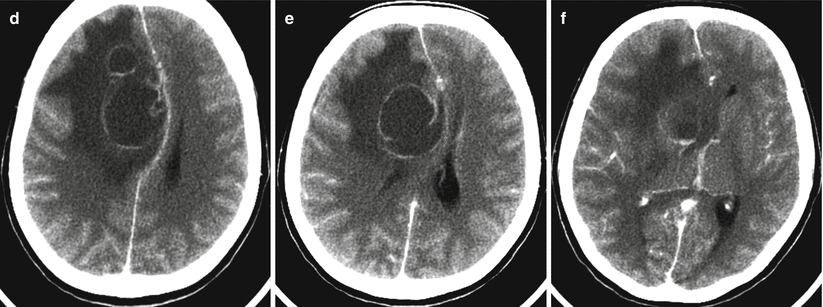
Fig. 12.4
Multiple lung cancer metastases in the brain. CT before (a–c) and after (d–f) intravenous contrast enhancement. There are two tumor sites with contrast enhancement in the form of a thin rim (a, d). Metastases have a centrally located area of necrosis with signs of a pronounced perifocal edema and a midline shift to the left
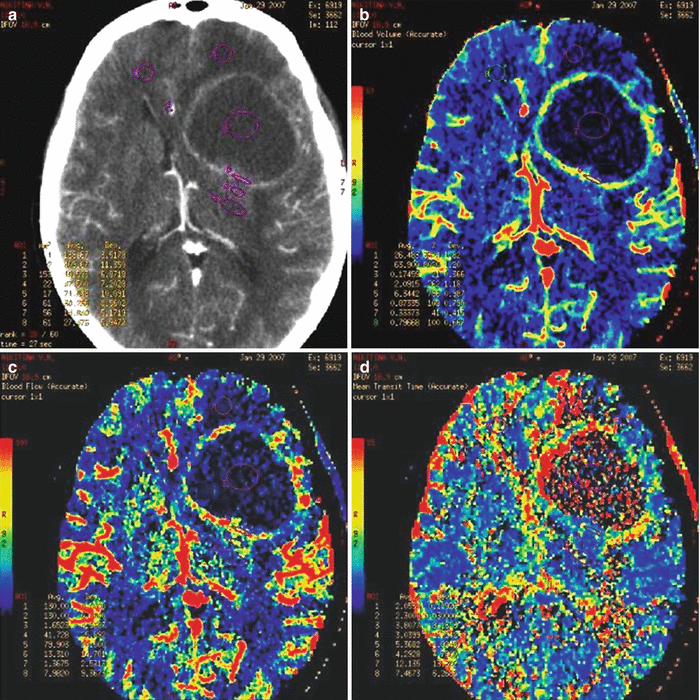
Fig. 12.5
A lung cancer metastasis in the brain. CT perfusion. In the left frontal lobe, there is a space-occupying lesion, predominantly with a cystic structure, accumulating the contrast agent on the periphery (a), CT perfusion shows a marked decrease in perfusion in the cystic component and a pronounced uneven increase in CBV (b), CBF (c), and MTT (d) along the contour of the cavity
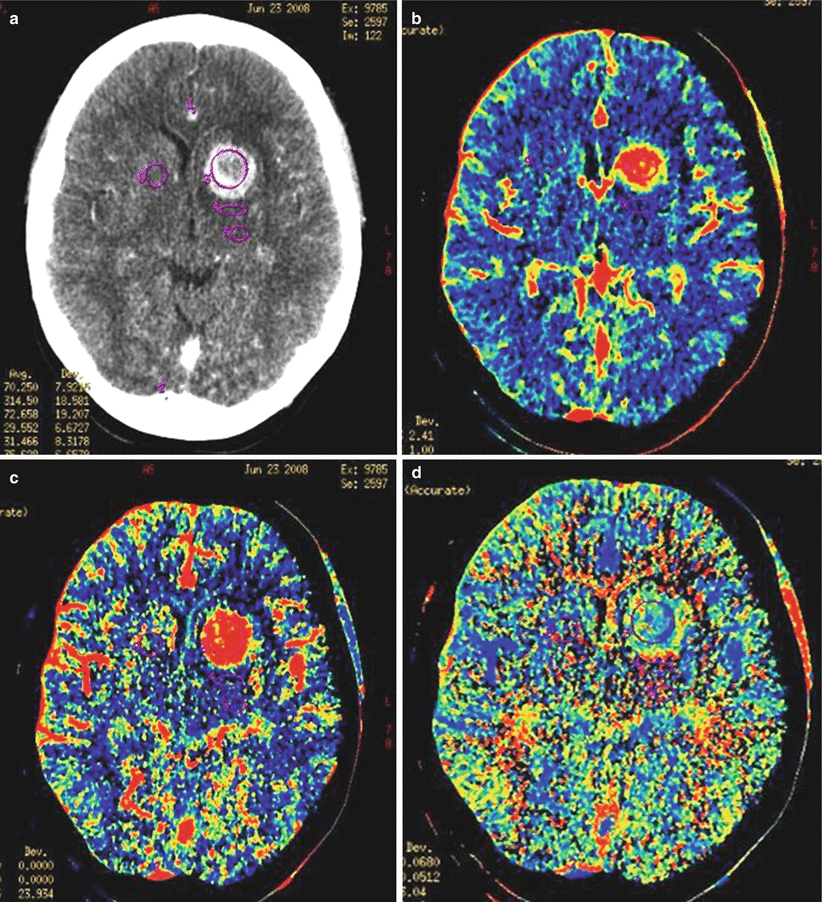
Fig. 12.6
A lung cancer metastasis in the brain. In the subcortical nuclei on the left, there is a richly vascularized space-occupying lesion, rapidly accumulating the contrast agent on CT (a) and characterized by higher perfusion parameters CBV and CBF relative to the intact brain substance (b, c), and MTT values (d) are increased to a lesser extent
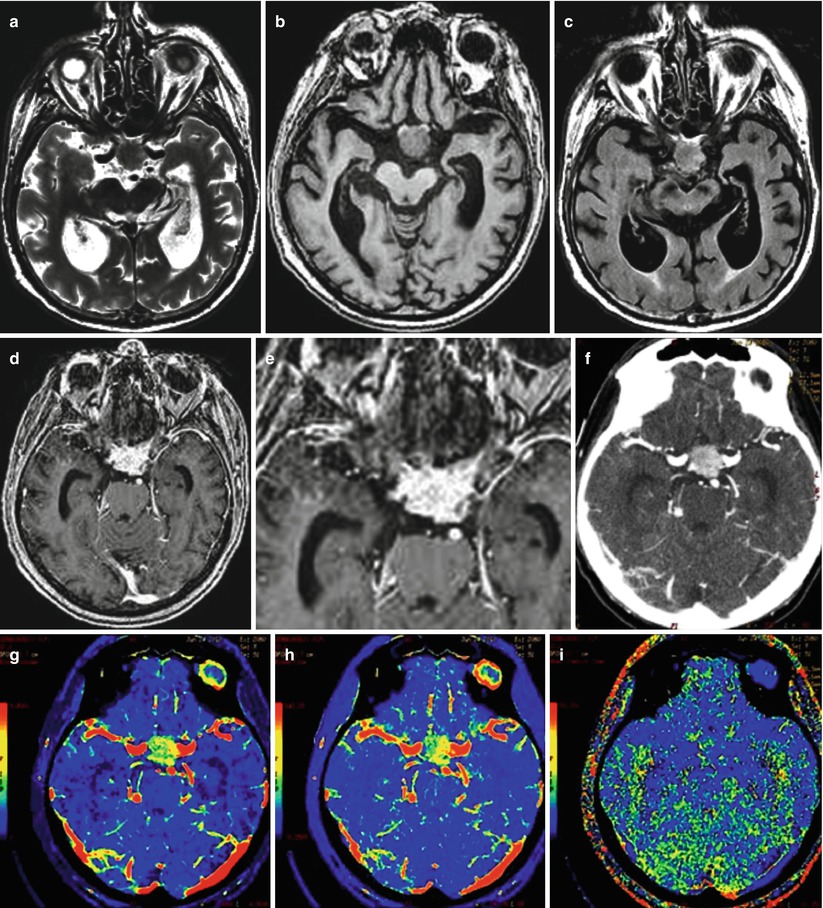
Fig. 12.7
A lung cancer metastasis in the brain. Intra- and suprasellarly, there is a space-occupying lesion with isointense MR signal in T2-weighted (a), T1-WI (b), and T2-FLAIR (c) images that uniformly and intensely accumulates the contrast agent both in MRI (d, e) and CT (f). On CT perfusion maps, there is a marked asymmetrical increase in CBV (g) and CBF (h), while MTT (i) values are slightly increased
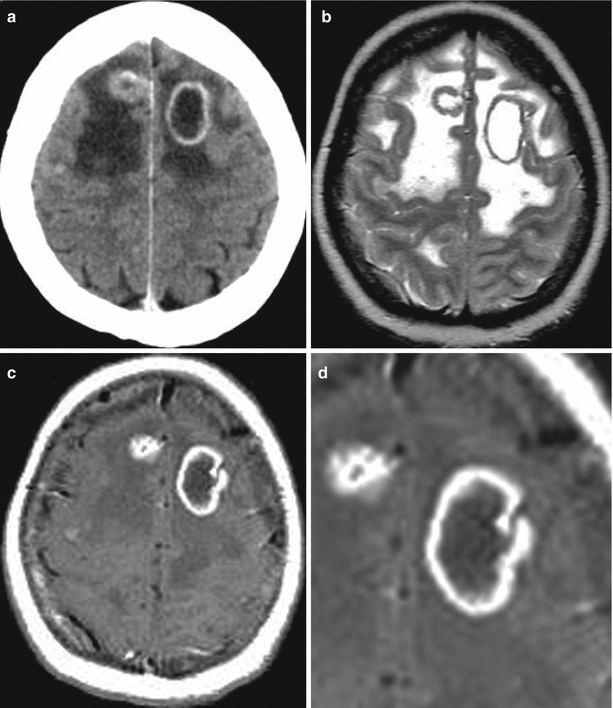
Fig. 12.8
Multiple lung cancer metastases in the brain. In frontal areas, two space-occupying lesions with different diameters are identified. The lesions intensely accumulate the contrast agent both on CT (a) and T1-weighted MRI (c, d). The signal from the central part of the lesions on T2-weighted MRI (b) is increased. The symmetry of the perifocal edema enhancement stands out, despite the differing sizes of tumors
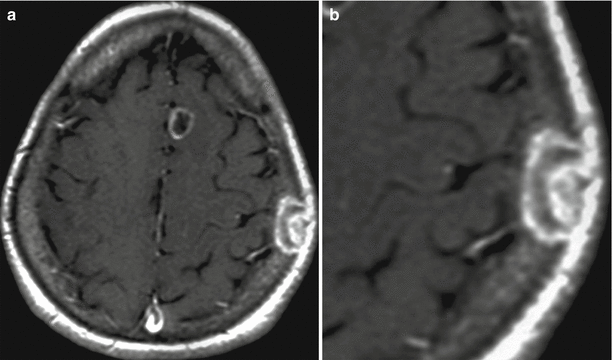
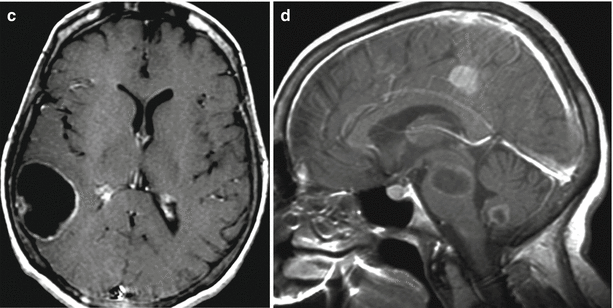
Fig. 12.9
Multiple lung cancer metastases in the brain. On a series of MRI images with contrast enhancement in the axial (a, b, c) and sagittal (d) projections, there are multiple tumor lesions located supra- and subtentorially, with no signs of perifocal edema. In the squama of the frontal bone on the left (a, b), there is a large lesion with uneven contrast enhancement. Metastases in the brainstem and cerebellum (d) are characterized by a cystic structure
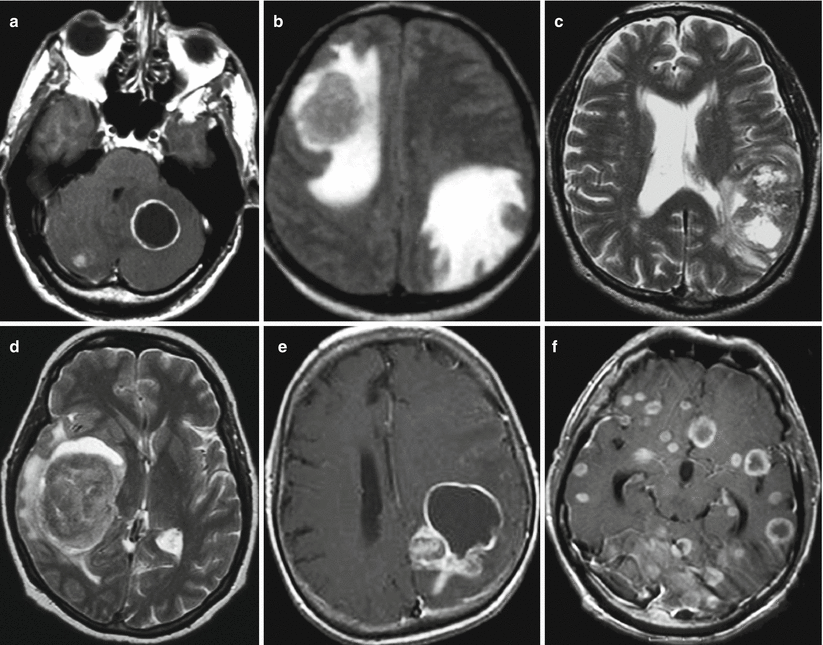
Fig. 12.10
Lung cancer metastases in the brain. Different types of MR manifestations of metastases: (a) cystic subtentorial metastases, (b) multiple solid lesions with a pronounced perifocal edema, (c) a lesion with the mixed structure without a perifocal edema, (d) a lesion with the solid structure with a minimum perifocal edema, (e) a lesion with the mixed structure, and (f) multiple lesions with necrosis in the center
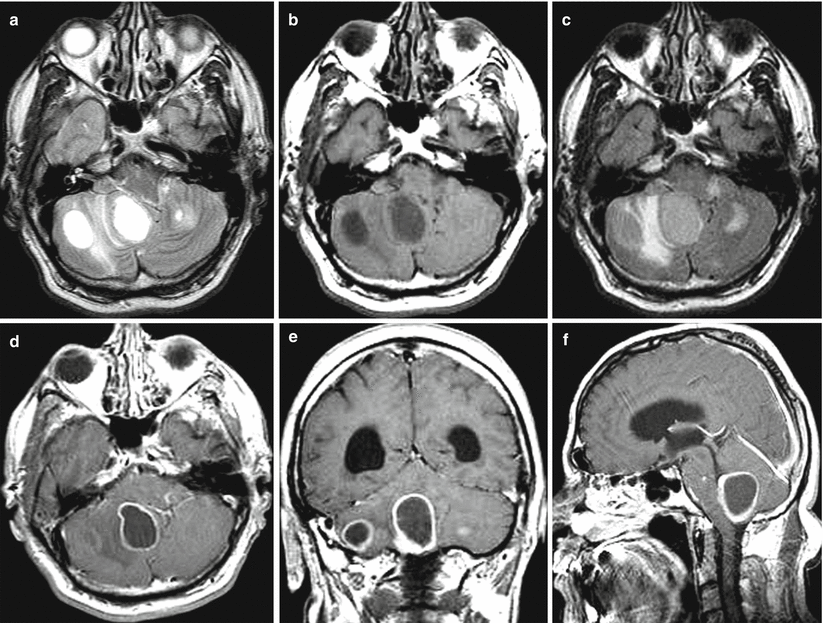
Fig. 12.11
Multiple lung cancer metastases in the brain. In both cerebellar hemispheres, there are solid cystic lesions with a moderate perifocal edema (a–c). After the administration of the contrast agent, its intense accumulation is noted mainly on the periphery of the lesions (d–f). On frontal sections (e) in the area of the cerebellar vermis, along the lower contour of the metastasis, a solid tumor fragment is well visualized
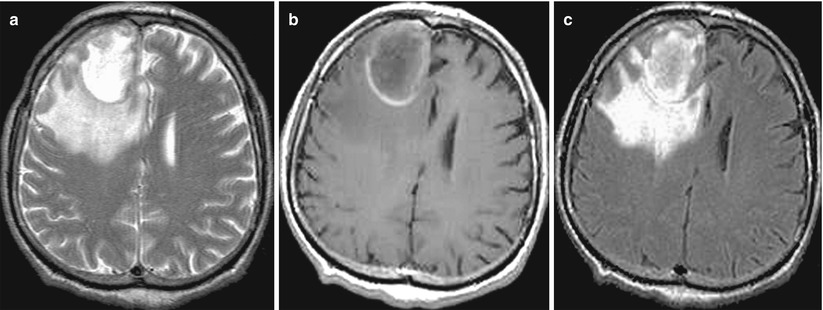
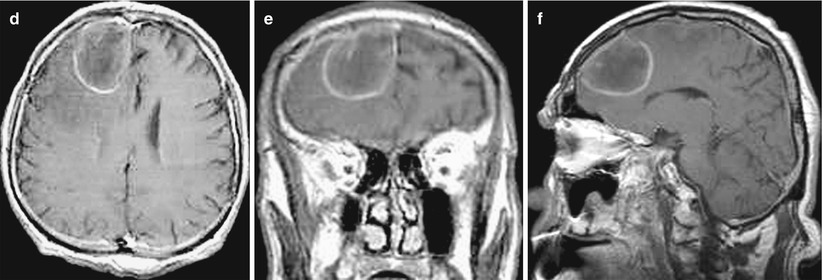
Fig. 12.12
A lung cancer metastasis in the brain. In the right frontal area, there is a solitary cystic lesion with a pronounced perifocal edema (a, b, c) with a hemorrhage area along the contour (b) and a hyperintense signal in T1-weighted MRI. No apparent signs of an increased T1-weighted signal after intravenous CA injection on a background of hemorrhagic changes were identified (d–f)
