Fig. 62.1
MR sequences used during core needle biopsy. Following localizing images (a), axial and sagittal, pre- (b) and postcontrast sequences (c, d) are obtained to identify the target (long arrows). After placement of the obturator, axial and sagittal sequences are obtained to confirm proper depth and location prior to the biopsy (short arrows) (e, f). Further images are performed after the biopsy to confirm the deployment and location of the marker clip (thick arrows) (g, h)
Radiologic-Histologic Correlation
Malignancy rates ranging from 16 to 37 % have been reported in the MRI-guided core biopsy literature [4, 7, 10–12]. Radiologists who perform biopsies should review images to determine whether the target lesion was adequately sampled. For high-risk and discordant lesions, surgical excision is recommended. Reported imaging-histologic discordance rate is approximately 7–9 % [4, 13]. Benign concordant histologic results may warrant short-term (6-month) follow-up MRI to confirm stability, depending on confidence of the radiologist regarding adequacy of tissue sampling. Higher rates of imaging-histologic discordance and underestimation of atypical ductal hyperplasia (ADH) and ductal carcinoma in situ (DCIS) are reported with MRI-guided biopsies than with mammography stereotactic-guided biopsies [13–15].
MRI may depict additional findings occult by mammography and sonography and may result in follow-up and biopsy recommendations as well as mastectomies. However, clinical judgment should be exercised, and the planned management should not be changed based on MRI findings alone without initial biopsy confirmation. MRI-detected, clinically occult disease in a patient with newly diagnosed breast cancer is frequent (Fig. 62.2). Multifocality, the finding of a separate site of cancer within the same quadrant, has been reported in 9–28 % of women. Multicentric tumor in different quadrants or beyond 5 cm from the index tumor occurs in approximately 7–10 % of cases [16–18]. Additional research is needed with regard to the impact of MRI on the outcome of breast cancer patients [2].
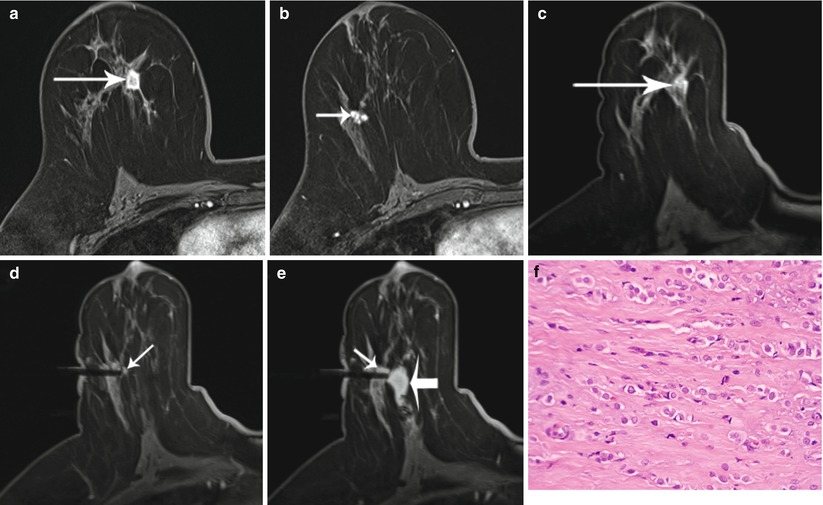
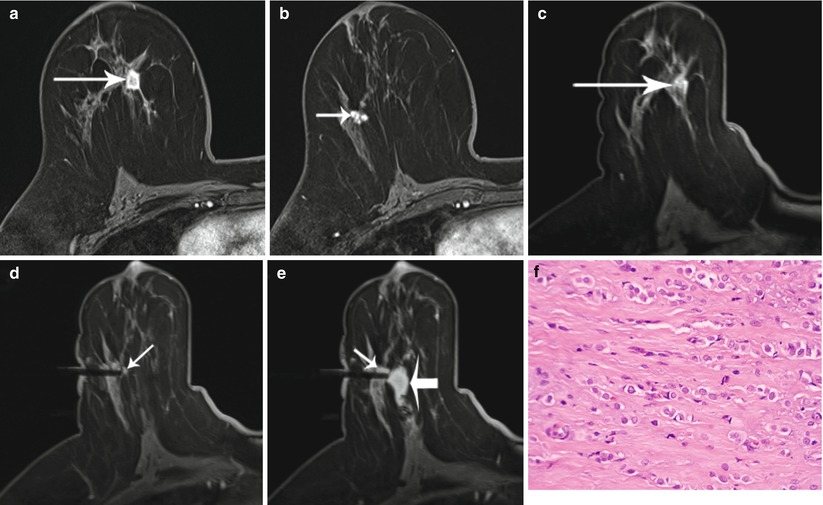
Fig. 62.2
A 51-year-old woman with recently diagnosed right breast 1.5 cm invasive lobular cancer. Breast MRI performed for extent of disease showing the known cancer at approximately 12 o’clock position (long arrow) (a) as well as a second suspicious, irregular, ill-defined enhancing mass measuring 1.1 cm in the lower outer breast (short arrow) (b). The second mass was occult by mammography and sonography, and an MRI-guided biopsy was recommended. Images obtained during MR-guided biopsy show again the index cancer (long arrow) within the slightly compressed breast (c) as well as the introducer at the targeted second mass (short arrow) (d). Partial imaging of the breast is performed at the time of biopsy to decrease image acquisition time. Corresponding post-biopsy MR image (e) shows less visibility of the targeted mass (short arrow) and area of hematoma (thick arrow) medial to the mass. All MR images above were performed in axial plane after contrast administration using a T1-weighted fat-saturated 3D spoiled gradient-echo sequence. Histopathology of core biopsy (f) revealed invasive carcinoma with predominantly lobular features, moderately differentiated (modified Bloom-Richardson grade II/III), ER/PR positive, and HER2/neu negative (hematoxylin-eosin stain; original magnification ×200). Subsequent mastectomy confirmed presence of multicentric invasive cancer
MRI-Guided Breast Wire Localization
When amenable, performing a percutaneous needle biopsy is advantageous compared to performing a wire-localized (WL) biopsy, as it may save the patient from unnecessary surgery and reduce cost [7]. Needle localization is performed in patients whose extent of disease is not considered evident by conventional imaging modalities and when the patient prefers surgical removal or refuses core biopsy.
Equipment and Technique
The positioning and localization procedure for needle localization is the same as that for needle biopsy. Once the lesion is localized, an MR-compatible guide needle is introduced to the appropriate depth. After imaging confirms appropriate location and depth, a guide wire is deployed through the needle. The needle is similar in design to the Kopans needle. The MR-compatible hook wire is somewhat softer than non-MR-compatible localization wires; thus there is a propensity to break during surgery [19], and deployment in hard fibrous tissue may be occasionally difficult. After the localization procedure, a two-view mammogram is usually obtained so that the surgeon can see the location of the wire and relationship to the nipple and breast tissue.
Radiologic-Histologic Correlation
MR imaging of an excised breast specimen is, at this time, not useful for lesion detection, since current methods are predicated on the wash-in/wash-out characteristics of contrast agent. Gross examination and specimen radiography do not identify most of the malignancies in MRI-localized procedures. For that reason, the only reliable method to identify all malignant lesions in MRI-guided excisions is to examine all of the tissue microscopically, and optimal pathology processing requires microscopic examination of the entire specimen [20].
MRI-Guided BCS
MRI Use in the Newly Diagnosed Breast Cancer Patient
Contrast-enhanced breast MRI is primarily used for three groups of patients. Screening with MRI may be used for women at increased risk for breast cancer, especially those with a strong family history, a known germline mutation, or a personal history of prior breast cancer [21–23]. Second, MRI can also be useful in the evaluation of patients when the findings of other imaging modalities are inconclusive or equivocal [24]. Finally, breast MRI may be performed after a diagnosis of breast cancer to evaluate for disease extent and occult contralateral breast tumors [25–27].
Accurate delineation of the extent of the malignant lesion and possible multifocality and multicentricity is an essential part of local breast cancer staging, especially when a breast-conserving surgical approach is preferred. While limited in general screening applications by moderate specificity, the excellent sensitivity of the MRI for invasive breast cancer has permitted more precise definition of extent of disease, tumor boundaries, and margins of target lesions. It is being increasingly evaluated for its role in the preoperative local staging of breast cancer [28], although to date, MRI’s ability to reduce re-excisions, lower local recurrence rates, or improve survival has not been shown.
Evaluation of Extent of Disease
Women with newly diagnosed breast cancer are in general offered breast-conserving therapy or mastectomy for early-stage breast cancer that is considered unicentric. Mastectomy and BCS, also known as breast conserving surgery (BCS) offer equivalent survival [29, 30]; however, BCS carries a slightly increased risk of local recurrence which in the modern era is approximately 5–7 %. With more intensive breast imaging and more sensitive modalities, additional disease will be identified around 10–30 % of the time. This disease is treated by a combination of radiation therapy and adjuvant drug therapy. The rate of multifocality and multicentricity in patients initially diagnosed with unicentric disease varies widely from approximately 10 to 50 % [28, 31]. Mammography and ultrasound are often not sensitive enough to detect these additional lesions [32]. Studies have shown that MRI in women with newly diagnosed breast cancers identified additional otherwise undetected synchronous tumor foci in about 10–30 % of patients [17, 28, 31]. Schelfout et al. [32] prospectively studied 204 women and found that of 170 breast cancer patients, MRI detected 96 % of pathologically proven multifocal disease and 95 % of multicentric disease, whereas mammography depicted 37 and 18 %, respectively, and US depicted 41 and 9 %, respectively. Furthermore, findings of more extensive disease and unsuspected multiple breast cancer foci identified on MRI only changed the therapeutic approach correctly in 30 % of breast cancer patients, concluding that preoperative MRI is an important adjunct to conventional imaging in the locoregional staging of breast cancer and a useful tool in treatment planning. In a retrospective analysis of 160 patients with operable breast cancer Braun et al. [33] showed that preoperative MRI diagnosed additional in situ or invasive cancers or a more widespread tumor extent in 30 cases (18.75 %), which went undetected by conventional means. In a meta-analysis by Houssami et al. [28], a total of 2,610 patients published across various retrospective reports found that MRI detected additional foci of disease 16 % of the time, which led to a more extensive lumpectomy 3–15 % of the time, and a conversion to mastectomy 3–33 % of the time. Hence, preoperative breast MRI does change surgical management in a considerable number of patients. The benefit of the higher sensitivity of MR must be weighed against the false-positive findings, increase in number of biopsies, delay in surgery, and increase in mastectomy rates.
Surgical Planning with Preoperative MRI for Lumpectomy
Beyond purported improvement in the selection process for breast-conserving surgery by preoperatively identifying multifocal and multicentric disease, MRI may be considered useful in helping to delineate the extent of (nonpalpable) disease. The benefit of higher sensitivity would be a resulting decrease in the number of surgical procedures required to obtain negative BCS margins and possibly a decrease in local recurrence. These anticipated benefits have not been clearly shown to date in various retrospective and one randomized study.
To appreciate the role of MRI in the surgical planning of BCS, understanding the technique of lumpectomy for a mass is critical. The breast as an anatomic structure does not provide much guidance and orientation. WL under mammogram, US, or MRI guidance into the nonpalpable mass facilitates intraoperative identification of the lesion. An understanding of the three-dimensional relationship between the skin entry site, the shaft of the wire, and the target lesion is critical in obtaining complete resection of the tumor. After WL is performed for the nonpalpable lesion, the skin incision is made close to the wire in a cosmetically acceptable fashion. A skin flap is elevated toward the wire and the wire is exposed. The wire trajectory toward the lesion is palpated, and the distal end is anchored so that it does not get dislodged. Dissection is carried down parallel to, but 1–2 cm distant from, the wire, dissecting around and toward the tip. The dissection is terminated by cutting well past the tip of the wire. The specimen is removed and the margins are marked for orientation either by suture or ink. The specimen with the wire is submitted for radiologic confirmation that the resection encompassed the intact wire, clip, mass, calcifications, and a rim of perceived normal surrounding tissue. The cavity is palpated for any abnormality, hemostasis is obtained, and the incision is closed.
Despite improvements in surgical technique and imaging, the need for re-excision to achieve complete tumor resection in the United States is 20–40 % [34–42]. The negative impacts of re-excision include delay in completion of therapy, increase in infection rates, increase in cost, and possible negative psychological impact on the patient [42]. Additionally, re-excisions can result in diminished aesthetic outcomes [43–45]. The principle of clear margins is imperative because presence of close or positive margins is associated with increased locoregional recurrence and failure to maintain local control is associated with a decrease in long-term cancer-specific survival [39, 46, 47].
Preoperative MRI has been evaluated for clinical efficacy in reducing the re-excision rates and ultimately reducing local recurrence rates. Retrospective data and a single randomized trial have not confirmed these suggested benefits. In the current era of multimodality therapy, local recurrence rates after breast-conserving therapy are low at approximately 5–7 % with modern radiation/adjuvant therapy and do not match the rate of identification of additional foci of disease [48]. This finding suggests that radiation with adjuvant therapy will usually control the additional tumor burden not detected on ultrasound or mammography. Thus despite the presence of additional disease outside of standard field of surgical resection, including microscopic tumor burden, adequate treatment is provided by a combination of whole-breast irradiation along with appropriate adjuvant chemotherapy, endocrine therapy, and/or targeted therapy. Elegant studies performed in the 1980s by Holland et al. [49], on serial sectioning of mastectomy specimens in women with presumed unicentric tumors T2 or smaller identified additional disease 43 % of the time greater than 2 cm from the reference tumor, again suggesting this disease has always existed and was treated with adjuvant therapy.
The University of Pennsylvania group’s retrospective review of women who underwent breast MRI versus no breast MRI in the preoperative setting followed by breast-conserving therapy showed no statistical difference in the local recurrence rates between the two groups (3 % vs. 4 %, p = 0.51) at 4.6 years of follow-up. A similar retrospective review from the University of Toronto group with 8 years of follow-up also did not show a statistical difference in local recurrence with 1.8 % in the MRI group versus 2.5 % in the no MRI group (p = 0.67) [50].
Breast MRI has however been associated with an increase in mastectomy rates, including prophylactic contralateral mastectomy [51], delay in completion of local therapy, and increase in the number of additional biopsies in several studies [2, 28, 52]. A review of the data from Fox Chase Cancer Center comparing women who underwent breast MRI to the group that did not, revealed no statistical difference in positive margin rates associated with lumpectomy [53]. In a retrospective analysis, McGhan et al. [54] compared reoperation rates in patients who underwent MRI and those who received conventional management. This group found the use of MRI offered no improvement in reoperation rates.
The criticisms for the above mentioned recent publications include the retrospective nature and selection bias. The COMICE trial (Comparative Effectiveness of MR Imaging in Breast Cancer) is the only randomized trial performed to date and revealed re-excision rates of 19 % in each arm and equivalent local recurrence rates albeit with relatively short-term follow-up [52]. This trial has been criticized for the large number of patients who underwent mastectomy based on MRI finding without pathologic confirmation, the high number of postmenopausal woman included, the high number of low volume breast surgeons in the trial, and the remarkably low re-excision rates. These criticisms along with the varying equipment, technique, sequencing, and interpretation decrease potential impact of MRI in this trial. Despite these pitfalls, the trial has provided valuable information on the use of breast MRI in the setting of extent of disease evaluation.
Our own group at the Dana-Farber/Brigham and Women’s Cancer Center retrospectively reviewed women diagnosed with breast cancer between 1/2005 and 12/2008 who received breast-conserving surgical treatment for breast cancer at our institutions. We identified 622 patients, 133 received preoperative MRI and 489 did not. Our re-excision rates were 40 %, double that of what was seen in the COMICE trial and in line with the retrospective data published in North America. The preliminary analysis of 448 patients without MRI and 105 patients with MRI shows the following: of the no MRI group, 59.82 % required only one surgery to achieve definitive surgical treatment; of the MRI group, 47.62 % required only one surgery to achieve definitive surgical treatment. A Fisher exact test indicates a statistically significant difference between the two groups with p = 0.0280. This preliminary analysis (Gombos EC, Sippo DA, Weiler N, Birdwell RL, unpublished data) does not adjust for differences between the two groups, such as tumor grade, and includes a mix of DCIS only and invasive cancer.
While MRI is exceptionally sensitive, the accuracy of MRI as defined by pathologic confirmation is extremely variable [55]. Currently the data are not sufficient to support or dismiss the use of MRI for extent of disease evaluation; however, as MRI techniques improve, new trials are needed to better define the role of preoperative MRI and to possibly identify a subgroup of patients who may benefit from the enhanced imaging modality.
Surgical Navigation and Intraoperative MRI for Lumpectomy
In addition to the potential role of MRI for surgical planning and postoperative margin status assessment (Fig. 62.3), imaging techniques may be extended to the intraoperative setting. The goal of intraoperative imaging is to identify bulk residual disease which can be removed with shave margins and potentially spare patients a second operation for re-excision. Considerations for intraoperative MRI are image interpretation in the presence of acute surgical changes as well as the challenge of imaging in the supine position. These issues have been evaluated by several groups who utilized supine imaging positioning to approximate the surgical position. This approach is an alternative to standard prone imaging, where the breast is pendulous. Although prone positioning is essential for identifying small lesions near the chest wall and in the periphery of the breast, supine positioning facilitates registration of the images with the patient in the surgical position. Few studies explored the attending challenges of this approach.
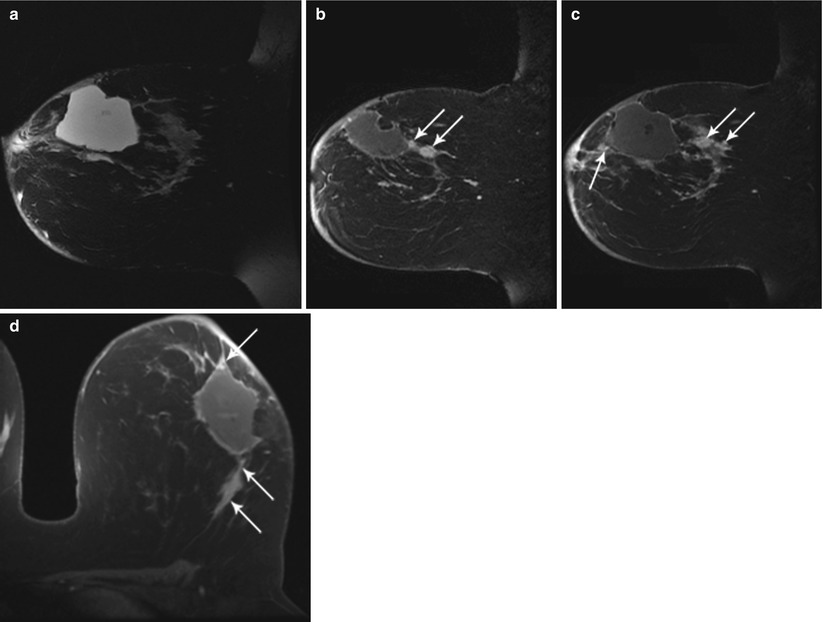
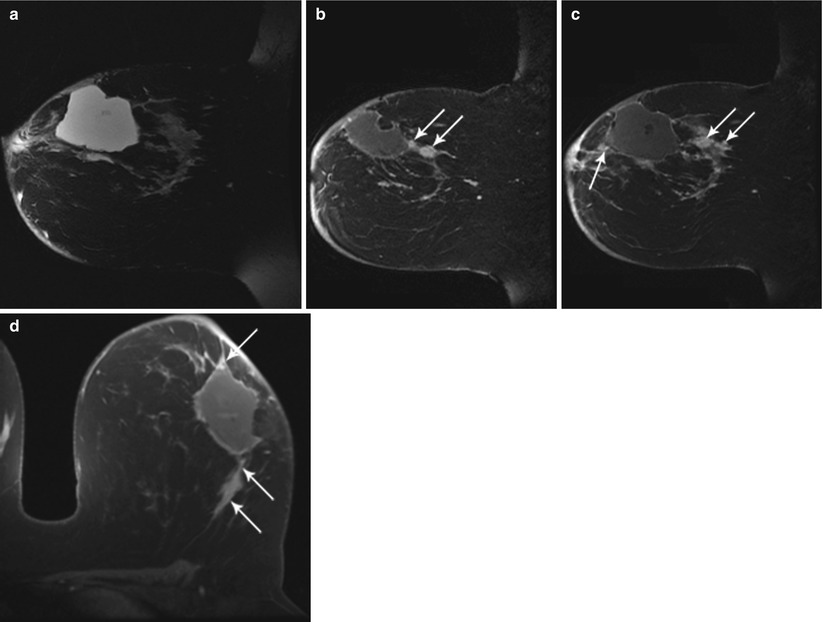
Fig. 62.3
MR helps to detect evidence of bulk residual disease. A 27-year-old woman; palpable abnormality led to a left lumpectomy with a diagnosis of invasive cancer and DCIS with all but the lateral margins positive. (a) MR demonstrates high signal on T2 at the seroma site. (b, c) Dynamic sagittal images show irregular enhancing masses at the anterior and supero-posterior margins. (d) Delayed axial image shows irregular enhancement at the anterior and posterior margins. Pathology of mastectomy confirmed four foci (1.0, 1.0, 0.4, and 0.2 cm) of residual invasive ductal carcinoma, poorly differentiated (modified Bloom-Richardson grade III/III), and DCIS. Lymphovascular invasion was present. Invasive carcinoma was at the posterior resection margin and <0.1 cm from the anterior/superior margins
Yamashiro et al. [56] reported on a supine MRI marking technique for planning of lumpectomy to modify the first resection line, as determined by US. Tozaki et al. [57] explored a single breathhold MRI technique during supine positioning in 27 patients. Two patients had positive margins. Sakakibara et al. [58] reported that supine preoperative MRI in conjunction with projection techniques to match the surgical position to the imaging position reduced the rate of positive margins while decreasing the size of the surgical specimen. Abe et al. [59] performed supine MRI marking technique with a drape-type thermoplastic mesh sheet and fiducials to plan the surgical resection margins. The sheet was heated and stretched to conform to the breast and torso. Upon cooling, the sheet becomes rigid. MRI-visible fiducials were placed on the sheet prior to imaging. The physical fiducials were used to approximate the location of the underlying tumor based on the MR images, and the dissection line was inked on the skin through the holes in the mesh. During surgery, the plastic sheet was again placed on the breast to return the breast to imaging position and to reaffirm the surgical approach (Fig. 62.4). The shell was removed prior to resection. Thirty-five patients were treated. Among the 24 patients who did not undergo preoperative chemotherapy, 5 (20.8 %) had positive margins, attributed to MRI underestimation of the tumor and hence incorrect margin indication for lumpectomy. In addition, one patient underwent an unnecessarily large lumpectomy based on overestimation (false-positive findings) of lesion on MRI. The remainder of cases underwent surgery with correctly planned surgical margins.
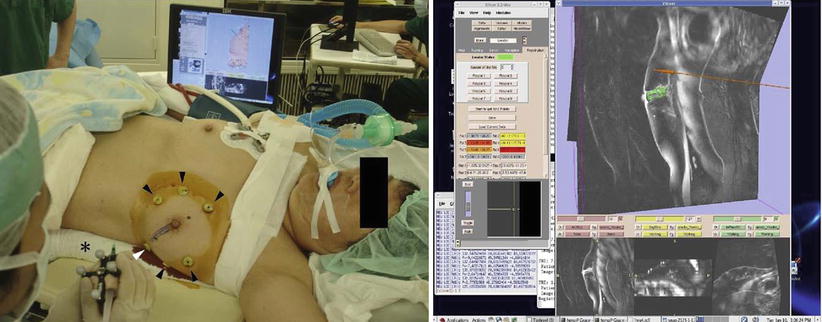
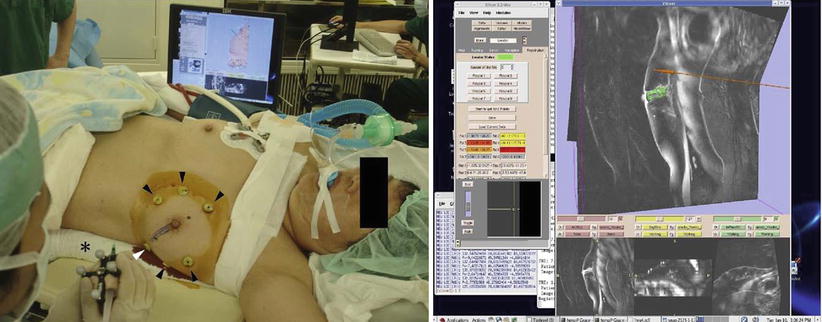
Fig. 62.4
Left, percutaneous intramammary injection of dye in the syringe to make a dissection line of the tumor with the navigation of 3D Slicer. Arrowheads, skin markers; asterisk, a syringe attached with infrared markers; right, virtual MR images on the monitor of 3D Slicer reflecting the same slice as the puncture line. A breast tumor is indicated in green; a needle and a puncture line are indicated in red (Reproduced from Tomikawa et al. [60])
Alderliesten et al. [61] acquired supine preoperative MR images to be used during surgery for navigation to localize breast cancer in 21 patients. During navigation, the MR image that corresponds to the location and orientation of a tracked probe is displayed. Reproducibility of patient positioning, deformation caused by the instrument, and movement due to breathing were identified as issues.
The use of MRI scanners in the operating room has been shown to benefit tumor localization, refinement of surgical approach, and detection of residual lesion in the field of neurosurgery [62]. In the application to breast surgery, to date, three intraoperative MRI-guided lumpectomy studies have been performed. Gould et al. [63] used a 0.5 T vertically open scanner and reported close agreement between maximum dimensions of MRI localization of benign breast lesions (n = 10) and histopathologic examination (p > 0.17). All post-procedure scans demonstrated complete resection.
At Brigham and Women’s Hospital, Hirose et al. [64], using the same type of scanner, reported on MRI-guided lumpectomy for patients with an invasive breast cancer diagnosed by core needle biopsy. All tumors (n = 20) were localized with MRI.
Using final permanent pathology as a gold standard, margins determined by post-resection contrast-enhanced intraoperative MR were compared to margins determined by gross pathology. Nine patients (45 %) appropriately underwent intraoperative shave margins, and eight patients were spared a second surgery. In five patients, this re-excision was prompted by the gross pathology only (false-negative intraoperative MRI, true-positive gross pathology) and in four patients by the intraoperative MRI image only (true-positive MRI, false-negative gross pathology). In one of the four true-positive MRI patients, the gross pathology and permanent pathology margins were negative, and the MRI-guided shave margin contained a satellite invasive ductal focus. In a second patient, the final pathologic shave margins were positive for DCIS; this patient underwent completion mastectomy, which revealed extensive, mammographically occult DCIS throughout the breast specimen and no residual invasive cancer. In the remaining two patients whose shave margins were prompted by the MR image, and in whom the permanent pathology margins were also read as positive, the shave margins were histologically negative (Unpublished data collected at Brigham and Women’s Hospital, Boston, MA).
Although addition of MRI guidance obviated the need for a second operation in 4 (20 %) cases, the procedure was suspended. The deficit in image quality, attending imaging at 0.5 T, which could be address with an invasive receive coil, made reliable detection of residual tumor a challenge. Since fat saturation is difficult at low field, exotic and time-consuming imaging techniques were employed. Dynamic imaging with fat saturation was not possible. For these reasons, Brigham and Women’s Hospital chose to translate the program to a 3 T MRI scanner.
The first patient of this series was operated on in BWH’s AMIGO Suite, an OR with adjoining MRI and PET/CT rooms. The 3 T MRI scanner (Siemens Healthcare, Erlangen, Germany) was integrated such that it can be moved via ceiling tracks into the OR as needed and removed subsequent to image acquisition (IMRIS Inc, Winnipeg, Canada). The surgical workflow broadly consisted of following steps: 1. Anesthetize the patient, 2. Move the MRI over the patient, obtain pre-procedure images and then retract the MRI scanner 3. Excise the tumor, 4. Move the MRI scanner over the patient, image for remnant tumor, and then retract the scanner, 5. Perform shave margin resection. The imaging protocol consisted of Axial VIBE pre-contrast and four post-contrast images, sagittal post-contrast VIBE and T2 edema mapping sequences. The four post-contrast images were obtained 120s, 210s, 300s and 390s after contrast injection. Patients received 0.2 ml/kg of gadolinium (Magnevist, Bayer HealthCare) iv contrast infused at 2 ml/s. Dynamic contrast enhanced MRI was utilized to delineate the extent of the tumor and evaluate remnant tumor immediately after the surgery. Subtracted images (first post-contrast minus the pre-contrast images) were computed on the scanner and provided to the radiologist. Additional tumor maps generated by CADstream (Merge Healthcare, Chicago) were also provided to detect the tumor.
Feasibility of the surgical workflow was successfully demonstrated. The pre-procedure scan showed, as expected, that the breast deforms significantly, particularly compared with the standard diagnostic, prone image, as shown in Fig. 62.5. This finding underscores the importance of preoperative supine MRI, which simulates the surgical position of the breast. The technique needs to accommodate the intra-procedural retraction of the breast in order to be most helpful to the surgeon to map out margins adequately. The DCE-MRI showed significant signal enhancement of the tumor at the first post-contrast image due to the enhanced perfusion of the contrast within the tumor, as seen in Fig. 62.6 (a) and (b). Correspondingly, the CADstream output showed regions of contrast wash-in and washout and can be seen in Fig. 62.6 (c). It was also demonstrated that in an operative field, there is no significant enhancement from bleeding vessels if adequate hemostasis is obtained. T2 edema imaging was also performed to compensate for the reduced perfusion of the contrast within the tumor. Machine learning [65] and time-series [66] based algorithms have also been developed to detect remnant tumor by MRI. In addition, non-rigid deformable registration algorithms with fiducials for accurate orientation of the margins are also being developed to detect remnant tumor [67], as shown in Fig. 62.8. Figure 62.5 illustrates the first procedure.
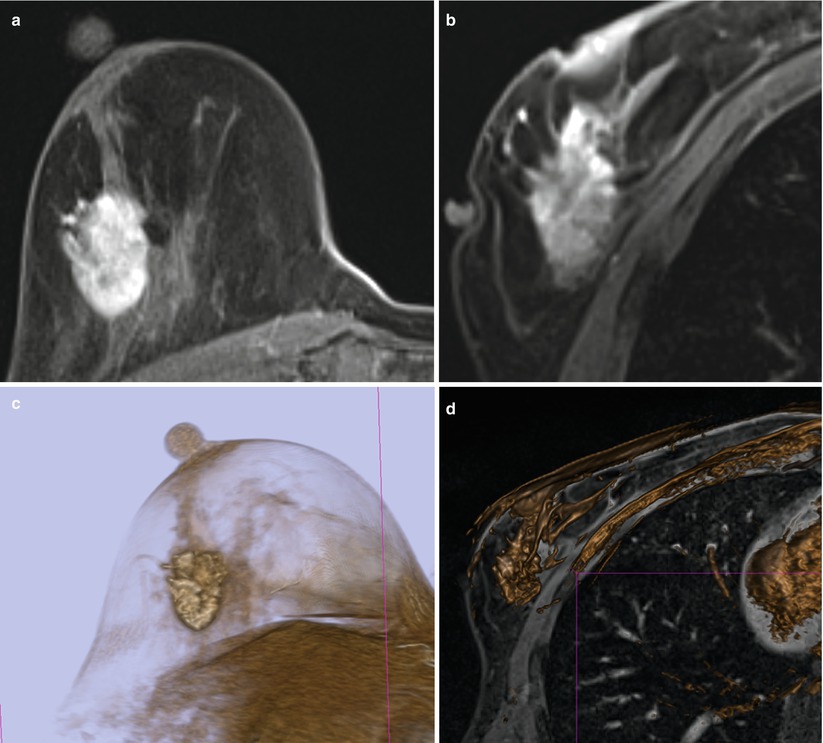
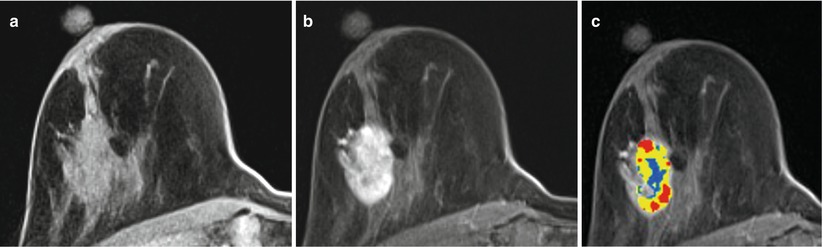
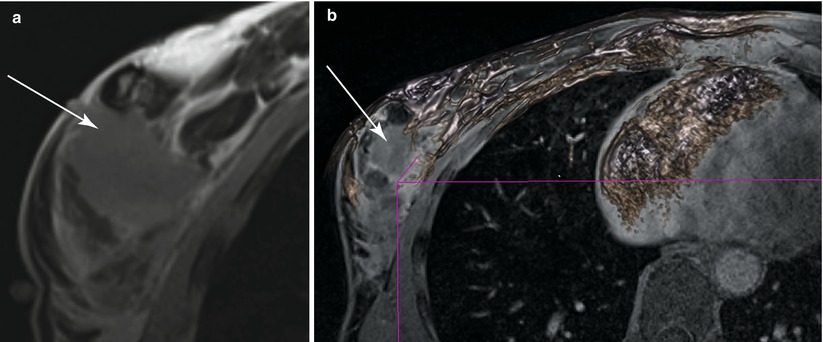
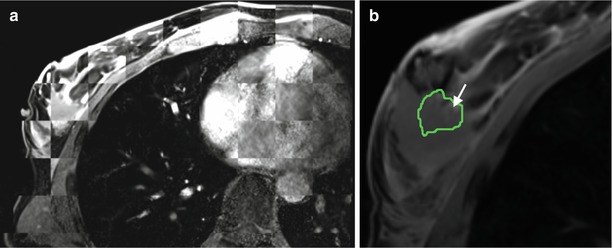
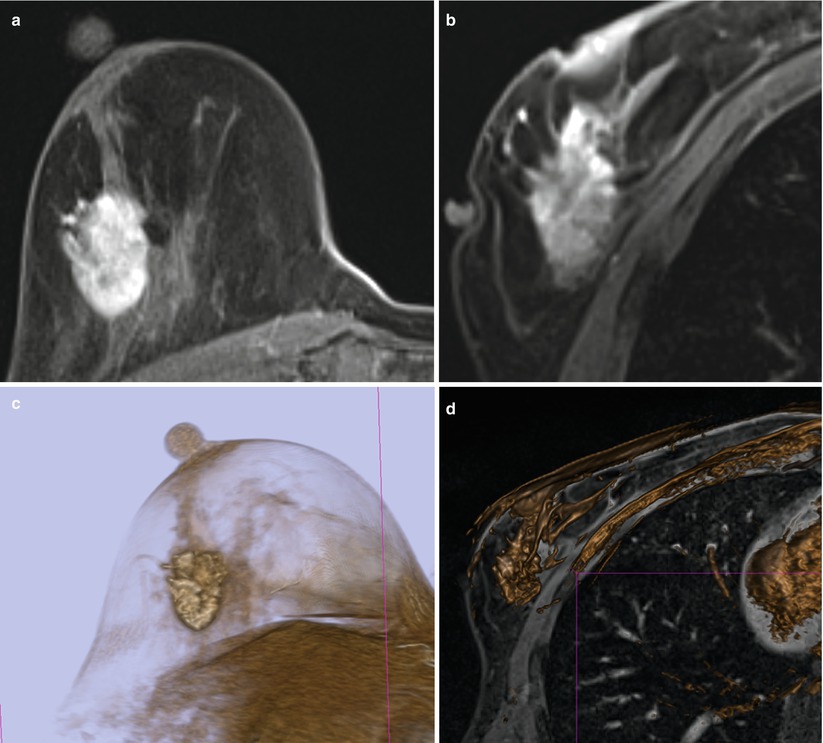
Fig. 62.5
(a) First post-contrast prone diagnostic image (b) First post-contrast supine pre-procedure image (c) Volume rendering of the diagnostic MRI (d) Volume rendering of the pre-procedure MRI
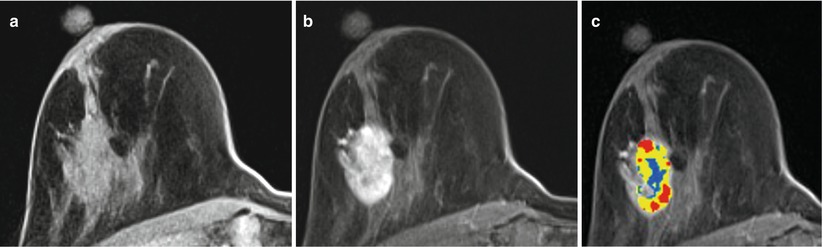
Fig. 62.6
(a) Pre-contrast DCE-MRI (b) First post-contrast DCE-MRI (c) CADstream output. Red, yellow and blue represent voxels with a Type III, II and I curve respectively
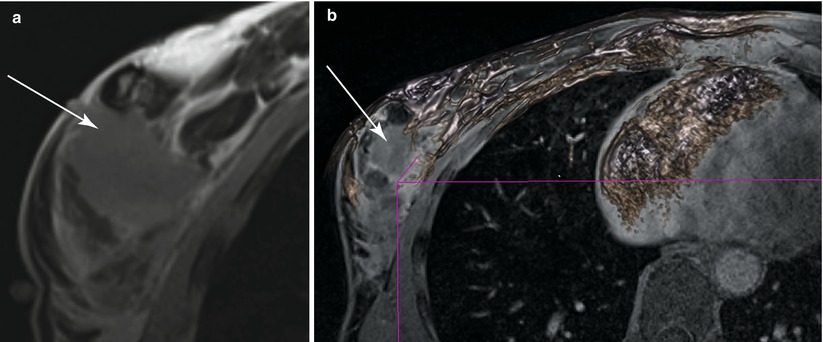
Fig. 62.7
(a) Post-procedural DCE-MRI showing the surgical cavity (b) Volume rendered image of the post-procedural MRI
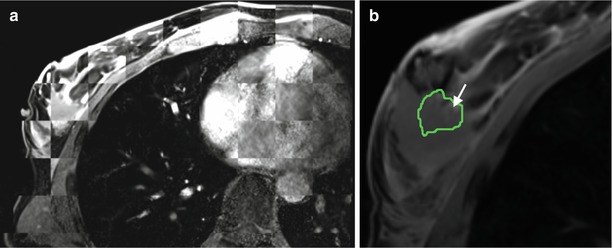
Fig. 62.8
(a) Checkerboard filter showing the result of the non-rigid registration algorithm with alternate squares of pre-procedural and post-procedural first post-contrast DCE-MRI (b) Tumor outline (green) registered to the post-procedural DCE-MRI. Arrow shows possible remnant tumor
The first patient of this series was operated on in BWH’s AMIGO Suite, an OR with adjoining MRI and PET/CT rooms. The 3 T MRI scanner (Siemens Healthcare, Erlangen, Germany) was integrated such that it can be moved via ceiling tracks into the OR as needed and removed subsequent to image acquisition (IMRIS Inc, Winnipeg, Canada). Feasibility was successfully demonstrated. The pre-procedure scan showed, as expected, that the breast deforms significantly, particularly compared with the standard diagnostic, prone image. This finding underscores the importance of preoperative supine MRI which simulates the intraoperative position of the breast. The technique needs to accommodate the intraprocedural retraction of the breast in order to be most helpful to the surgeon to map out margins adequately. It was also demonstrated that in an operative field, there is no significant enhancement from bleeding vessels if adequate hemostasis is obtained. However, fiducials are needed for accurate orientation of the margins [67]. Figures 62.5–62.8 illustrates the first procedure.
Tomikawa et al. [60] treated two cancer patients with 0.4 T MRI guidance. MRI-visible fiducials were placed on the breast, and the images were registered to the patient for navigation. A 3D model of the tumor was created and targeted with a needle for visible dye injection. The dye was used to indicate the dissection line (Fig. 62.9). Post-resection MRI and specimen mammography were performed. Both patients had negative margins upon histology.
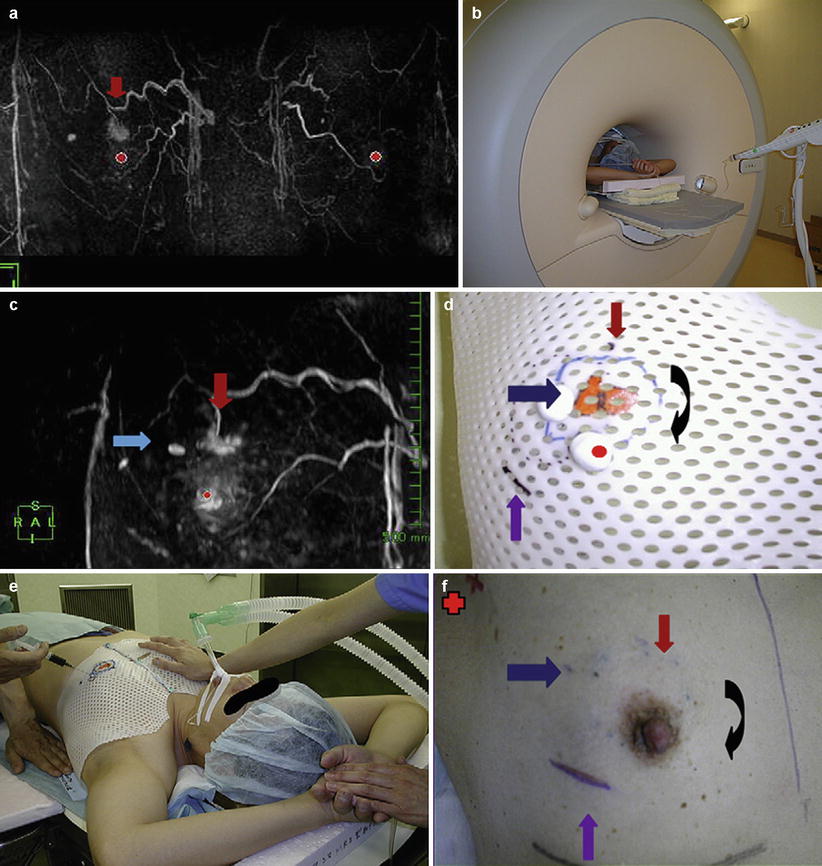
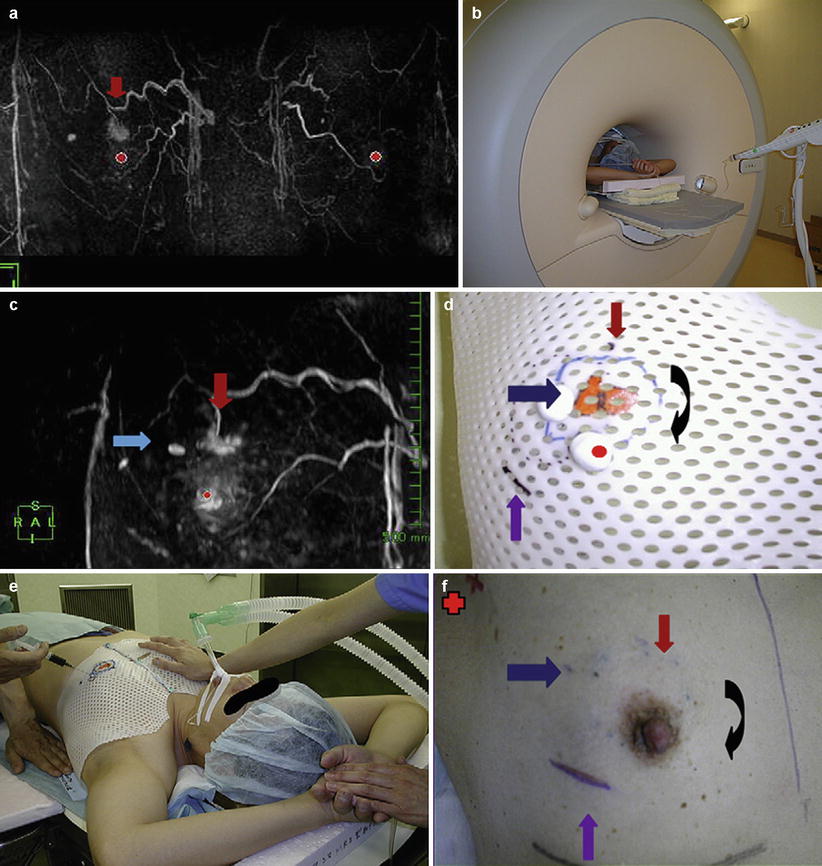
Fig. 62.9
(a) The bilateral breast MRI, performed with patients in the prone position, used for preoperative staging of lesions before breast-conserving treatment and for synchronous tumors. The red arrow shows the cancer lesion. (b) The MRI examination with the patient in the supine position for making the MRI shell. (c) The MRI with the patient in the supine position shows the same lesion as seen in (a) (red arrow). The blue arrow shows the needle biopsy scar. (d) The MRI shell designed for the resection line. Red arrow, cancer lesion; black arrow, resection line; blue arrow, needle biopsy scar; purple arrow, old biopsy scar. (e) With the patient under general anesthesia, the dye is injected into the patient’s breast through the shell pores on the determined resection line 1 cm away from the tumor border. (f) The appearance of the breast after dye injection. Red arrow, cancer lesion; black arrow, resection line; blue arrow, needle biopsy scar; purple arrow, old biopsy scar; red cross, isotope sentinel point (Reproduced from Abe et al. [59])
Intraoperative (Non-MR-Guided) Adjuvant and Competitive Guidance Modalities for Lumpectomy
Intraoperative Ultrasound (IOUS)-Guided Lumpectomy
In contrast to MRI, US is portable, affordable, and – recently – readily available in the operating room. The sensitivity of US is, however, much lower than that of MRI. In one surveillance study for high-risk breast cancer, sensitivity was reported to be 40 and 97.2 % for US and MRI, respectively [68]. Efforts to guide breast lumpectomy of nonpalpable lesions with IOUS date back more than 30 years [69]. Incremental improvement of technique and outcome assessment is ongoing.
In a feasibility study, Fornage et al. [70] reported the mass was clearly identified on IOUS in all selective 26 patients. Harlow et al. [71] report overall success in achieving pathologically negative excision margins at first operation was 97 % (63 of 65 cancers), with a 0.8 cm mean distance to the closest margin of excision. Three patients underwent a second operative procedure, two for positive margins and one for a margin <1 mm. In a feasibility study, Rahusen et al. [72] reported 17 of 19 carcinomas (89 %) were excised with adequate margins with IOUS. In the WL group, 17 of the 43 (40 %) carcinomas had been resected with adequate margins. These early and disparate results call attention to the widely varying outcome across institutions, likely due to varying aggressiveness of volume taken of normal tissue to achieve positive margins. Snider and Morrison [73] also showed an excellent rate of tumor-free resection margins using IOUS (82 %) with a smaller volume of healthy breast tissue resection compared surgery subsequent to WL (62.2 and 81.1 cm3, respectively). Moore et al. [74] reported on use of IOUS for palpable invasive ductal breast cancer. One of 27 (3.5 %) patients in the IOUS group had a positive margin (infiltrating ductal carcinoma in a single margin). This patient underwent re-excision and no residual disease was identified. Seven of 24 (29 %) patients in the control group, with palpation guidance, had a positive margin (p < 0.05). The margin of uninvolved breast tissue was greater (7.6 ± 2.0 mm vs. 4.8 ± 1.4 mm) in the IOUS patients compared with the controls. A total of 24 patients had dense breasts on preoperative mammograms; 10 were randomized to the IOUS group and 14 to the control group. In these patients, the specimen volume was 127 cm3 in the IOUS group and 180 cm3 in the control group. If the breast tissue was not dense, the use of IOUS made little difference (90 cm3 vs. 102 cm3). In a randomized trial conducted by Rahusen et al. [75], the excision was adequate in 24 of 27 (89 %) IOUS-guided excisions and in 12 of 22 (55 %) in WL-guided excisions (p = 0.007). Mean tumor diameter, specimen weight, and operating time were similar in both groups. Kaufman et al. [76] achieved negative margins for invasive carcinoma in 67 of 69 (97 %) patients undergoing IOUS for WL. Re-excisions were performed in only 4 of 69 (6 %) of patients. Overall negative margins were found in 62 of 69 (90 %) of patients. Most positive margins (71 %) were due to the presence of noncalcified DCIS. Mastectomy was necessary in 4 % of patients, usually due to multifocal invasive carcinoma. Results remained consistent when the patient population was expanded to 100 [77]. Ngô et al. [78] identified the lesion in 67 of 70 (95.7 %) patients using IOUS. Two of the three lesions not detected were ≤5 mm in diameter in patients with a body mass index of ≥25 (normal range, 19–24). Resection margins free of invasive lesions were obtained in 66 cases (94.3 %). Three patients (4.3 %) required surgical re-excision, one mastectomy due to multifocal cancer and two lumpectomy due to positive resection margins. James et al. [79] reported that positive margin rate for the 96 IOUS DCIS patients was 10.4 %, and close margins (<1 mm) were observed in 22.9 % after initial surgery. Re-excision was performed in 20.8 % of the patients. The 59 DCIS patients who underwent surgery after WL had positive and close margin rate of 12 and 27 %, respectively, with a re-excision rate of 30.5 %. A saving of $925 per case was noted for the IOUS procedures compared to preoperative mammographic WL, although details were not provided and it is unclear if this savings would translate to other institutions. Krekel et al. [80] reported 117 of 201 (58 %) were guided by WL, 52 (26 %) by IOUS, and 32 (16 %) by radio-guided occult lesion localization (ROLL). The rate of focally positive and positive margins for invasive carcinoma was significantly lower in the IOUS group (N = 2 (3.7 %)) compared to the WL (N = 25 (21.3 %)) and radio-guided occult lesion localization (N = 8 (25 %)) groups (p = 0.023). Expanding the study to a multicenter randomized controlled clinical trial for palpable tumors, Krekel et al. [81] intend to recruit 120 (60 IOUS, 60 control) women in attempts to prove the assertion that use of IOUS will result in reduced resection volume while maintaining clear margins with the aim of improved cosmesis. In a 45 patient study, Olsha et al. [82] showed IOUS margins ≥0.5 cm achieved adequate pathology margins of ≥0.2 cm in 95 % of margins. Overestimation of pathology margins by ultrasound measurement was significantly affected by multifocality (p = 0.0473). Tumor size, palpability, invasive lobular histology, and presence of DCIS did not cause significant overestimation of pathology margins by ultrasound. Inoue et al. [83] obtained 3D IOUS images in 38 of 40 (95 %) patients with primary breast cancer (stage 0–II). The tumor size was measured in a coronal view of the 3D tumor image and compared and correlated well with the pathologic tumor size (r = 0.898), which is an improved over assessment of size by palpation.
In summary, in patients with US visible lesions, IOUS has shown promise for elimination of WL procedures with the attending scheduling logistics and radiation exposure. In these cases, the concern of inadvertent wire displacement is obviated. The logistic trade-off is that personnel to acquire and interpret the images must be available at the time of surgery. A reported caveat in using IOUS is that only 50 % of the nonpalpable breast lesions can be visualized by ultrasonography [84]. Another limitation is the unreliability of ultrasound detecting DCIS lesions associated with calcifications [85].
Breast Molecular Imaging: Radio-Guided Lumpectomy
Breast molecular imaging, also known as breast-specific gamma imaging (BSGI) has been shown to be both sensitive and specific for the detection of breast cancer. Brem et al. [86] showed in a study of 146 patients that BSGI has high sensitivity (96.4 %) and moderate specificity (59.5 %) in helping detect breast cancers.
Radio (isotope)-guided surgery has gained rapid recognition and utilization for sentinel node biopsy in breast cancer and melanoma. A further extension of this technique is its application to localization of nonpalpable breast lesions. ROLL in breast was pioneered at the European Institute of Oncology. Luini et al. [87] reported on 30 patients where an occult lesion was injected with 99mTc-labeled colloidal albumin under radiologic guidance. A gamma probe was then used to locate the lesion and guide its surgical removal. They found that in the WL group, the distance from the lesion center to the specimen margin was 24.5 mm (range 15–45 mm), while that for the radio-guided group was 13.8 mm (range 5–25 mm). The mean lesion centricity (difference between the maximum and the minimum distance from the lesion border to specimen margin) was 6.2 mm (range 2.15 mm) compared to 3.8 mm (range 1–10 mm) in the radio-guided group. The authors concluded that the gamma probe allowed rapid, easy, and accurate removal of occult breast lesions with reduced excision volume and better centering within the specimen.
Chow et al. [88] applied the same technique as used by Luini to 74 patients. The breast lesion was successfully localized and excised in 73 of 74 patients (99 %), and complete excision was achieved in 61 patients (82 %). Twelve patients had a second operation for involved or close (<1 mm) margins. The group did a retrospective comparison of this method to WL and found that the localization was faster with ROLL. The need for reoperation because of an inadequate margin was higher with WL than with ROLL, but the difference was not statistically significant (32 % vs. 16 %, p = 0.129).
There are limitations to ROLL. For a large area of calcification, the extent of resection is difficult to mark by isotope, and there is concern for radiation exposure. Additionally, the majority of these patients also need sentinel node biopsy, which is done via isotope guidance as per standard of care. Studies have shown that intratumoral injection of isotope for lesion localization has resulted in a lower rate of sentinel node localization [88].
Looking to overcome the limitation that radiopharmaceutical uptake is more common in tumors larger than 1 cm or those that are palpable when injected systemically, Duarte et al. [89] sought to improve on the specificity and sensitivity of gamma probe radio-guided surgery by using the association of MRI. The aim was to use IV radionucleotide material to identify multicentricity of breast cancer. In a feasibility study of ten patients, all demonstrated 99mTc sestamibi uptake in tumor cells. Eight patients showed no additional disease, one presented with one foci of invasive ductal carcinoma, measuring 0.5 cm located 5 cm from known tumor bed, and one presented with one foci of DCIS measuring 0.8 cm at the resection margin. Although this feasibility study was done in combination with the surgical intervention being modified radical mastectomy, the technique may have application in lumpectomy, where radio-guided surgery with 99mTc sestamibi is based on the increased metabolism of cancer cells compared to normal breast tissue, while the use of MRI, potentially intraoperative MRI, provides a sensitive examination that can show the entire anatomic extent of the lesion. Radio-guided surgery in association with MRI provided a more helpful guidance of actual tumor extent during surgery [90].
Radioactive seed localization (RSL) is another method for radio-guided surgery for nonpalpable breast cancer. Gray et al. [91] randomized 97 patients with nonpalpable breast lesions to either RSL or WL. This study demonstrated that RSL was as effective as WL for the excision of nonpalpable breast lesions, and it reduced the incidence of pathologically involved margins of excision. Fewer RSL patients required re-excision of margins (26 % vs. 57 %, respectively, p = 0.02). The study also reported no significant difference in surgical excision time. This finding was consistent with other studies such as that done by Jakub et al., where they reduced the incidence of positive margins from 24 to 10 % (p = 0.01) [92].
Intraoperative portable PET probes enable detection of other isotopes. The device could be used synergistically with a preoperative PET images to intraoperatively accurately identify tumor tissue elucidated on the preoperative images. Using the handheld beta and gamma probes, González et al. [93] identified all 17 lesions seen on PET in a mesothelioma model in rats using (18)F-FDG. Smaller tumors not visualized on PET were detected intraoperatively with the probe and found malignant on pathology. Using both beta and gamma probes, Strong et al. were able to intraoperatively detect all lesions seen on PET using (124)I-labeled humanized monoclonal antibodies specific for colorectal cancer (huA33) and renal tumors (cG250). Wendler et al. [94] concurrently acquired IOUS images during the PET probe acquisition and found that the addition of functional imaging significantly improves the accuracy of localizing and identifying malignant and benign lesions. Furthermore, the study demonstrated that the inclusion of an advanced, augmented visualization provides more reliability and confidence on lesions classification. The same group also demonstrated in phantoms, 3D imaging by tracking the probe and stitching together the component images [95].
Positron emission mammography (PEM) uses dedicated scanners and F18-labeled FDG to determine the metabolic activity of suspicious lesions in the breast. PEM requires a compression of the breast similarly to mammograms and currently is not applicable for intraoperative use.
Berg et al. [96] compared the performance of PEM with that of MRI in the evaluation of the 472 ipsilateral breasts of women with newly diagnosed cancer. MR imaging had slightly greater sensitivity and more accurately depicted the need for mastectomy, while PEM had greater specificity. Eighty-nine (23 %) participants required more extensive surgery: 61 (69 %) of these women were identified with MR imaging, and 41 (46 %) were identified with PEM (p = 0.003).
Near-Infrared Fluorescence (NIRF) Optical Imaging
The NIRF images may someday be a viable candidate to elucidate biomarkers in the resection cavity. Fluorochromes can be injected to target vascular endothelial growth factor receptors, epidermal growth factor (EGF) receptor, or the HER2/neu receptor [97–102]. Activated probes have the advantage in that they exhibit little fluorescence activity in their native state until they are excited by a specific enzyme such as cathepsin B, cathepsin D, and matrix metalloproteinase 2 (MMP2) [103–109]. Images made from fluorescence can be overlaid on a visible light image for a frame of reference.
It is feasible to create a NIRF probe for in vivo assessment of margin status, with resolution on the order of 10 μm. Imaging superficial structures in the resection cavity may overcome some of the limitations of the technique arising from absorption and scattering of light [110] and reemission of light by intrinsic tissue fluorochromes which can reduce sensitivity [111]. Foremost, the limited depth resolution as well as the nonlinear signal dependence on the optical properties of tissue and the depth of the activity are constrained in superficial imaging [112, 113].
Diffuse Optical Tomography (DOT)
In contrast to optical coherence tomography, which is reviewed later in this chapter, DOT permits noninvasive optical imaging of the whole breast but is incapable of resolving features at the cellular level. Although the technique has been used in staging, to our knowledge, the technique has yet to be intraoperatively applied. Ultrasound is typically applied to target the lesion for DOT imaging and provide a background as a frame of reference. Dual wavelength near infrared is used to calculate total hemoglobin (tHb) concentration, which is indicative of angiogenesis [114]. You et al. [115] have shown that tHb is significantly higher in malignant lesions compared to benign lesions. Using histopathology as a gold standard, sensitivity, specificity, accuracy, positive predictive value, and negative predictive value of DOT were 83.9, 66.7, 76.2, 75.6, and 77.1 %, respectively, in 214 lesions from 198 women undergoing lumpectomy. In a similar study, Kim et al. [116] show excellent agreement with the tHb metric and histopathology (intra-class correlation coefficient score 0.888), however, with only marginal improvement over US alone. Zhu et al. [114] compared DOT and histopathology in 178 women and found the sensitivity, specificity, positive predictive value, and negative predictive value for in situ carcinomas and T1 cancers were 92, 93, 81, and 97 %. The corresponding values for T2–T4 tumors were 75, 93, 69, and 95 %, respectively. Intraoperative detection of positive margins is predicated on the vessel bed feeding the tumor remaining intact subsequent to resection, just as in dynamic contrast-enhanced MRI.
MRI-Guided Tumor Ablation of the Breast
As a natural extension of breast biopsy and its attending image-guided targeting techniques, therapeutic probes can be percutaneously placed in the lesion to deliver cooling (cryoablation) or heating energy (radio-frequency ablation, laser interstitial thermal therapy) intended to cause cell death. Such ablative techniques represent an emerging trend in minimally invasive approaches which, when applied to the breast, offer an alternative to lumpectomy and should prove to be less disruptive to the contour of the breast, carry less risk of infection, do not require general anesthesia, and should require less recovery time. Taking this trend to the limit, high-intensity focused ultrasound delivers thermal energy without piecing the skin.
The aim of thermal therapies is to treat the lesions and a surrounding margin while sparing normal tissue. The role of imaging is to aid the clinician in planning the probe placement for optimal coverage, targeting the lesion, and monitoring the deposition of energy. The advantages of MRI guidance in these tasks are 3D visualization via multiplanar, multi-slice acquisition, high sensitivity and delineation for breast lesions, and tissue thermal sensitivity.
If image-guided minimally invasive tumor ablation is to be competitive with the current standard of care in the treatment of breast cancer, these emerging treatments must be as effective in completely destroying cancerous tissue as the established breast-conserving treatment – surgery followed by whole-breast radiation. There are many caveats, discussed later in the chapter, which should be well understood before widely adopting ablative approaches. Careful inclusion/exclusion criteria and control measures are critical elements of proper study design if in situ ablative methods are to become a viable alternative treatment to lumpectomy for patients with early-stage breast cancer. Ablative techniques may also have value in palliative care of patients with stage 4 breast cancer [117] and recurrent disease or for patients who refuse surgery [118].
Breast Cryoablation and Cryolumpectomy
Cryoablation is a method used to ablate tumor tissue using freezing temperatures. With the current generation of the technology, needlelike probes are introduced through a nick in the skin and cooled with nozzled gas utilizing the Joule-Thompson effect [119].
Although most breast ablation has been guided with US [120], MRI is particularly well suited for monitoring the growth of the iceball. MRI, unlike US, is not subject to shadowing, which obscures the tissue beyond the iceball [121]. On MRI, the iceball appears as a signal void due to the short T2* of the crystalized water. Tissue below 0 °C is visualized as a signal void on MRI, whereas the kill zone (−30 C to −50 C) is typically 5–10 mm inside the iceball, necessitating the treatment margin [122]. With advanced MR techniques, it is possible to map isotherms inside the frozen tissue [123]. Although most experience with MRI-guided cryoablation has been gained with the treatment of liver and kidney lesions [124], a few breast studies have been performed. In a feasibility study, Morin et al. [125] reported on the MRI-guided cryoablation of 25 patients with breast carcinoma without complications. Four weeks after treatment, surgical excision was performed for histopathologic correlation. Total ablation was achieved in 13 of the 25 tumors treated. Pusztaszeri et al. [126] reported on 11 MRI-guided procedures (with one patient not evaluated due to technical problems), followed by resection 4–5 weeks post therapy. They report that all ten of the reviewed targeted tumors were within the iceball, but only two patients had a complete response. The authors suggest that DCIS was more resistant to the cryotherapy than was invasive carcinoma and moreover that components of the previously undetected DCIS in the larger tumors were far from the two probes used. Cell death is related to the lowest temperature reached, the amount of time at subzero temperatures, and the number of freeze-thaw cycles [127]. The clinical liver/kidney literature suggests longer treatment times (e.g., 15-min freeze, 10-min thaw, 15-min freeze) may be more effective than the two 5-min freezing cycles used in this study. Moreover, use of an odd number of probes has been shown to provide better coverage of spherical tumors than an even number [128]. Littrup et al. [129] studied the effects of number of probes and freezing power in a phantom; the results should be extended to clinical practice and validated in the presence of heat sinks like the chest wall. Five patients in the Pusztaszeri suffered from skin necrosis, a complication which can be avoided by selection criteria for minimum distance between the lesion and the skin or managed with the use of warm saline on the skin or saline injection [130]. The Morin and Pusztaszeri studies employed cryoprobes made from materials with low MRI artifact driven by a device designed to operate without introducing electromagnetic interference into MR images. In these studies, the patient was supine. More recently, Tozaki et al. [131] treated a single patient with core needle biopsy-proven invasive ductal carcinoma without intraductal component, using a non-MRI-compatible cryotherapy system. MR imaging of a prone patient with a breast coil was used to define the target tissue. US guidance was used to place the probes, using an US system safely integrated into the MRI room. At the end of the freeze, the non-MRI-compatible cryoprobes were briefly heated to dislodge them from the iceball and removed from the breast. MR images were acquired to visualize the iceball, and after further thawing, contrast-enhanced MR images were acquired. The treatment protocol was 10-min freeze, 10-min thaw, 10-min freeze. At 9-week MRI evaluation, the lesion was not enhancing and was shown to be inside the cryozone. No viable cancer cells were noted on histology following lumpectomy at 14 weeks.
The preponderance of experience in breast cryoablation was gained with US guidance and US monitoring. Fibroadenoma, a benign lesion, has been the breast disease most commonly treated with cryoablation, with some authors reducing the practice to an office visit [132–134]. A typical response to treatment is localized tenderness and swelling, which resolves by 3 weeks. The breast returns to baseline by 6 weeks, and the necrotic fibroadenoma tissue reabsorbs over the next several months.
Another potential application of cryoablation is palliation of locally advanced breast carcinoma and palliation; the reported results showed acceptable local tumor control [118, 135–137].
The use of cryoablation during surgical resection (cryolumpectomy) has been investigated, where the cryoprobe takes the place of a hook. The iceball is resected aiding in the aim of en bloc tumor removal. A 310-patient randomized multicenter study [136] concluded that positive margin status and re-excision rate status did not differ from conventional lumpectomy. However, the volume of tissue removed in cryolumpectomy was significantly lower than in conventional lumpectomy, with improved quality of specimen, surgical cosmesis, short-term cosmesis, patient satisfaction, and overall procedure time for the patient.
The most driving question, however, is can breast cryoablation supplant lumpectomy for resectable disease? An active Phase II National Cancer Institute trial [137, 138] is attempting to answer this question. It is of interest that negative margins were reported for 77 of 78 patients comprising a subpopulation with tumor size <10 mm, enrolled in five different studies [138–143]. Complete treatment for tumors greater than 10 mm and less than 15 mm was noted for the remaining patients with no DCIS. Since efficacy of the freeze falls off with distance from the probe, the number and placement of probes should be carefully planned and executed, in order to effectively treat larger lesions. Pusztaszeri et al.’s [126] claim that certain tumor types are cryo resistant and should be tested in ex vivo experiments [144], animal models [145], and careful clinical practice. The possible immune response to the ablated tissue also demands investigation. Leaving the frozen tissue in situ for the body to absorb potentially stimulates an immunologic response to tumor-specific antigens in the frozen tissue. In this manner, cryoablation may serve as both a local and systemic therapy for breast cancer [146].
MRI Temperature Mapping in the Breast
A tool common to the ablative techniques that use elevated temperature is noninvasive MRI temperature mapping, based on temperature-sensitive MR parameters such as the proton resonance frequency (PRF), the diffusion coefficient (D), T1 and T2 relaxation times, magnetization transfer, the proton density, as well as temperature-sensitive contrast agents [147]. Thermal dose can be extracted from time-temperature curves, and through empirical experimentation cell death can be correlated with thermal dose [148]. Proton resonance frequency shift (PRFS) can be exploited to measure temperature in aqueous tissue; however, chemical shift in fat is nearly constant over the temperatures used in thermal ablation. The T1 temperature dependence can be exploited in fat [149, 150].
In supine positioning, without breast fixation, imaging techniques to compensate for motion must be applied [151, 152]. An additional complication in the breast comes from heat-induced changes in susceptibility. The changes in susceptibility for the water signal are negligible, but the change in susceptibility of fat is much larger. Thus, even with fat suppression methods, the changes in the susceptibility must be taken into consideration [153].
Breast Radio-Frequency Ablation (RFA)
Radio-frequency ablation (RFA) refers to the destruction of tissue via the application of electromagnetic fields, created by interstitial electrode delivery of high-frequency waves (0.4–8 MHz). Current density is induced in the tissue, causing resistive heating. Current is returned through a gel pad electrode placed on the thigh or back. RF power deposition is a function of tissue conductivity and is difficult to predict and control. The formation of the lesion may be inhomogeneous especially in regions of tissue boundaries. Also, susceptibility artifacts around the probe during MR imaging prevented accurate temperature monitoring. No monopolar commercial solution is at this time available to remedy the problem of electromagnetic interference emitting from the RF generator manifesting itself as noise in the MR images. Several research sites have implemented gating [154] or filtering solutions.
First efforts have been made in clinical application of MRI-guided breast RFA by van den Bosch et al. [155] (Fig. 62.10). Feasibility was demonstrated in three patients. US-guided large-core needle biopsy confirmed invasive ductal carcinoma in all three patients, with DCIS adjacent to the invasive lesion in patients 2 and 3. These three patients were considered to be candidates for MRI-guided RFA study, because their breast lesions were located centrally in the breast, implying a distance of more than 1 cm to the skin or chest wall, reducing the risk of skin burn during the RFA procedure. The treat and resect protocol ensured complete tumor treatment regardless of RFA outcome in all patients. Patients were positioned prone in a 0.5 T vertically open MRI scanner. A simple flexible loop coil gave unfettered access to the breast and no compression plate was used. Imaging was used to confirm deployment of the RF probe tines, which can be challenging in fibrous tissue. The MRI temperature mapping was robust to motion since rather than referencing the temperature change to a baseline image, non-heated tissue in the current image was referenced. Measurements from a fiber optic temperature probe were used for comparison. Histopathology confirmed successful (100 %) tumor ablation in one patient and partial tumor destruction (33 and 50 %, respectively) in two patients. The lesion size was likely underestimated on MR in the latter two cases. The authors note that susceptibility artifact caused by the probe, which was >6 mm diameter, will create a challenge for temperature mapping in lesions <10 mm. This problem will be even more evident with higher field strength MRI scanners. Imaging techniques [156] and careful materials selection and tooling of the probes may mitigate this problem. High success rate for the technique in other organs [157] may drive industry to provide complete solutions for MRI-guided RFA.
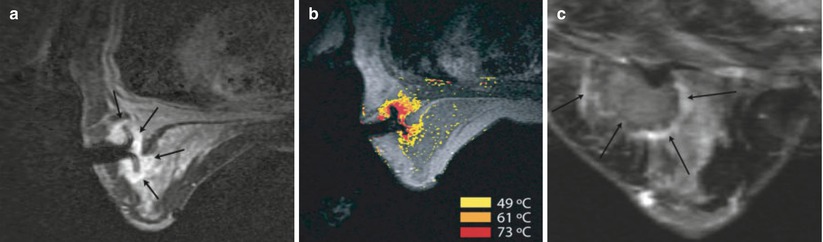
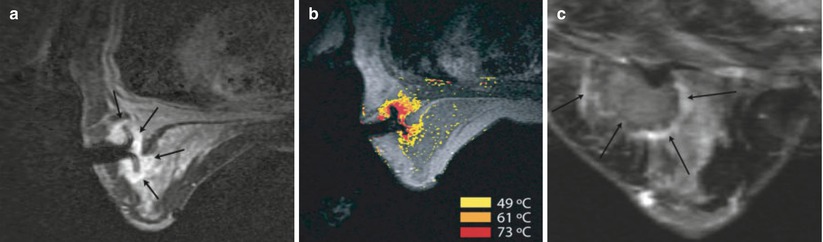
Fig. 62.10
MRI-guided RF ablation (a) Axial contrast-enhanced (CE) three-point Dixon gradient-echo images (150/12.8, 19.8, 26.8; flip angle 90°) with patient prone position showing the enhancing tumor mass (arrow) lateral in the right breast with the hypointense fully deployed LeVeen needle electrode centrally in the mass. (b) Same imaging protocol with the same patient in prone position showing the magnetic resonance PRF shift thermomap (yellow zone 49 °C, orange 61 °C, red 73 °C) around the hypointense deployed RFA electrode centrally in the mass. (c) Post-procedure CE water-selective, spectral-spatial axial FSE image of the right breast demonstrates a small enhancing rim representing the border of the ablation zone corresponding to fresh scar tissue (arrows) (Reproduced from van den Bosch et al. [155])
Multiple centers have applied US-guided breast RFA for uses such as elderly inoperable patients [158, 159], local recurrence [160], as an adjuvant to vacuum-assisted excision in local disease [161] and, like cryoablation, predominantly to small operable primary tumors [158, 162–167] with varying degrees of success [168–170].
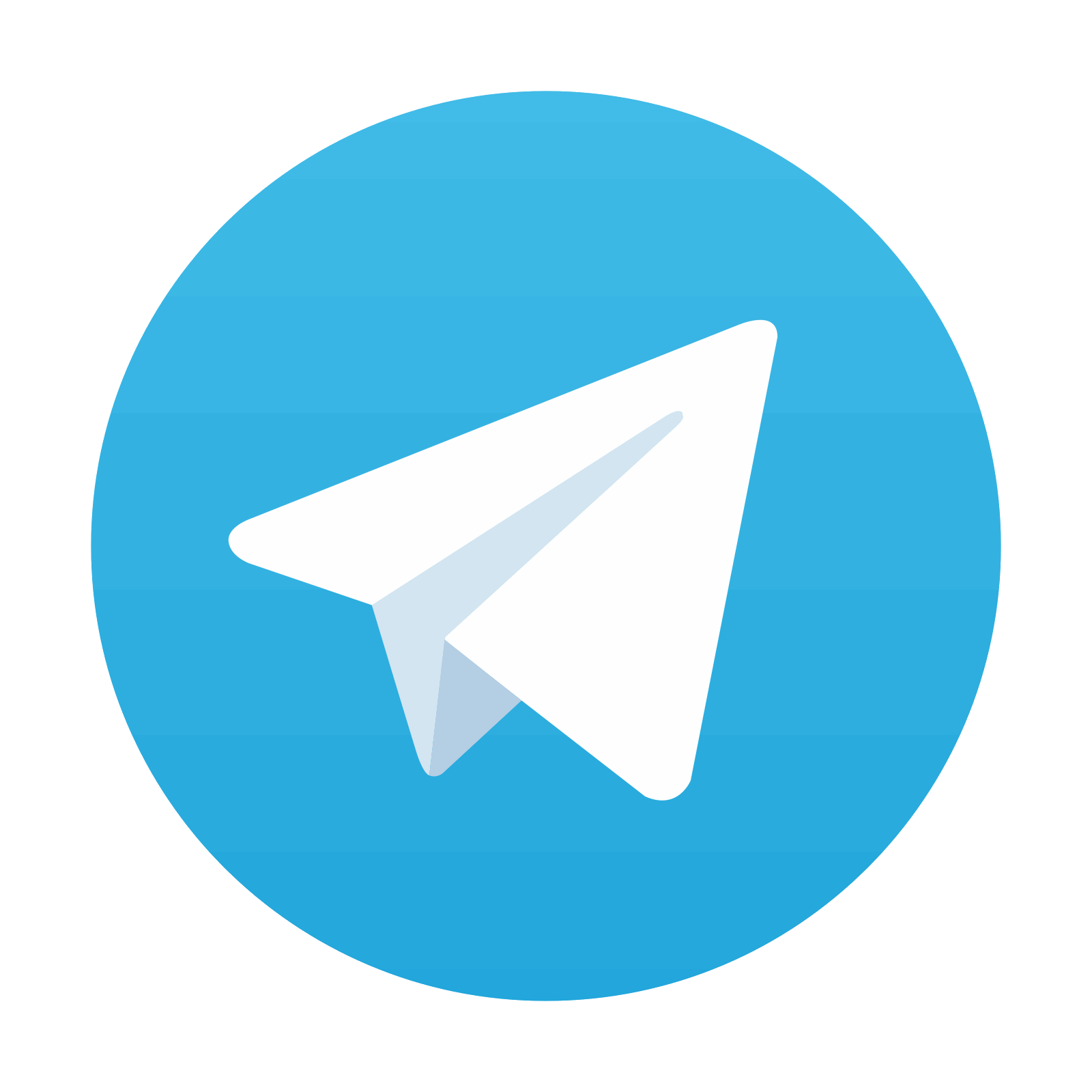
Stay updated, free articles. Join our Telegram channel

Full access? Get Clinical Tree
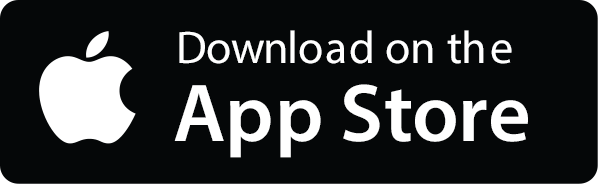
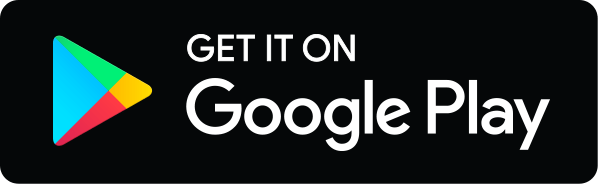