Magnetic Resonance Imaging of the Breast
7.1 Technique and Methods
7.1.1 Basic Principles
Magnetic resonance imaging (MRI) is based on the physical principle of nuclear magnetic resonance, first demonstrated in solids by Purcell and Bloch in 1946. Classically, electrically charged particles produce a local magnetic field when they have rotational motion. Atomic nuclei with an odd number of protons and neutrons have an intrinsic “spin” (analogous to classical angular momentum) and have a resultant magnetic moment. Because of their high concentration in biological tissues (in water and fat), protons are the primary particle used for imaging purposes. Furthermore, the hydrogen nucleus has the largest magnetic moment of all naturally occurring isotopes. When such nuclei are subjected to a strong external magnetic field, their spins align themselves with this static magnetic field either in a parallel orientation (low-energy state) or an antiparallel orientation (high-energy state). Only the spins oriented in an antiparallel direction (1:105) contribute to the signal generation. In addition, the external magnetic field exerts a torque on the individual magnetic moments of the protons, causing them to gyrate (precess) around the magnetic field direction—i.e., the longitudinal axis—at a certain frequency. This “Larmor frequency” (ω, omega) is proportional to the strength of the external magnetic field (B0) according to the formula
where the proportionality constant y is called the gyromagnetic ratio and represents a material-specific constant.
When an external electromagnetic pulse of high-frequency (a radiofrequency [RF] pulse) that corresponds to the Larmor frequency of the proton is applied, the individual magnetic moments are deflected out of alignment with the longitudinal axis of the external magnetic field. The amount of this deflection is characterized by the excitation pulse, measured in angular degrees (°). After the external electromagnetic pulse is turned off, the magnetic moments tend to return to their original state of longitudinal alignment and precession: this is the process of relaxation. During the relaxation process, the absorbed input energy is released in the form of radio waves. Further energy is lost through electromagnetic dipole–dipole interaction and thermal dissipation. The restoration of the magnetization vector to its original state of alignment is an exponential process characterized by the increase of magnetization in the longitudinal plane (T1 relaxation time) and the loss of transverse magnetization (T2 relaxation time or T2 decay). The decrease in the transverse magnetization is caused by local magnetic field inhomogeneities and temporally fluctuating magnetic fields, so-called spin–spin interactions. Due to these interactions, some the magnetic moments of protons will be caused to precess faster and some to precess slower, and thus to fall out-of-phase (dephasing). The T1 and T2 relaxation times are unique and intrinsic properties of the tissue examined. The emitted electromagnetic signal (magnetic resonance [MR] signal) can be detected as an induced voltage by an appropriate receiver coil and processed to produce an image. The relaxation times are the times that it takes for the RF signal to exponentially rise (T1) or decay (T2) to half its maximum value.
For image generation, an emitted signal must be identified with a specific location on a three-dimensional matrix using gradient fields in the three spatial axes (x, y, z). Spatial encoding can be done using 2D or 3D techniques.
2D technique: Here spatial encoding is carried out by applying a linear magnetic field gradient along a selected plane (x, y, or z gradient), then applying an RF pulse with a selected center frequency (resonant frequency) that excites only spins located within a specific slice along that gradient (slice selection). Phase and frequency encoding within this slice is achieved by application of magnetic field gradients along the other two axes. This allows the spatial localization of MR signals from each voxel within the slice, which can then be used to create an image.
3D technique: In contrast, for 3D acquisition, an entire tissue volume is excited. An additional phase-encoding gradient must therefore be applied along the third orthogonal dimension (e.g., the z-axis) to allow spatial encoding along the slice-select direction, thereby generating a set of contiguous slices within the volume.
Factors that influence contrast in an MR image include factors inherent in the tissue and also external variables. The most important of the inherent factors are the proton density and the T1 and T2 relaxation times. The external factors include hardware and software parameters such as slice thickness, angulation, and acquisitions; the type of pulse sequence chosen, such as SE (spin echo), GRE (gradient echo), and fat saturation; the field strength; and whether a contrast agent is administered.
A variety of different pulse sequences are available for MR imaging. The basic principles of the SE sequences and GRE sequences (of special significance for dynamic breast MRI) are explained below.
SE sequences: For classical SE (spin-echo) sequences, excitation always occurs by application of a 90° RF pulse that completely abolishes the longitudinal magnetization and converts it into transverse magnetization. After half of the echo time (TE), a second, 180° RF pulse is applied. This flips the vectors so that precession now brings the vectors back to their starting positions; that is, it leads to a rephasing or refocusing of the spins, resulting in a maximum signal (spin echo) that is read out after the full TE. Successive repetitions of the entire sequence are performed after the lapse of a given repetition time (TR). Depending on the chosen TE and TR, resulting images that are either T1 weighted, proton-weighted, or T2 weighted.
GRE sequences: Gradient-echo (GRE) pulse sequences use a reversal of gradient polarity to form an echo rather than a 180° RF pulse. Additionally, the initial 90° RF pulse can be replaced by a smaller pulse (flip angle, α < 90°) that does not completely eliminate the longitudinal magnetization. This results in more rapid image acquisition and a weaker signal yield. In comparison with SE sequences, GRE sequences show higher sensitivity for artifacts caused by susceptibility differences in tissues (bone, air) or through metal deposits. Correspondingly, the sensitivity of GRE sequences to paramagnetic contrast media is greater.
Signal intensity in MRI imaging can be modified by the administration of contrast materials. Paramagnetic substances are primarily used for this purpose, but superparamagnetic substances can also be used. Substances of both these groups exhibit the property of changing the relaxation times of the anatomical structures being imaged. Paramagnetic contrast media shorten the T1 and T2 relaxation times. Contrast-enhanced breast MRI takes advantage of this shortening effect on the T1 relaxation time to increase the signal intensity in the T1W sequences. Superparamagnetic substances have no utility for breast MR imaging.
7.1.2 Tumor Detection
MRI of the breast has high sensitivity for the detection of hypervascularized areas in the breast through the depiction of the intramammary structures in very thin layers before and after intravenous administration of a contrast agent suitable for MRI. Contrast-enhancing areas are characterized by increased vascularization, increased vessel permeability, and an enlarged interstitial space (tissue matrix). These tissue characteristics are found in almost all invasive cancers and in a majority of intraductal breast carcinomas (keyword: tumor neoangiogenesis). Some benign breast lesions, however, are also associated with an increased perfusion pattern, so that there is an overlap in the imaging characteristics of benign and malignant processes. Technical and methodological aspects of a high-quality breast MRI are discussed in the following sections.
7.1.3 Equipment
Breast MRI is generally performed in a whole-body magnet with field strengths of 1.5 and 3.0 T (tesla) (▶ Fig. 7.1a). It is no longer acceptable to use systems with 0.5 T. Special bilateral surface coils are required for breast MRI to achieve a sufficiently high spatial resolution. These are adapted to fit the shape of the female breast and should preferably be open to enable optimal support and positioning of the breast, and to allow for carrying out interventional procedures when required (▶ Fig. 7.1b). Dedicated compression plates can be integrated into such open breast coils to reduce the occurrence of motion artifacts (▶ Fig. 7.1c).
Fig. 7.1 Breast MRI. (a) 1.5 T Examination unit (CE Healthcare, Milwaukee, Wl, USA) with the surface coil in place. (b) Open surface coil with integrated compression plates (Noras, Höchberg, Germany). (c) Compression device with superior-inferior fixation of the breast (Noras, Höchberg, Germany).
Note
Open breast surface coils with integrated compression plates are an essential requirement for excellent breast MRI quality.
7.1.4 Timing of the Examination
The parenchymal perfusion pattern, and thus the contrast enhancement in the mammary gland, fluctuates with the changing hormonal influences on the female breast during the menstrual cycle. The degree of disturbing signal enhancement on breast MRI imaging is least in the second week of the menstrual cycle. Thus, when circumstances allow, an examination should be scheduled between the 7th and 14th days of the cycle. Examinations performed in the third week of the menstrual cycle are generally still acceptable, but the first and fourth weeks should be avoided. If the imaging has to be done during this unfavorable period, the potential diagnostic disadvantage from increased enhancement must be taken into account.
Practical Tip
The best time to perform breast MRI is in the second week of the menstrual cycle.
After menopause, there are no concerns related to the menstrual cycle when planning a breast MRI examination appointment. Occasionally, however, there can be undesirable early parenchymal contrast enhancement due to hormone replacement therapy (HRT), necessitating a repeat examination after stopping HRT for 4–6 weeks. As a general principle, however, it is not justifiable to stop hormone substitution before performing a breast MRI examination.
An MRI examination can be performed without restriction after a previous fine needle puncture, and after a core needle biopsy without significant hematoma. After a vacuum-assisted biopsy, there is typically a large resection cavity with reactive, hyperemic changes around the edge and hematoma in the center. In general, it is better to undertake MRI before rather than after such an interventional procedure.
Subsequent to an open biopsy, no breast MRI should be performed within an interval of 6 months so as to avoid interference from increased enhancement associated with wound healing. After tumor resection (as breast-conserving therapy [BCT]) and radiation therapy, this interval is increased to about 12 months after termination of the radiotherapy. There is, however, pronounced variability between individuals with respect to the extent and duration of the reactive changes after a course of radiation therapy.
7.1.5 Patient Positioning
The examination is performed with the examinee in the prone position with the arms along the sides, preferably tucked into the belt of a bath robe (▶ Fig. 7.2). As an alternative, the arms can be crossed above the head, although in this position the breasts are often not completely visualized in the axial slice orientation. The use of a cushioned head support on which the forehead rests has proven value, allowing the woman to view a picture motif by way of an angled mirror during the procedure.
Fig. 7.2 Prone patient positioning for breast MRI. Frontal headrest with angled mirror and picture motif for viewing during the examination. Headphones for listening to recorded music. Compression plates with positioned and fixed breast. Arms along the body with the arms tucked into the belt of the bath robe and relaxed pectoral muscles. Alarm bulb to terminate the examination if necessary.
Note
The key to a high-quality breast MRI examination is patient comfort.
7.1.6 Measurement Parameters
Technique
Contrast-enhanced breast MRI can be performed with the 2D and 3D techniques. Each technique has advantages and disadvantages that do not significantly affect the assessability or sensitivity of the MRI examination. The decision as to which technique should be used should be based on the experience of the examiner; it is not advisable, however, to alternate techniques frequently. Recently, parallel imaging has optimized image acquisition speed and spatial resolution. This is essentially simultaneous acquisition of image data over two or more receiving coils with different spatial sensitivities.
Sequences
T1-weighted (T1W) GRE sequences still represent the heart of the breast MRI examination (▶ Fig. 7.3a). Essentially, they allow sensitive capture of an enhancement after administration of an MR-appropriate paramagnetic contrast agent. These T1W sequences are typically performed once before and several times after the peripheral venous administration of contrast medium. To allow clear visualization of contrast-enhancing areas, it is necessary to eliminate or suppress interfering intramammary fat signals. This can be accomplished in two ways:
By the subtraction of identical slice images before and after administration of contrast material (“European school”).
By the acquisition of sequences with primary fat suppression, i.e., saturation (FAT-SAT) (“American school”).
Fig. 7.3 T1W and T2W sequences in breast MRI. (a) Precontrast T1W image. (b) T2W image.
The additional acquisition of a T2 weighted (T2W) sequence is recommended in order to obtain a signal-intense visualization of water-containing or grossly edematous structures (▶ Fig. 7.3b). For this, breast MRI primarily employs T2W SE or TSE (turbo spin echo) sequences, or IR (inversion recovery) sequences, combined with simultaneous fat saturation. The T2W measurement is not for tumor detection, but rather to characterize suspicious findings found in the contrast-enhanced T1W measurement.
Notes
In the T1W image, contrast media, fat, protein, and blood appear white (signal intense).
In the T2W image, water-containing substances appear white (signal intense).
Orientation
MRI allows examinations in any slice orientation. In Europe, the transverse (i.e., axial) plane is usually preferred when contrast-enhanced breast MRI is used for tumor detection; in North America, the sagittal plane is preferred. For implant evaluation, at least one complementary sagittal angulation is expressly recommended.
Temporal Resolution
In the vast majority of cases, contrast enhancement occurs more rapidly in hypervascularized breast tumors than in the surrounding glandular parenchyma. This is particularly true for invasive breast carcinomas, in which the maximum signal intensity peak is usually reached approximately 3 minutes after the intravenous administration of the contrast agent. However, some carcinomas can exhibit their maximum signal intensity peak as early as 1 minute after the administration of the contrast agent, followed by a “washout” effect. In contrast, the signal intensity of parenchyma displays a continuous increase during the entire examination time of 8 to 10 minutes, and there is a considerable degree of variation between individuals. Because early and strong contrast enhancement of parenchyma may occur (e.g., in areas of adenosis or due to hormonal stimulation), pathological processes can be masked (masking effect). Generally, a temporal resolution of maximally 2 to 2.5 minutes/sequence over a period of at least 6 minutes after contrast agent administration is required for dynamic measurements.
Spatial Resolution
Various factors and setting parameters affect the spatial resolution of breast MRI. Specifically, these are the matrix (depending on the chosen field of view), the slice thickness, and the magnitude of the resulting volume. For examinations in axial orientation, the field of view is predetermined by the patient’s proportions (usually 300–350 mm). Recommendations advise a matrix size of at least 256 × 256 pixels. Ideally, however, a matrix of 512 × 512 pixels should be used, which should not be the result of interpolation. Although general recommendations are for a slice thickness between 2 and 4 mm, breast MRI should be performed with single slice thicknesses of 1.5 to 2.5 mm. Indisputably, the best results are obtained by high-resolution breast MRI with a matrix of 512 × 512 pixels and a single slice thickness of 1.5 to 2 mm.
Contrast Medium
Intravenous administration of an extracellular paramagnetic contrast agent allows visualization of the vascularization within the breast. Repetitive measurement after the administration of contrast media gives information about the uptake and clearance of the contrast material over time. For dynamic breast MRI (1.5 T), the recommended dose of contrast agent is 0.1 mmol Gd-DTPA (gadopentetate dimeglumine) per kg body weight. The contrast agent is mechanically injected at a rate of 2 to 3 mL/s via a venous access previously placed in the cubital fossa. Gd-DTPA is cleared quickly and completely by way of renal excretion. Tubular reabsorption has not been demonstrated. The half-life of Gd-DTPA in the blood is approximately 90 minutes. After 24 hours, more than 90% of the substance has been excreted. Gd-DTPA is very well tolerated. From available data, the rate of side effects is considered to be less than 2%, and approximately 80% of side effects are classified as mild. Adequate and clear information must be provides to the patient to allow written consent to be obtained before starting the examination.
The use of Gd-DTPA during pregnancy has not been established as innocuous, so contrast-enhanced breast MRI in pregnant women should be avoided on principle. The use of gadolinium-based contrast material can lead to nephrogenic systemic fibrosis in patients with renal insufficiency. This risk is especially high in patients with acute or chronic renal insufficiency and a glomerular filtration rate less than 30 mL/min per 1.73 m3, and in patients with acute renal insufficiency in conjunction with hepatorenal syndrome or a history of liver transplantation.
Phase-encoding Gradient
Spatial orientation in three-dimensions is achieved in MRI by way of phase encoding and frequency encoding of selected slice planes. For the axial view of breast MRI, as is commonly used in Europe, the phase-encoding gradient is typically positioned in a mediolateral direction. However, this occasionally impairs the ability to evaluate the axillary aspects of the breast, with the overlapping artifacts usually being worse in the vicinity of the heart (left axillary area) than on the right side. This problem has been resolved by modifying the examination protocol to feature alternation of the phase-encoding gradient. As signal curve analysis in high-resolution breast MRI becomes less significant, revision of the established examination protocols should take new considerations into account.
Zebra Protocol
The zebra protocol (▶ Fig. 7.4) involves sequentially alternating the phase-encoding gradient. Before administration of contrast medium, one measurement is made with the phase-encoding gradient along the mediolateral or horizontal direction, and one with it along the frontoposterior or vertical direction. These serve as the base measurements for the later image subtraction. After administration of contrast medium, two measurements are performed with the phase-encoding gradient along the mediolateral direction followed by one measurement with it along the frontoposterior direction. Subsequent image postprocessing yields three subtracted images:
Earliest subtraction with mediolateral phase-encoding gradient.
Early subtraction with mediolateral phase-encoding gradient.
Late subtraction with frontoposterior phase-encoding gradient.
Fig. 7.4 Zebra protocol. Acquisition of dynamic T1 sequence slices with alternating phase-encoding gradients. (a) Precontrast examination with anteroposterior phase-encoding gradient. (b) Precontrast examination with mediolateral phase-encoding gradient. (c, d) After administration of contrast medium, two T1 image sequences with mediolateral phase-encoding gradients are acquired: first for performing the earliest subtraction (c), and second for performing the early subtraction (d). (e) After these postcontrast T1W images for the earliest and the early subtraction postprocessing are acquired, the third postcontrast T1W sequence is acquired with anteromedial phase-encoding gradient for the late image subtractions. (f) Maximum intensity projection with mediolateral phase-encoding gradient. (g) Maximum intensity projection with anteroposterior phase-encoding gradient.
The zebra protocol enables complete visualization of all the parenchymal segments, including the potential “dead spaces” in the left axillary tail region while preserving a very high temporal resolution.
7.1.7 Image Postprocessing
The multitude of images acquired in dynamic MR mammography necessitates additional postprocessing to help in the detection of contrast-enhancing lesions, the further characterization of findings, and the optimal presentation of lesions.
Image Subtraction
The subtraction of precontrast images from postcontrast images with identical slice positions serves to eliminate the signal-intense adipose tissue and facilitates detection of hypervascularized areas of tissue (▶ Fig. 7.5a). It is mandatory to subtract the precontrast examination series from the first (earliest subtraction) and the second (early subtraction) postcontrast series on a pixel-to-pixel basis. Within the zebra protocol, an additional subtraction of the precontrast series from the third series after administration of contrast medium (late subtraction) is performed, allowing an evaluation of the tissue in the axillary tails (see ▶ Fig. 7.4).
Fig. 7.5 Image postprocessing in breast MRI. (a) Subtraction. (b) Curve analysis. (c) Maximum intensity projection. (d) Computer-assisted diagnosis (CAD).
Curve Analysis
The analysis of time–signal intensity curves (TICs) within representative areas of contrast enhancement has lost importance with the increasing optimization of the spatial resolution of breast MRI. The analysis remains useful for mass lesions with defined volumes, but it does not provide any additional information in the case of multiple foci or non-space-occupying lesions. TIC measurements should be made in defined measurement areas (ROIs, regions of interest), which are selected such that they include the greatest possible proportion of the maximally enhancing area while disregarding less vascularized areas (e.g., central necrotic tissue areas). The size of the field of view here should be between 2 and 5 pixels and the selection of three ROIs per lesion is recommended. The numerical results of the time–signal intensity measurements are expressed as percentage increase over the initial signal intensity values (▶ Fig. 7.5b).
Maximum Intensity Projection
The maximum intensity projection technique yields a comprehensive three-dimensional view of both breasts. Based on the subtraction images generated by postprocessing, which only take into account image pixels having a certain signal intensity (threshold algorithm), the representation of image information gives the impression of a “transparent breast” which can be viewed from different angles (▶ Fig. 7.5c).
Computer-Assisted Image Analysis
Various models of CAD (computer-assisted diagnosis) for dynamic MRI examinations of the breast have been presented in the literature. These range from color-coded parameter images (▶ Fig. 7.5d) over automatically defined regions of interest, and from pulsating playback sequences with a repetition rate of 1 or 2 images per second to the use of pharmacokinetic models.
▶ Table 7.1 shows the breast examination protocol used at the Göttingen Women’s Health Care Center (BZG [Diagnostisches Brustzentrum Göttingen]).
Parameter | Setting |
Field strength | 1.5 T |
Surface coil | Bilateral, open |
Breast stabilization | Dedicated breast compression plates, superoinferior |
Angulation | Axial |
Technique | 3D |
Phase-encoding gradient | Zebra protocol (see ▶ Fig. 7.4) |
Sequences | T1W GRE repetitive, T2W inversion recovery with fat saturation |
Dynamics | Two measurements before, three measurements following administration of contrast media (zebra protocol) |
Contrast agent | Gd-DTPA 0.1 mmol/kg body weight |
Contrast agent injection | Cubital fossa; automated injection (3 mL/s); followed by 20 mL saline solution |
Spatial resolution | 1.5–2.5 mm |
Field of view | 300–350 mm |
Matrix | 512 × 512, noninterpolated |
Temporal resolution | 87 s/sequence |
TR | 8.4 ms |
TE | 4.1 ms (in-phase) |
7.1.8 Implant Evaluation
Examinations that target the detection of complications related to prosthetic implants differ fundamentally from examinations designed to rule out carcinoma. For one thing, implant diagnostics do not require a dynamic examination, so that IV administration of contrast medium is unnecessary. For another, special sequences come into use that allow the selective evaluation of the different prosthesis components (e.g., silicone or saline).
As opposed to contrast-enhanced breast MRI for early tumor detection, MRI examination of breast prostheses requires the acquisition of images in multiple planes. In addition to the usual axial slice orientation, sagittal images are acquired to detect any silicone located outside the superior and inferior circumference of the prosthesis, as well as to evaluate any deformation of the prosthesis in a superoinferior direction (▶ Fig. 7.6). A good prosthesis examination requires extremely high spatial resolution (1–2 mm), but this is unproblematic as there is no need for administration of contrast medium, and thus the measurement protocol has plenty of leeway regarding the temporal resolution.
Fig. 7.6 Breast implant MRI. Visualization of an implant in the usual T1W sequence with normal findings.
Fat-suppressing inversion recovery sequences are usually used, because they are most effective in differentiating between the various prosthetic fluids. By additionally suppressing the water signal, such inversion recovery sequences allow a signal-intense visualization of silicone (silicone-sensitive measurement), while the signal of the saline components and the surrounding adipose tissue is suppressed (▶ Fig. 7.7). Conversely, inversion recovery sequences combined with silicone suppression allow the selective visualization of the saline components (water-sensitive measurement), so that, especially in the case of double-lumen prostheses (silicone/saline), the two prosthetic lumina can be visualized separately.
Fig. 7.7 Breast implant with intracapsular rupture on MRI. (a) Examination with silicone-sensitive sequences and suppression of the water signal. Evidence of rupture of the implant shell and collapse with incipient linguine sign. (b) Examination with water-sensitive sequence and silicone signal suppression. Visualization of aqueous inclusions within the silicone (“salad oil sign”).
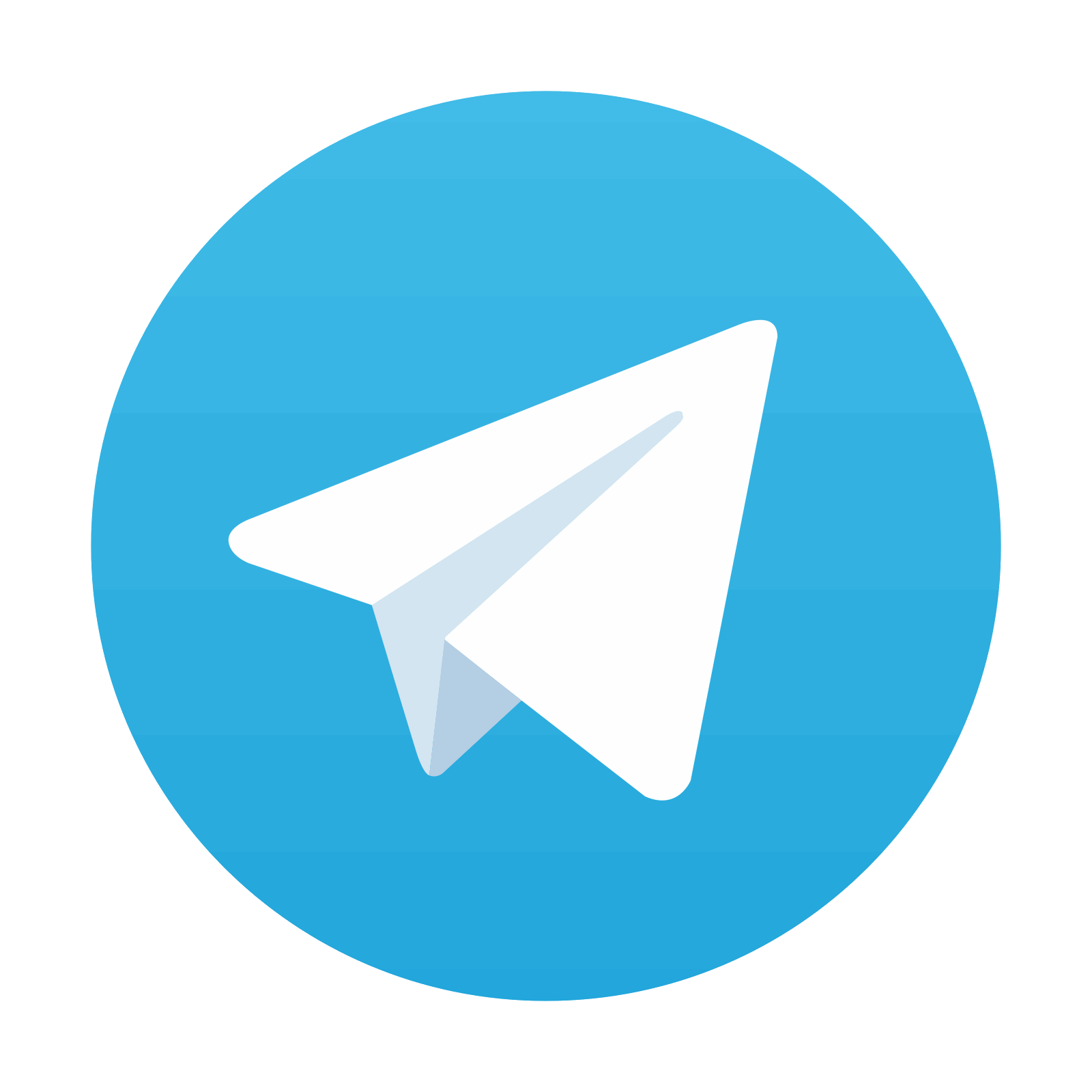
Stay updated, free articles. Join our Telegram channel

Full access? Get Clinical Tree
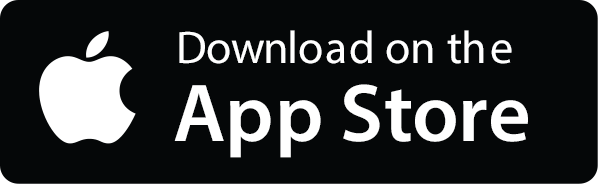
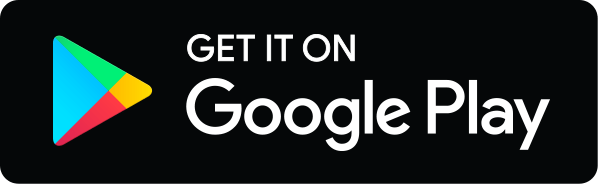