MR nephrourography (MRNU) makes it possible to obtain structural and functional data within a single imaging examination without using ionizing radiation. The functional data available with MRNU allows renal physiology to be examined in ways that were not possible previously. Coupled with the exquisite soft-tissue contrast provided by standard MR images, MRNU can provide a comprehensive study that yields critical diagnostic information on structural diseases of the kidneys and collecting system, including congenital and acquired diseases, and also on the full range of the causes of dysfunction in the transplanted kidney.
The MR imaging techniques used for liver are well suited for renal analysis. A useful imaging protocol includes a combination of (1) breath-hold T2-weighted single-shot echo-train coronal and axial images, with at least one plane performed with fat suppression; (2) T1-weighted gradient echo (GRE) precontrast axial and coronal fat-suppressed images; and (3) T1-weighted gadolinium-enhanced arterial capillary– and delayed-phase images. T1-weighted imaging using newer three-dimensional (3D) GRE sequences (eg, 3D volumetric interpolated breath-hold examination [VIBE], T1-weighted fast acquisition multiple excitation [FAME], or 3D T1-weighted high resolution isotropic volume examination [THRIVE]), combined with gadolinium enhancement, has improved spatial resolution for resolving masses and vascular anatomy. T2-like imaging using breath-hold balanced echo true free-induction with steady-state precession (TFISP) imaging may provide additional information for urographic evaluation of the collecting system, with urine having high signal on such images. Additionally, acquisition of pre- and postcontrast 3D GRE images performed with identical field of view, resolution, and slice parameters allows the precontrast images to be used as a subtraction mask. The resultant image shows only areas of increased signal caused by gadolinium enhancement. This technique may be useful for determining vascularity and tumor within a high-signal protein- or blood-containing renal lesion.
Normal kidney
The advantages of MR multiplanar imaging are exploited by combining axial and coronal imaging, allowing optimal visualization of the renal pelvis and poles ( Fig. 1 ). The use of dynamic gadolinium-enhanced T1 imaging combined with rapid acquisition may be referred to as “functional MR nephrourography” (MRNU).
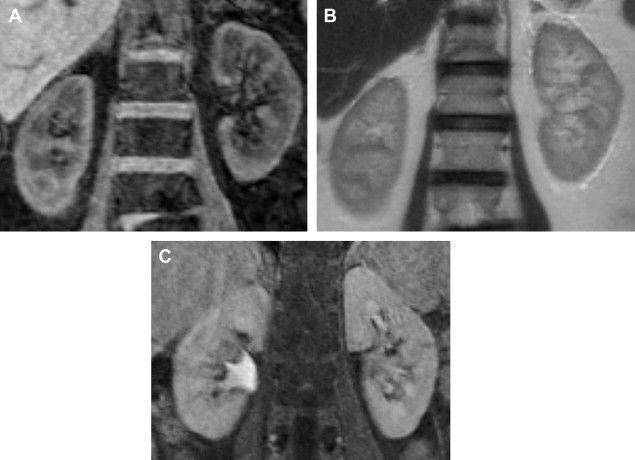
MR nephrourography—structure
In the past, obtaining both structural and functional data of the kidneys has not been possible without compromise involving one or both of these areas. With increasingly rapid image acquisition, however, MRNU offers the ability to capture simultaneously exquisite anatomic detail and physiologic data in parameters that have not been previously possible, even with traditional nuclear medicine techniques.
To obtain structural and functional renal data simultaneously, several imaging sequences are needed. T2-weighted images offer excellent morphologic evaluation of the kidneys and collecting system. The intrinsic signal characteristics of T2-bright urine contrast well with isointense urothelium, allowing the identification of filling defects within the collecting system. Distension of the urinary system with the administration of furosemide has been shown to be helpful, although many clinically significant abnormalities of the collecting system, especially the bladder, often can be identified even with minimal distension because of the intrinsic contrast resolution on T2-weighted sequences. The relative contribution of T2-weighted images may be increased in patients who have significantly impaired renal function, because the visibility of the collecting system does not depend completely on the excretion of contrast material, a requirement of gadolinium-chelate–enhanced imaging.
In addition, with the use of relatively motion-insensitive T2-weighted half-Fourier acquisition single-shot turbo spin-echo (HASTE) sequences, high-quality images of the kidneys can be obtained even in free-breathing patients. Pulse sequences that use steady-state magnetization, such as TFISP, also can demonstrate the morphology of the collecting system in the absence of excretion. Like T2-weighted HASTE sequences, TFISP has excellent in-plane motion insensitivity and may display collecting system morphology in a nondistended system better than single-shot T2-weghted images, although this advantage should be balanced against a drop in contrast resolution compared with T2-weighted sequences. A disadvantage of two-dimensional (2D) T2-weighted images is the inability to reconstruct the images in a volume format. Volume-acquired 3D T2-weighted images, however, may be acquired to generate a maximum-intensity projection (MIP) dataset that can demonstrate collecting system morphology in a rotating manner from multiple-projection reconstructions, similar to the MIP reconstructions often used in vascular imaging. Multiple projection reconstructions provide a powerful anatomic overview of the collecting system helpful for referring clinical services. Disadvantages of the 3D technique are a significant increase in data acquisition time compared with single-slice T2-weighted HASTE and loss of the motion insensitivity inherent in the 2D technique. Respiratory gating with a 2D navigation system can be used but at the expense of further increases in the total acquisition time for all the slices.
3D GRE contrast-enhanced T1-weighted sequences are another mainstay of MR nephrourography. Although T2-weighted images provide excellent contrast resolution and morphologic data, postcontrast 3D GRE images can provide functional data in addition to complementary views of tissue contrast. Precontrast T1-weighted images are ideal for displaying hemorrhage and proteinaceous debris. Dynamic contrast-enhanced images at varying time points allow the identification of neoplastic processes involving either the renal parenchyma or the urothelium. For example, early contrast-enhanced images clearly demonstrate enhancement of the urothelium against a background of dark urine before the excretion of gadolinium. This inherent contrast differential allows identification of enhancing tumors within the renal pelvis, ureters, or bladder, even in the setting of poorly functioning kidneys with diminished excretion. Tumor visibility is enhanced with the routine use of fat suppression, causing the surrounding retroperitoneal fat to become dark. This technique has the effect of allowing enhancing tissues to fill the available gray-scale values, allowing easier depiction of urothelial neoplastic processes ( Fig. 2 ). Use of a 3D volume acquisition with thin partitions allows the initial coronal data to be reformatted into additional imaging planes as desired to enhance lesion detection further. Disadvantages to the 3D T1-weighted technique include sensitivity to motion, which can degrade images significantly in a free-breathing patient. Additional techniques to shorten the acquisition time (eg, increased parallel processing acceleration) can reduce these detrimental effects but at the cost of a decreased signal-to-noise ratio. Contrast enhancement of the collecting system is dependent on renal excretion, which may be reduced markedly with severe renal disease. This problem is not unique to MR imaging, however, and is encountered in both nuclear renal scintigraphy and CT urography. Thus MR imaging provides still another advantage by having alternative contrast strategies using T2-weighted imaging to visualize the collecting system even when the kidney’s excretory function may be severely impaired.
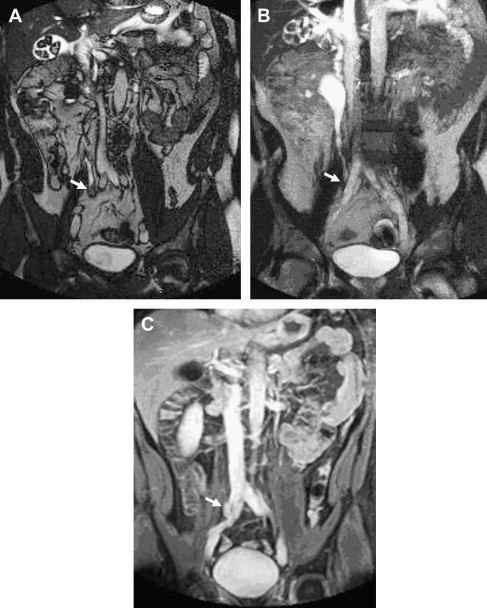
In addition to morphologic imaging, 3D GRE images are the critical sequences necessary for the quantitative evaluation of renal function with MR imaging. Advances in parallel processing and in undersampling and underfilling of k-space have reduced imaging times so that a coronal volume of images through the kidneys can be acquired in 0.9 seconds with these techniques. Repeated T1-weighted GRE acquisitions through the kidneys every second during the administration of intravenous gadolinium allows the calculation of differential glomerular filtration rate (GFR) and renal blood flow (RBF) for each kidney.
MR nephrourography—structure
In the past, obtaining both structural and functional data of the kidneys has not been possible without compromise involving one or both of these areas. With increasingly rapid image acquisition, however, MRNU offers the ability to capture simultaneously exquisite anatomic detail and physiologic data in parameters that have not been previously possible, even with traditional nuclear medicine techniques.
To obtain structural and functional renal data simultaneously, several imaging sequences are needed. T2-weighted images offer excellent morphologic evaluation of the kidneys and collecting system. The intrinsic signal characteristics of T2-bright urine contrast well with isointense urothelium, allowing the identification of filling defects within the collecting system. Distension of the urinary system with the administration of furosemide has been shown to be helpful, although many clinically significant abnormalities of the collecting system, especially the bladder, often can be identified even with minimal distension because of the intrinsic contrast resolution on T2-weighted sequences. The relative contribution of T2-weighted images may be increased in patients who have significantly impaired renal function, because the visibility of the collecting system does not depend completely on the excretion of contrast material, a requirement of gadolinium-chelate–enhanced imaging.
In addition, with the use of relatively motion-insensitive T2-weighted half-Fourier acquisition single-shot turbo spin-echo (HASTE) sequences, high-quality images of the kidneys can be obtained even in free-breathing patients. Pulse sequences that use steady-state magnetization, such as TFISP, also can demonstrate the morphology of the collecting system in the absence of excretion. Like T2-weighted HASTE sequences, TFISP has excellent in-plane motion insensitivity and may display collecting system morphology in a nondistended system better than single-shot T2-weghted images, although this advantage should be balanced against a drop in contrast resolution compared with T2-weighted sequences. A disadvantage of two-dimensional (2D) T2-weighted images is the inability to reconstruct the images in a volume format. Volume-acquired 3D T2-weighted images, however, may be acquired to generate a maximum-intensity projection (MIP) dataset that can demonstrate collecting system morphology in a rotating manner from multiple-projection reconstructions, similar to the MIP reconstructions often used in vascular imaging. Multiple projection reconstructions provide a powerful anatomic overview of the collecting system helpful for referring clinical services. Disadvantages of the 3D technique are a significant increase in data acquisition time compared with single-slice T2-weighted HASTE and loss of the motion insensitivity inherent in the 2D technique. Respiratory gating with a 2D navigation system can be used but at the expense of further increases in the total acquisition time for all the slices.
3D GRE contrast-enhanced T1-weighted sequences are another mainstay of MR nephrourography. Although T2-weighted images provide excellent contrast resolution and morphologic data, postcontrast 3D GRE images can provide functional data in addition to complementary views of tissue contrast. Precontrast T1-weighted images are ideal for displaying hemorrhage and proteinaceous debris. Dynamic contrast-enhanced images at varying time points allow the identification of neoplastic processes involving either the renal parenchyma or the urothelium. For example, early contrast-enhanced images clearly demonstrate enhancement of the urothelium against a background of dark urine before the excretion of gadolinium. This inherent contrast differential allows identification of enhancing tumors within the renal pelvis, ureters, or bladder, even in the setting of poorly functioning kidneys with diminished excretion. Tumor visibility is enhanced with the routine use of fat suppression, causing the surrounding retroperitoneal fat to become dark. This technique has the effect of allowing enhancing tissues to fill the available gray-scale values, allowing easier depiction of urothelial neoplastic processes ( Fig. 2 ). Use of a 3D volume acquisition with thin partitions allows the initial coronal data to be reformatted into additional imaging planes as desired to enhance lesion detection further. Disadvantages to the 3D T1-weighted technique include sensitivity to motion, which can degrade images significantly in a free-breathing patient. Additional techniques to shorten the acquisition time (eg, increased parallel processing acceleration) can reduce these detrimental effects but at the cost of a decreased signal-to-noise ratio. Contrast enhancement of the collecting system is dependent on renal excretion, which may be reduced markedly with severe renal disease. This problem is not unique to MR imaging, however, and is encountered in both nuclear renal scintigraphy and CT urography. Thus MR imaging provides still another advantage by having alternative contrast strategies using T2-weighted imaging to visualize the collecting system even when the kidney’s excretory function may be severely impaired.
In addition to morphologic imaging, 3D GRE images are the critical sequences necessary for the quantitative evaluation of renal function with MR imaging. Advances in parallel processing and in undersampling and underfilling of k-space have reduced imaging times so that a coronal volume of images through the kidneys can be acquired in 0.9 seconds with these techniques. Repeated T1-weighted GRE acquisitions through the kidneys every second during the administration of intravenous gadolinium allows the calculation of differential glomerular filtration rate (GFR) and renal blood flow (RBF) for each kidney.
MR nephrourography—function
The clinical management of patients who have kidney diseases is somewhat limited by the lack of readily available noninvasive methods to test and follow renal function, to diagnose causes of renal dysfunction, or to monitor treatment response. Among the most commonly used tests for evaluation of renal function are measurement of serum creatinine level, endogenous creatinine clearance, and urinalysis for measurement of proteinuria. These indirect measures of renal GFR and loss of filtration integrity are insensitive and nonspecific and do not supply information that would differentiate the right kidney from the left. Accurate 24-hour urine collections are challenging generally, and it is particularly difficult to ensure accuracy in younger patients.
There remains a clinical need for a sensitive and noninvasive in vivo imaging method that can be performed quickly and safely to provide regionally specific functional information about the kidney and to facilitate repeated evaluation, especially when there is a need for monitoring the progression of disease or the response to a treatment. Although nuclear medicine methods such as renal scintigraphy have been used for determining renal function, these techniques suffer from low spatial resolution and do not provide detailed analysis of both structure and function. Furthermore, Technetium-99m mercaptoacetyltriglycine (MAG-3), commonly used in nuclear renal imaging, is an agent actively excreted by the renal tubules, and the rate of excretion does not measure the GFR. The use of radioactive tracers, particularly in monitoring applications where the study will be repeated, raises the concern of radiation risk. This concern is particularly important when dealing with younger or pregnant patients. Dynamic CT imaging has been used, but it also involves undesirable risks from ionizing radiation. In the setting of acute renal disease, or in patients who have risk factors such as diabetes mellitus, CT may be dangerous because it uses potentially nephrotoxic iodinated contrast agents.
Advantages of MR imaging include the ability to achieve scans with higher temporal resolution than obtained with CT and nuclear scans and with higher spatial resolution than obtained with nuclear scans, without the exposure to ionizing radiation that occurs with CT and nuclear scans. Important measures of renal function can be related to RBF and GFR. From these parameters the filtration fraction can be determined. Recently, the potential of gadolinium-enhanced dynamic MR imaging, or MRNU, of the kidney has emerged as having the capacity to measure GFR. This method uses rapid “snapshots” of the kidney at different time points following administration of a gadolinium-chelate paramagnetic contrast agent, combining mathematical modeling of tracer kinetics to determine RBF and GFR. There has been development and evolving validation of gadolinium-chelate perfusion MR imaging techniques for the evaluation of GFR, relying on its behavior as a filtered agent without active excretion or uptake from the renal tubules.
Several methods have been developed for estimating the GFR from dynamic nuclear medicine data, but all are hampered by the poor counting statistics of such dynamic studies and the problem of accounting for the extrarenal component of the signal. Recently, several groups have applied the methods developed for nuclear medicine to dynamic MR imaging data acquired in conjunction with an injection of the contrast agent gadolinium-diethylenetriamine pentaacetic acid. In applying these techniques to MR imaging data, several issues must be addressed. First, although nuclear medicine measures the activity, and hence the concentration, of the contrast agent directly, in MR imaging the contrast agents change signal by altering the relaxation times of the tissue, producing a linear relationship with the concentration over only a limited range of concentrations. Second, the exact relationship between the signal and concentration depends on the flip angle used, and because the flip angle varies across the slice in 2D studies, time-consuming corrections are required for 2D data, making these unsuitable for routine clinical applications. Third, to obtain an adequate signal-to-noise ratio, it generally is necessary to use surface array coils for the reception of the signal, which in turn can lead to local variations in signal intensity that complicate the analysis of the data. One approach that the present authors have advocated addresses these problems by using a slow injection of contrast over 10 seconds to limit the arterial concentration, by using a 3D technique and discarding the outer slices to ensure a uniform flip angle, and by using the precontrast signal to correct for spatial variations in the signal intensity.
Calculation of the individual RBF and GFR from gadolinium-enhanced MRNU can be coupled with measurement of the individual kidney volumes (cortex plus medulla). This technique makes it possible to determine RBF and GFR in proportion to a unit measure of kidney volume that can be expressed, for example, as RBF or GFR per milliliter of kidney. This value may provide an additional functional parameter for monitoring renal dysfunction and response to interventions, which previously was not possible in the clinical setting ( Fig. 3 ). Potential applications range across the full spectrum of renal diseases.
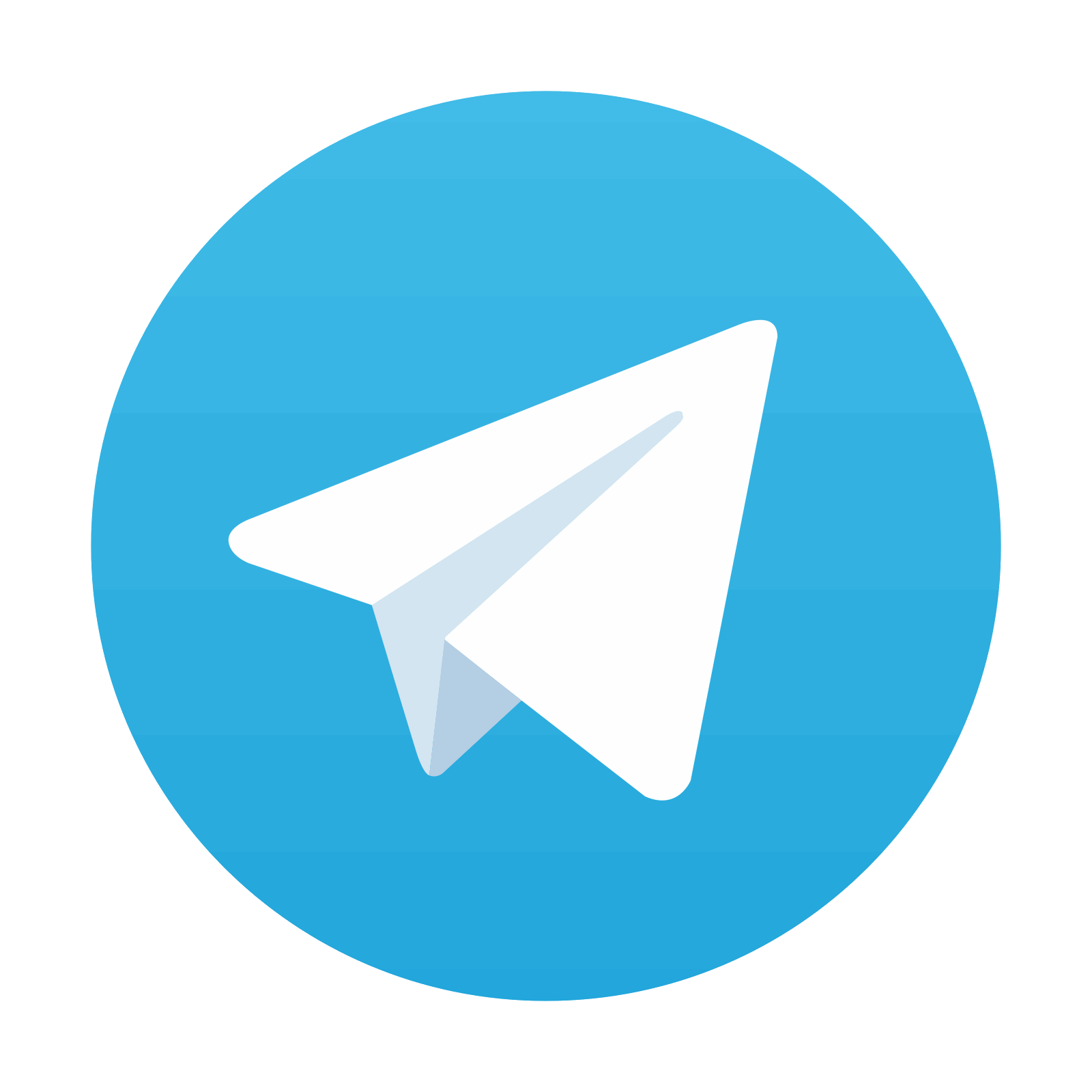
Stay updated, free articles. Join our Telegram channel

Full access? Get Clinical Tree
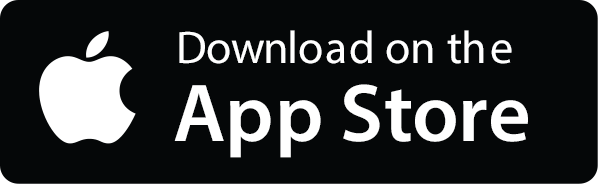
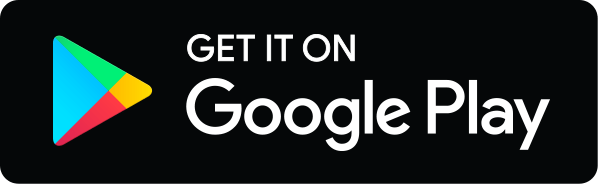
