(1)
Core Electron Microscopy Facility, Department of Cell Biology, University of Massachusetts Medical School, Worcester, MA, USA
Abstract
Metal shadowing of bacteria, viruses, isolated molecules, and macromolecular assemblies is another high-resolution method for observing the ultrastructure of biological specimens. The actual procedure for producing a metal shadow is relatively simple; a heavy metal is evaporated from a source at an oblique angle to the specimen. The metal atoms pile up on the surfaces that face the source, but the surfaces away from the source are shielded and receive little metal deposit, creating a “shadow.” However, the process of producing biological specimens that are suitable for metal shadowing can be very complex. There are a whole host of specimen preparation techniques that can precede metal shadowing, and all provide superior preservation in comparison to air drying, a required step in negative staining procedures. The physical forces present during air drying (i.e., surface tension of the water–air interface) will literally crush most biological specimens as they dry. In this chapter I explain the development of and procedures for the production of biological specimens from macromolecular assemblies (e.g., DNA and RNA), purified isolated molecules (e.g., proteins), and isolated viruses and bacteria preparations suitable for metal shadowing. A variation on this basic technique is to rotate the specimen during the metal deposition to produce a high-resolution three-dimensional rendering of the specimen.
Key words
Electron microscopyMetal shadowingUnidirectional shadowingRotary shadowingPlastic support filmsFormvarMetal evaporationSpreading of virusesBacteriaMacromolecular assemblies1 Introduction
1.1 History
The uses of transmission electron microscopy as applied in materials science progressed fairly rapidly. Because of the stable nature of most specimens (e.g., crystals and metallic films) material science type specimens were relatively easy to adapt to this new science tool. Biologists however encountered a different situation. The first published micrographs of chicken liver tumor by Dr. Ladislaus Laszlo Marton in 1934 [1] were in a word—awful; the material was literally incinerated by the beam. He later published the first electron micrographs of bacteria spread on carbon films demonstrating that biological specimens could be imaged if you used an appropriate preparatory technique.
The problem for biologists trying to use this new and exciting tool was how to prepare soft, wet, and very friable biological materials so they could be observed with an electron source. The answer, at least in part, came with the invention of plastic support films (e.g., Formvar) developed first and presented by Schaeffer Harker in 1942 for use in X-ray crystallography and applied physics [2]. Here again was an invention that was seized upon by the biological researchers as a way to mount and prepare their own delicate specimens.
By early 1942, these new, very thin support films were being used by Biologists, Biophysicist, and Virologists as a means to support, negative stain, observe and study the ultrastructure of microorganisms. Then in 1946 a young researcher, Ralph Walter Graystone Wyckoff, at Ann Arbor Michigan found an unused electron microscope in the basement of the Bacteriology Laboratory and succeeded in taking beautiful pictures of the influenza virus. They were particularly appealing because of the metal shadowing technique that he had just developed, which provided three-dimensional images of the virus. With further improvements on this technique; modern and substantially better apparatus for preparing our specimens, metal shadowing has become a rapid and reliable method to produce very-high-resolution images of many types of biological specimens.
The shadow casting consists of evaporating a metal source at an oblique angle to the specimen. The smaller the objects being shadowed the smaller the angle must be. The effect of the oblique deposition is to deposit metal onto the relatively higher portions of the specimen, thus casting a partial or total shadow in the direction opposite the source. When the metal shadowed specimens are observed in the electron microscope, areas where the metal coating is the thickest will scatter the electron beam creating dark areas while those areas where the metal is thin will be transparent to the beam and appear bright. The images were originally view as photograph negative images where the metalized areas appeared white and the shadows (very thin areas) appeared dark [3]. It was thought that these images conformed more closely to the usual usage of the term shadow. This changed with the invention of Rotary shadowing techniques. Heinmets introduced a variant on the original technique by placing a small electric motor into the vacuum chamber and spinning the specimen during shadowing to coat surface structures with a uniform film of metal on all sides [4]. This still produced a three dimensional image but now it appeared much more vivid if it was viewed as a positive image and so like all transmission electron micrographs, these images are now viewed as positive images rather than as the photographic negative (Fig. 1).
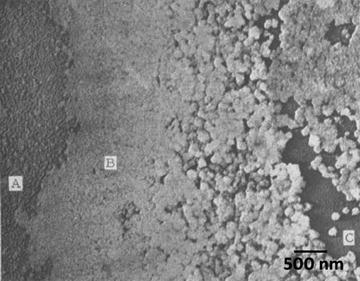
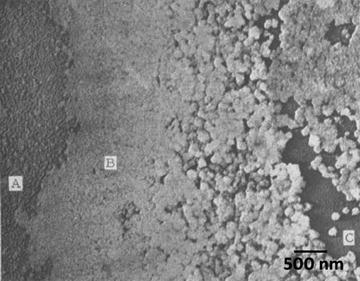
Fig. 1
One of the first images of metal shadowed influenza virus ever recorded. This slightly fuzzy image was published as a photographic negative of the original image. The authors had to produce a photographic positive on film and then print that back as the negative image (a second generation print) to produce the “realistic shadow effect”. Each individual virus particle is approximately 100 nm in diameter. Reproduced from Sharp et al. [3], with the permission of the Journal of Biological Chemistry
While uniform deposition of metal on all sides of a particle provides greater contrast, it also can produce curious artifacts. Neugebauer and Zingsheim observed these artifacts and studied the relationship of these artifacts to the angle of shadowing, and found that it was most pronounced at low shadowing angles [5]. Particles that have a great degree of structural symmetry often appeared to have a donut-shape hole at their centers when viewed from above. A similar problem occurs when looking at filamentous structures when you are examining the “handedness” (i.e., right or left helical twist) as exhibited by strands of protein, DNA, or RNA. The direction of the twist can be completely obscured by the metal coating. With symmetrical particles, the problem can be even more challenging if the particles are expected to have an actual hole in their center, such as connexons of gap junctions, then this artifact becomes very apparent. In 1982, Hirokawa and Heuser confirmed that connexons of gap junctions do indeed have a central tunnel after examining the structure by employing both unidirectional and rotary-shadowing techniques at relatively high angle [6]. This study clearly demonstrated that an understanding of the structure in question is prerequisite to the correct interpretation of the images provided by the electron microscope. Further, a thorough understanding of the specimen preparation techniques plays a key role in the ultrastructural interpretation of your specimens.
Many comparisons of metal shadowing techniques have demonstrated that unidirectional shadowing is superior to rotary shadowing for highlighting membrane topography and for detecting very small intermembrane proteins down to 5 nm [7]. The best use of rotary shadowing appears to be for specimens prepared by specialized techniques that allow one to see the membrane architecture: cytoskeletal filaments connecting organelles, external surfaces of cells (both eukaryotes and prokaryotes), individual macromolecules, and isolated proteins (Fig. 2).
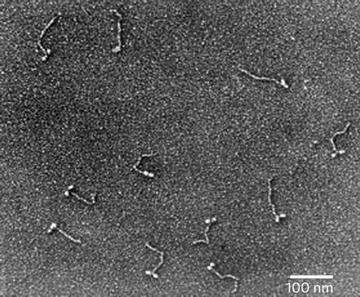
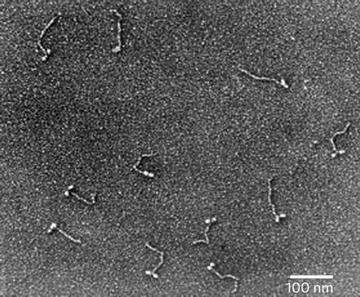
Fig. 2
Metal shadowed isolated chicken gizzard myosin molecules, sprayed onto a freshly cleaved mica sheet and rotary shadowed with platinum–carbon at 6°. The image was recorded at 60 KX. Courtesy of Dr. Roger Craig, University of Massachusetts Medical School
1.2 Requirements for Metal Shadowing
1.
The deposited film must be both chemically and thermally inert [8]. Once the metal film is deposited onto the specimen it must be stable and not subject to oxidation or corrosion.
2.
High density to provide maximum electron scattering for minimal deposit thickness [8].
The metal used to produce the deposited film must be of high enough atomic number (Z number) to ensure sufficient electron scattering to produce the differential contrast needed for good image quality even when deposited in a very thin film (see Table 1) (see Note 1).
Table 1
Common metals and their key characteristic for use in metal shadowing
Element/material | M.P. (°C) | Density (ρ) | Granularity | Evaporation method |
---|---|---|---|---|
C Carbon (graphite) | 3,652 | 2.25 | Extremely fine | Carbon rods or threads |
Cr Chromium | 1,857 | 7.20 | Fine, becomes very coarse as the film oxidizes | Coiled tungsten basket or molybdenum boat |
Au Gold | 1,064.43 | 19.3 | Very coarse | Tungsten filament or carbon rod |
Au–Pd 60:40 Gold–Palladium | 1,465 | 16.38 | Coarse | Tungsten filament or carbon rod |
Pt Platinum | 1,772 | 21.45 | Fine | Tungsten filament or carbon rod |
Pt–Pd 80:20 Platinum–Palladium | 1,760 | 20.64 | Fine | Tungsten filament or carbon rod |
Eu Europium | 822 | 5.24 | Very fine | Tungsten filament or carbon rod |
W Tungsten | 3,410 | 19.35 | Very fine | Tungsten filament |
Ta Tantalum | 2,996 | 16.60 | Very fine | Tungsten filament |
3.
The metal must have the capacity to be deposited in a uniform almost structureless and continuous film [8].
There are many metals that meet this requirement when the film is relatively thick (0.1–0.3 nm), which would be quite adequate for larger specimens like bacteria and nanoparticles. However, when imaging single molecules of proteins and macromolecules like DNA and RNA you would need a very thin continuous film on the order of 0.01–0.03 nm so as not to significantly enlarge or alter the fine structure of the specimen. For these applications the choice of shadowing metal is very important (see Note 2).
4.
The deposited metal film must also resist recrystallization and thermal creep of evaporated metal deposit which would change the apparent specimen structure [8].
The phenomenon of metal creep after evaporation has more to do with the setup of the vacuum evaporator apparatus and the vacuum conditions than the choice metal. Although some metals are more prone to creep and puddling (e.g., pure gold) than others, in most cases the use of alloys (i.e., gold–palladium or platinum–palladium) and working at very high vacuum (10−7 Torr) will prevent thermal creep of the deposited metal film. Modern shadowing techniques utilize a variety of metals and alloys including platinum, gold, palladium, tungsten, tantalum, and europium; see the table list of metals below. Of these metals, the most popularly used for shadowing biological specimens are platinum co-evaporated with carbon or alloyed with palladium, introduced in 1959 by Bradley, and tantalum evaporated on a tungsten filament, introduced in 1962 by Bachmann and Hayek [9, 10]. These two alloys have been found to produce very fine-grain films owing to the fact that recrystallization of the film after evaporation and deposition is minimal. In the case of platinum–carbon co-evaporation technique, the carbon appears to reduce the tendency of the platinum to recrystallization by improving the thermal conductivity of the biological matrix. However, tantalum belongs to the group of refractory metals; loosely defined in metallurgy and material science as a subgroup of elements (e.g., Nb, Mo, Ta, W, and Re) with melting points above 2,000 °C, high hardness at room temperature, chemically inert, and relatively high densities. Their extremely high melting points and stability against creep deformation and their inability to recrystallize make these metals a good choice for high-resolution metal shadowing (see Note 1).
1.3 Preparatory Techniques for Metal Shadowing Biological Specimens
There are a whole host of specimen preparation techniques that can precede metal shadowing, and all provide superior preservation in comparison to air drying, a required step in negative staining procedures. The physical forces present during air drying (i.e., surface tension of the water–air interface) will literally crush most biological specimens as they dry.
1.3.1 Glycerol Spraying/Low-Angle Metal Shadowing Method
Hall introduced a “mica replication” technique where he used freshly cleaved mica substrates, air spraying various macromolecules onto this surface followed by low-angle rotary shadowing to produce very precise metal shadowed renderings of the sample macromolecules [11]. Tyler and Branton reported a significant improvement on this technique by first mixing the macromolecules into a solution of glycerol (40–50 %) and ammonium acetate buffer before air spraying the sample onto freshly cleaved mica sheets [12]. This modification produced a more dispersed spreading and even distribution of the macromolecules on the mica surface. They called this new modification “Glycerol drying”. Specifically, they added glycerol to the sample before spraying it onto the mica and backed the metal replica with a carbon film. The addition of glycerol was first proposed as a cryoprotectant/antifreeze because in their first attempts the macromolecules were sprayed onto mica sheets that were placed onto liquid nitrogen cooled copper blocks. Glycerol spraying/low-angle rotary shadowing has been shown to preserve native conformations comparably to cryo-techniques [13]. However, the glycerol spraying technique works equally well at room temperature because glycerol is actually acting as a wetting agent to preserve the water activity at the molecular level around the molecules until they are dried in the vacuum apparatus.
Vacuum drying of sprayed macromolecules even in a glycerol solution does not completely avoid drying artifacts. Molecules sprayed onto freshly cleaved mica surfaces and visualized by this method are noticeably flattened and extended. However, the results obtained by this method are still far superior to those obtained by air drying alone. Fowler and Aebi [14] suggested that this artifact was due to the impact force with which the macromolecule-containing drops of glycerol hit the mica surface during spraying. The impact velocity is such that the droplets are flattened, then immediately retract, leaving a ring of macromolecules adsorbed to the mica in a thin layer of buffer solution around the central droplet. Once placed in the vacuum the very thin layer of volatile buffer dries “instantaneously,” leaving what has been described as the “halo” around the dried center droplets of glycerol and salt residues from the buffers [14]. However, this same “halo” can be seen around glycerol droplets sprayed directly onto grids prepared with carbon stabilized Formvar support films, but the molecules appear less flattened and extended, even demonstrating folded-to-extended conformational changes in Myosin V molecules [15]. This suggests that the flattening effect is do, at least in part to the highly charged surface of the freshly cleaved mica substrate and not the “instantaneous” drying proposed by Fowler and Aebi [14]. Since that Myosin V study (see Fig. 3) I did here at our laboratory in 2004, I have successfully used this modification on many different macromolecular preps including single strand DNA and RNA, and have had consistently good results.
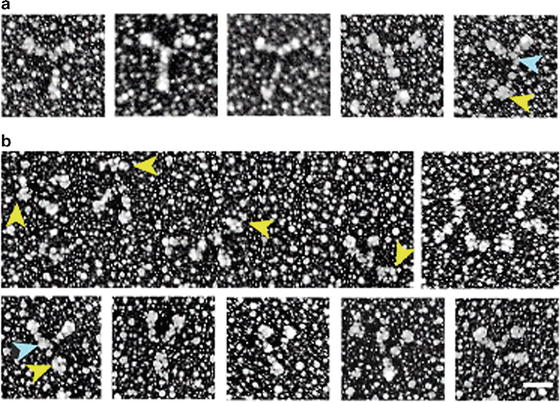
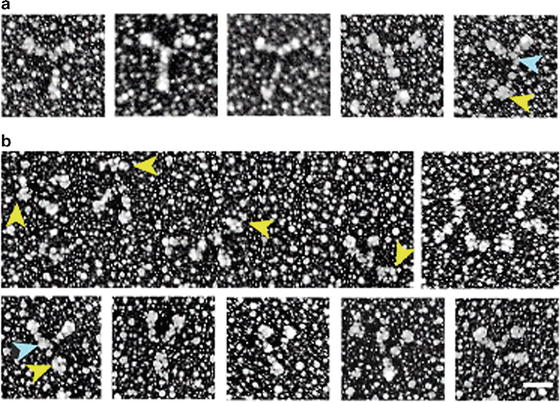
Fig. 3
Myosin V, Glycerol spraying/low-angle rotary shadowing (6°) with platinum–carbon on carbon stabilized Formvar support films. Reproduced from Krementsoy et al. [15], with the permission of Rockfeller University Press
1.3.2 Spontaneous Adsorption Method for DNA and RNA
There have been numerous variations of this technique; all are modifications of the original DNA molecules in protein-mixed films technique introduced by Albrecht Klenschmidt [16]. Using this method of spreading DNA, Klenschmidt demonstrated that the shape and molecular weight of DNA can be obtained by viewing these macromolecules spontaneously adsorbed onto prepared support films from solutions which contained formaldehyde and a critical amount of cytochrome C. The subsequent transfer of the macromolecules to support grids, metal shadowing, and imaging in the electron microscope revealed individual molecules suitable for quantitative evaluation.
1.3.3 Mica Sandwich Technique for Metal Shadowing
The mica sandwich technique was introduced by A. Paul Mould et al. as an alternative to glycerol spraying to reduce the shearing forces on the macromolecules during the spray application [17]. Their sandwich method suggested that by replacing glycerol spraying by simply placing a few drops of your sample suspension in 40–70 % glycerol between two sheets of freshly cleaved mica, provided a better way of adsorbing macromolecular assemblies, intact onto the mica substrate. The technique is very simple and effective for larger and more shear sensitive material than conventional spraying. Placing a few drops of your suspension of macromolecules onto the freshly cleaved mica surface and then re-opposing the other sheet on top causes the suspension to be applied evenly to both surfaces by capillary action. The macromolecules are absorbed onto the mica surfaces, and the two pieces are then separated and dried in the vacuum apparatus at 10−6 Torr with the glycerol layer uppermost and rotary shadowed. Mould and his colleagues were able to demonstrate that macromolecular filaments of F-actin and even collagen could be spread and metal shadowed without the inevitable shortening of these long filaments observed when these same preparations were glycerol sprayed [17].
1.3.4 Spreading of Viruses and Bacteria for Metal Shadowing
The first demonstrated and still one of the most common use of metal shadowing is the technique of spreading purified bacteria and virus specimens onto support films to obtain information about the surface structural details. As Cynthia Goldsmith and Sara Miller point out in their Clinical Microbiology Review [18], “Electron microscopy (EM) has long been used in the discovery and description of viruses.” The main advantages of using electron microscopy for viral and bacterial diagnosis is that it is very rapid and does not require organism specific reagents to recognize the pathogenic agent and very little sample is needed to produce EM specimens for analysis. As they suggested in their review, electron microscopy offers the investigator an unbiased, candid view of whatever might be present, while molecular tests require forehand knowledge of the potential infective agent.
The information gained through metal shadowing of the surface structural details can give the investigator an insight into the mechanism of action or infection of the organism. Even in the age of molecular diagnostics and PCR, electron microscopy is a mainstay in detecting newly immerging infectious agents. Examples of this can be seen worldwide: the discovery of Norovirus in 1972 in a preschool outside of Norwalk, OH, [19]; the detection of causative agent in Legionnaires’ disease was first described in July 1976 when an outbreak of a particularly lethal form of pneumonia that resembled acute influenza struck at a convention of the American Legion—it was found to be a bacterium, Legionella pneumophila [20]; and the corona virus outbreak in China in 2002 that led to the first description of Severe Acute Respiratory Syndrome (SARS) [21]. Exotic infections in animals such as the outbreak of an Ebola virus infection of a monkey colony in Reston, VA, in 1989, recognized as being caused by a filovirus, have also been identified by electron microscopy [22].
2 Materials
2.1 Generic Supplies Needed
Listed below are the generic supplies that you will need for all of the preparatory techniques that are discussed in this chapter. You will also need to acquire the specific materials listed under each of the various titled procedures that follow.
1.
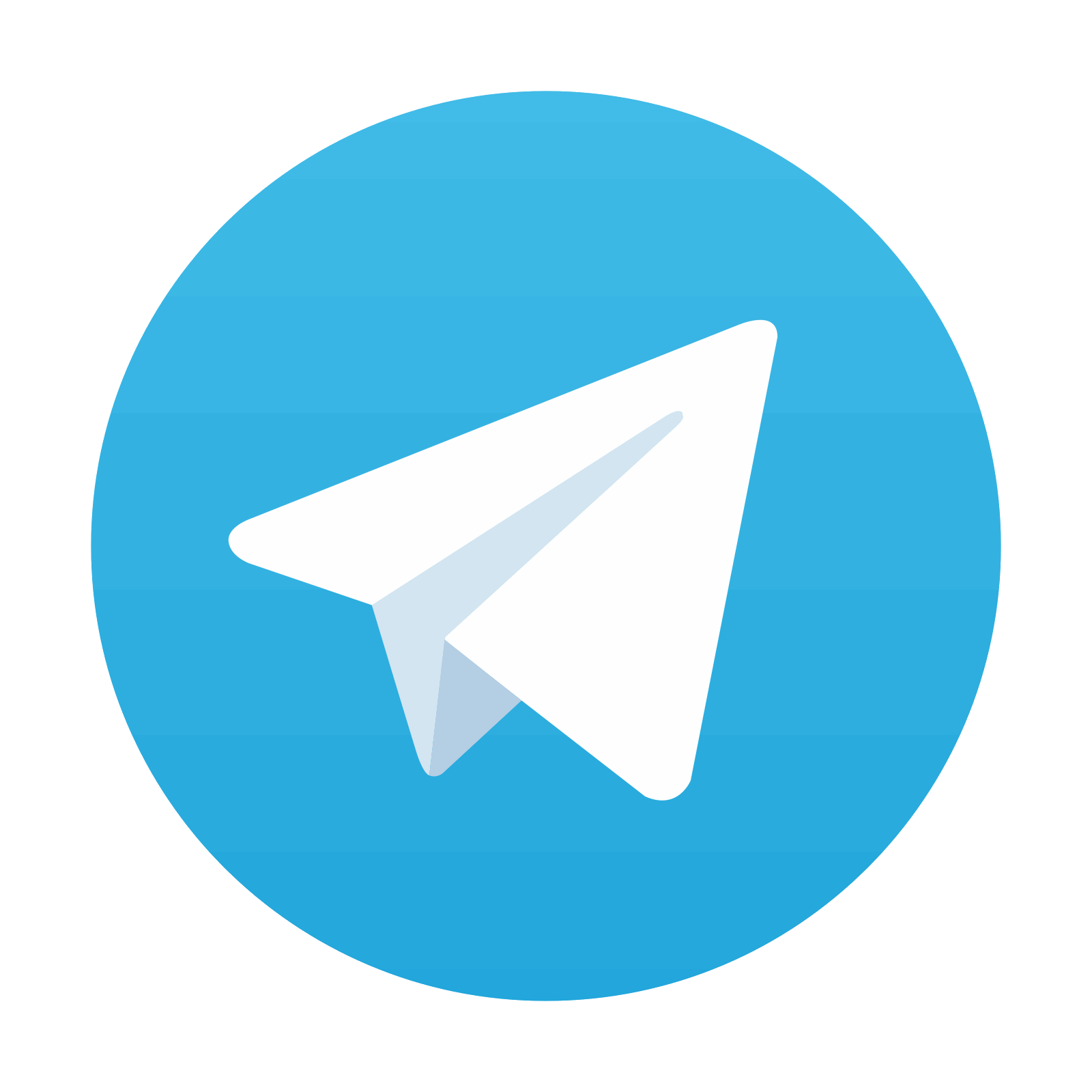
All protocols assume the use of a Denton 502B vacuum evaporator or other manufacturer’s unit for carbon coating and metal shadowing.
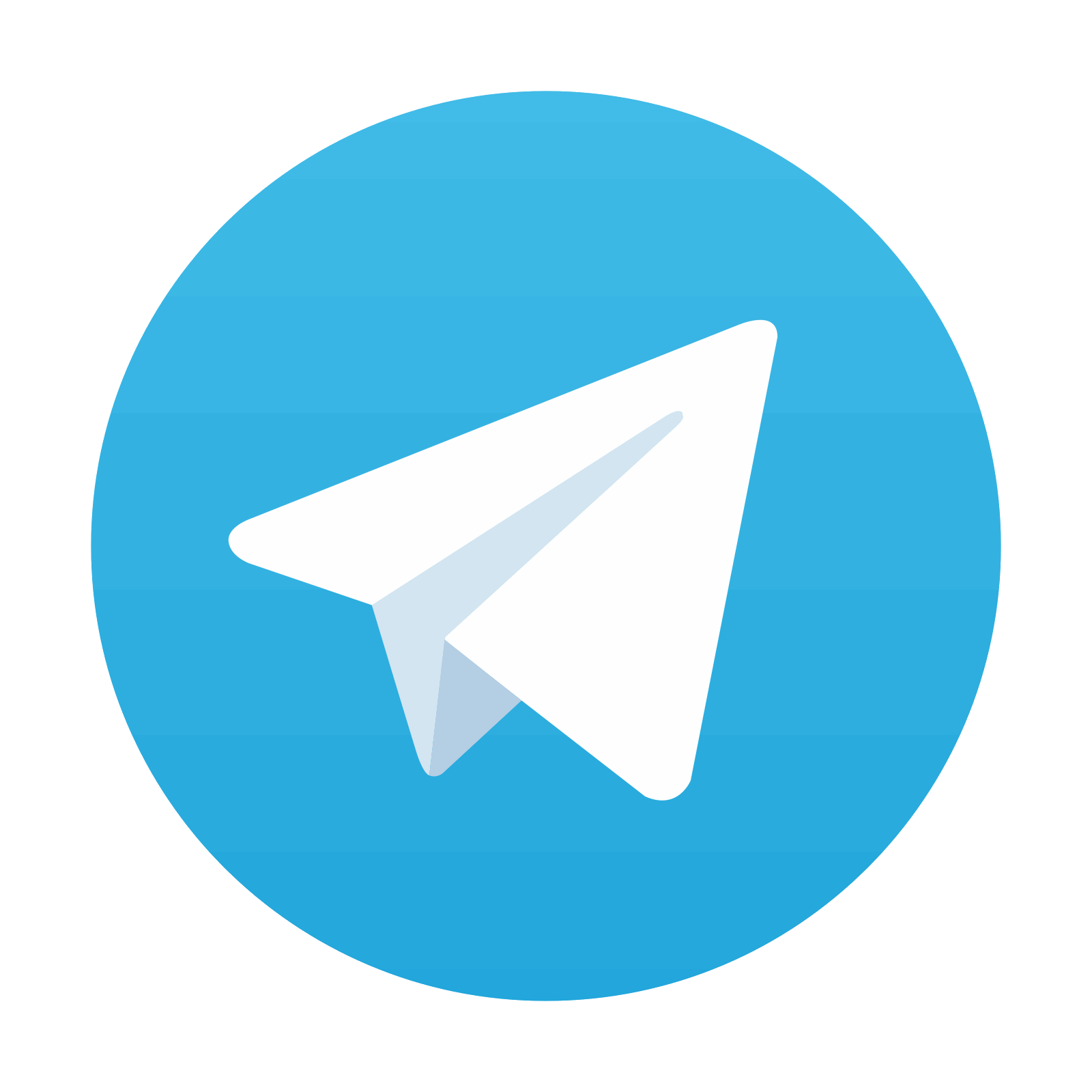
Stay updated, free articles. Join our Telegram channel

Full access? Get Clinical Tree
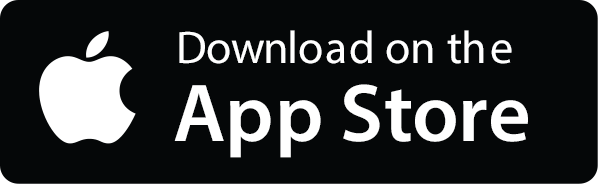
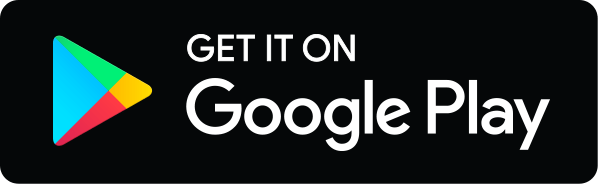
