, Leonid Tiutin2 and Thomas Schwarz3
(1)
Russian Research Center for Radiology and Surgery, St. Petersburg, Russia
(2)
Department of Radiology and Nuclear Medicine, Russian Research Center for Radiology, St. Petersburg, Russia
(3)
Department of Nuclear Medicine Division of Radiology, Medical University Graz, Graz, Austria
Abstract
One of the crucial conditions for a successful PET examination is precise knowledge of indications, counterindications, limitations and capacities of the method as well as correspondence between the approaches opted for and the goals and objectives of the examination.
One of the crucial conditions for a successful PET examination is precise knowledge of indications, counterindications, limitations and capacities of the method as well as correspondence between the approaches opted for and the goals and objectives of the examination.
2.1 Main Tasks, Indications, Counterindications and Limitations of the PET Method
The PET method makes it possible to solve a number of tasks in oncology, such as:
1.
Diagnosis and differential diagnosis of tumors of different localization
2.
Determining the diffusion of the tumor
3.
Determining the degree of malignancy of neoplasms
4.
Evaluating tumor response to treatment, including differential diagnosis of cicatricial changes and continued growth of tumors
5.
Revealing the continued growth and relapses of neoplasms
It should be stressed that the main radiopharmaceutical (RP) used in oncology is still 18F-fluoro-desoxyglucose (18F-FDG). The indications for using 18F-FDG-PET in oncological patients are: evaluating the efficiency of treatment of malignant neoplasms, determining their dissemination (staging), differentially diagnosis of tumors as opposed to benign and non-tumor processes and to cicatricial changes.
By means of 18F-FDG-PET, malignant tumors of any localization can be revealed, but not all kinds of malignant neoplasms have high glycolysis. One of the first relevant meta analysis of papers concerned with 18F-FDG-PET indications for oncology resulted in the Consensus Paper “Onko PET III” in 2002. For example, neoplasms of neuroendocrine nature, neoplasms in the kidneys, prostate, stomach, and gastrointestinal tumors may have a relatively low glycolysis levels. This is why these malignant tumors are not visualized when FDG-PET is used. Among diagnostic errors (false-negative results) examples are attempts to visualize neoplasms less than 4–6 mm large (that is less than the resolving capacity of the scanner), and using 18F-FDG-PET with patients suffering from disturbed glucose utilization and elimination.
Focal uptake of 18F-FDG is observed in areas of inflammation (including tuberculosis, sarcoidosis, aspergillosis, pneumonias), in cases of muscle hypertonicity, in gastric motor hyperactivity, in brown fat uptake, and in disturbances of the passage of urine through the ureter, all of which can induce falsely positive conclusions regarding the tumorous nature of the visualized foci (Ruhlmann et al. 1999; Valk 2006; Khmelev et al. 2004; Phelps 2004).
The indications and limitations of the PET method are described in more detail in the corresponding clinical sections of this book. Counterindications to PET examinations are pregnancy and breast-feeding.
2.2 Short Description of PET Methodology
PET examinations are subdivided into static and dynamic ones. Among static examinations, whole-body examination is the distinguished method.
The PET examination procedure includes several obligatory stages: dosing and injecting RPs, positioning the patient in the gantry, transmission scanning and emission scanning. The stage sequence depends on the kind of examination and on the tasks set for it (Ruhlmann et al. 1999; Valk 2006; Khmelev 2004).
2.2.1 Methodology of PET Examination of the Brain
PET examination of the brain, compared with other organs, has some distinctions due to the possibility of simple and reliable fixation and precise reproduction of the head position, as well as to the necessity of the highest resolution when examining the brain.
Brain scanning usually includes four main stages:
1.
Positioning the patient with head layout for the subsequent coregistration of transmission and emission scans.
2.
Transmission scanning (10 min) using calibration sources of ionizing radiation (68Ge) or CT.
3.
Prepacking and injecting the RP.
4.
Dynamic or static emission scans with subsequent reconstruction of the image.
The patient is positioned with the help of a laser optic centralizer. The orbitomeatal line (OML) usually serves as point of reference. The distance between the OML and the lower line of the centralizer may be different and depends on the axial dimension of the field of vision of the given tomograph and on the localization of the lesion area, which is determined previously by CT or MRI data. The standard position supposes that the lower line of the centralizer is pointed 2 cm below the OML (Fig. 2.1). The requirements of patient positioning are due to the limitation of the axial dimension of the field of vision in most scanners of 15–16 cm, whereas the vertical (frontal) dimension of brain is 14–15 cm. When neurooncological patients are examined, the diagnostician has often to choose between the necessity of scanning all the brain segments and scanning the neoplasm that exceeds the limits of the field of vision of the tomograph in the case of standard patient positioning. As a rule, the choice is made in favor of scanning the neoplasm.
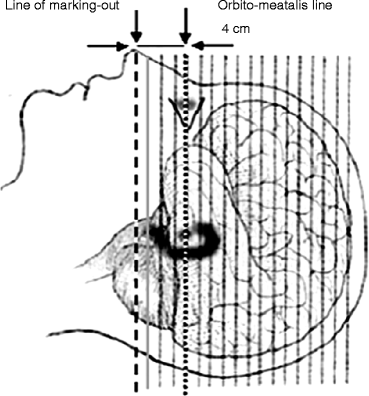
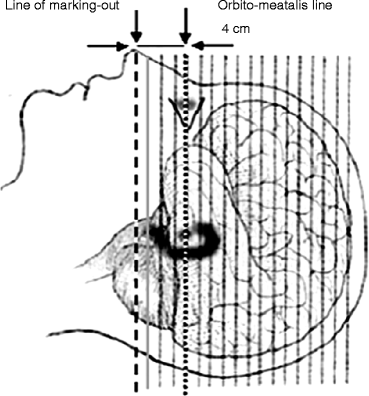
Fig. 2.1
Standard layout and patient positioning in brain examination
Before the emission scan, a 10-min transmission scan is done with calibration sources of ionizing radiation (68Ge), or CT for attenuation correction. The effective dose in this case amounts to 0.07 mSv (for 68Ge) for 10 min. Thirty to forty minutes before and in the course of 10–15 min after the injection of 18F-FDG, the patient is light- and sound-proofed in order to prevent physiological RP uptake in the optic cortex. 18F-FDG is introduced as an intravenous jet into a peripheric vein of the limbs in the volume of 0.5–1.0 mL 35–40 min before the onset of the static emission scanning. The diagnostic dose of 18F-FDG when examining the brain is 100 MBq/m2 of the body surface (or in total 150–250 MBq for an examination). The coefficient for calculating the effective dose in 18 F-FDG introduction is 0.019 MSv/MBq. When introducing the indicated diagnostic dose of RP, the effective dose is 2.85–4. 75 for an examination. After RP introduction, the syringe is washed through with 5.0 mL physiological saline to ensure the maximum complete introduction of the required dose.
The static scan of the brain (20 min long) is carried out in 2D or 3D regimes with use of a 128 × 128 matrix and 1.5-times image enlargement. If quantitative analysis is needed, a dynamic scan is done, the conditions being the same as in static scanning. Dynamic scanning begins immediately after the injection of the RP and lasts 50 min.
To reduce the radiation load the urinary elimination of the tracer can be forced by additional hydration of patients following given Standardised Operating Procedures.
Sinograms are reconstructed by way of forward back projection with use of a Hanning filter and 2.5-times image zooming.
The characteristics of dosing and injecting other RPs are described in particular sections of the present handbook.
Transmission scanning of the brain can be done also after the introduction of the RP; for example, after the emission scanning. Such a sequence is called hot transmission scan. The necessity to make such an examination may be due to the patient’s state of health. This sequence is used in the whole-body examination and in combined PET-MRI. However, it should be noted that in using “hot transmission” the radioactivity diffusion in the section may be distorted.
For image standardization and removal of symmetry disturbances in patient positioning it is accepted to do rotation of the obtained axial image in three projections sequentially, satisfying the following requirements, which are obligatory in brain examinations:
The interlobar fissure in transaxial sections should be parallel to the vertical axis of the screen.
The basal segments of the temporal lobes in coronal sections are parallel to its horizontal axis.
The poles of the frontal and occipital lobes in the midline sagittal section are parallel to the horizontal axis.
It is accepted to keep transaxial cuts 5 mm thick, containing the whole image of the brain, so that both convexital and basal brain segments may be represented, including the cerebellum. The indicated thickness and sequence of obtaining makes it possible to confront scintigraphic brain images with stereotaxic atlases for subsequent localization of pathological formations.
PET images are interpreted according to visual and quantitative criteria. The visual analysis of images is done with use of a color scale (RGB), permitting the intensity of RP uptake in the brain to be determined, and in the areas of interest or in foci, their localization, dimensions, contours and the diffusion of the RP in the focus. PET images are compared with the results of MRI (or CT), as a rule by way of simple visual confrontation. In cases when the formation is not visualized in PET images or is not completely visualized, the criterion of choosing a region of interest in PET images is provided by MRI or CT data which are transferred and superposed onto the PET image manually or electronically. In order to perform image fusion, the method of hard- or soft-image transformation is used (Hasegawa et al. 2002).
At the second stage, the semiquantitative analysis of the image in terms of absolute and relative values is used. The so-called asymmetry index (AI) serves as a semiquantitative criterion in brain examination; it represents the ratio of radioactivity accumulated in the most active high-density area of the tumor to the cortex known to be unchanged (the region of reference).
In diffuse lesions of organs such an approach is impossible due to the absence of reference regions, therefore mathematical models have to be used. Mathematical models suppose the dynamic type of brain scanning for calculating the input function, which is described by the curve activity/time of uptake/ejection of radioactivity in blood. In order to evaluate the level of radioactivity in the blood, it is more convenient to do measurements at the left-ventricular cavity of the left ventricle. For this reason, mathematical models are more widely used in cardiology (Roelcke and Leenders 2001; Valk 2006; Khmelev et al. 2004; Phelps 2004).
Since recently in semiquantitative analysis of the brain, a relative indicator – the standard uptake value (SUV) – has been used. SUV is a dimensionless value, defined as the ratio of specific radioactivity in the measured region (kBq/cm3) to the amount of the introduced radioactivity per body mass (MBq/kg) (Ruhlmann et al. 1999).
SUV is automatically calculated by the software of the PET scanner during image reconstruction, with account taken of the physical half-life period of the radionuclide. When analyzing data with use of the SUV, one should remember that inaccurate information on the weight and stature of the patient, the amount of the introduced radioactivity and the time of its introduction, as well as physical “drift” of the detectors, may induce grave errors. That is why it is accepted to consider as significant a change in SUV during treatment exceeding by 25% its initial value.
The characteristics of methodology, principles of drawing and analyzing conclusions in neurooncological, neurological and psychoneurological patients are described in more detail in the corresponding clinical sections of this book.
2.2.2 Methodology of the Whole-Body PET Examination with 18F-FDG
The methodology of the sequential static scans of several regions (beds) of the measured object with uniting them into one image has been called whole-body examination.
The procedure of the whole-body PET examination includes three stages: the introduction of RP, patient positioning, and transmission and emission scanning, which can be carried out in different orders: sequentially or alternately. As a rule, alternate scanning is used since it permits the reduction of inaccuracy due to the patient’s displacement.
Preparation for the examination. The examination is done on an empty stomach (6-h fast). If the examination is planned for the afternoon, the patient is allowed to have an early breakfast (before 8:00 a.m.) without carbohydrates. If the anamnesis contains indications of diabetes, it is necessary to measure the level of sugar in blood before the examination in order to preclude hyperglycemia. The patient should be in a comfortable position with their eyes closed no less than 20–30 min before and 15 min after the injection of 18F-FDG in order to preclude hypermetabolism of glucose in muscles; in case of examination of the neck, it is recommended to inject 1.0 mL phenazepam 30 min before the introduction of the RP in order to avoid artifacts due to muscular hypermotor activity. In order to reduce the myocardial uptake of RP, lypolysis stimulation is done, for which caffeine-containing products are recommended (black coffee without sugar). For the purpose of reducing background activity, water load is applied, for which the patient should drink no less than 0.7 L water. When examining the region of the pelvis minor, scanning should be begun with organs of pelvis minor immediately after bladder emptying. When visualizing tumors of the abdominal cavity, intravenous injection of a spasmolytic is indicated in order to avoid artifacts due to spasms of smooth muscles of the intestines. In cold seasons, the patient bed is previously warmed with an electrical rug.
Methodology of the examination. In whole-body examination, 18F-FDG is slowly introduced intravenously in a volume of no less than 5–10 mL physiological saline at 200–220 MBq/m2 of the body surface. The diagnostic dose of RP in whole-body examination amounts to 300–500 MBq. The effective dose when injecting the diagnostic dose is 8.1–13.5 mSv. It is suitable to introduce the RP into the peripheric vein of a limb to rule out artifacts in the image due to the RP remaining in the site of injection. The optimum time to begin scanning varies between 60 and 120 min after the introduction of 18F-FDG. Such a wide range of time of starting scanning after the introduction of RP is due to the state of the patient’s metabolism and to concomitant diseases. For example, in the case of a patient below 30 years old without a concomitant pathology, scanning may start already 45–60 min after the injection of RP; whereas for a patient 60–80 years old with atherosclerosis, the examination is recommended no earlier than 90–120 min after the injection (Jadvar et al. 2003).
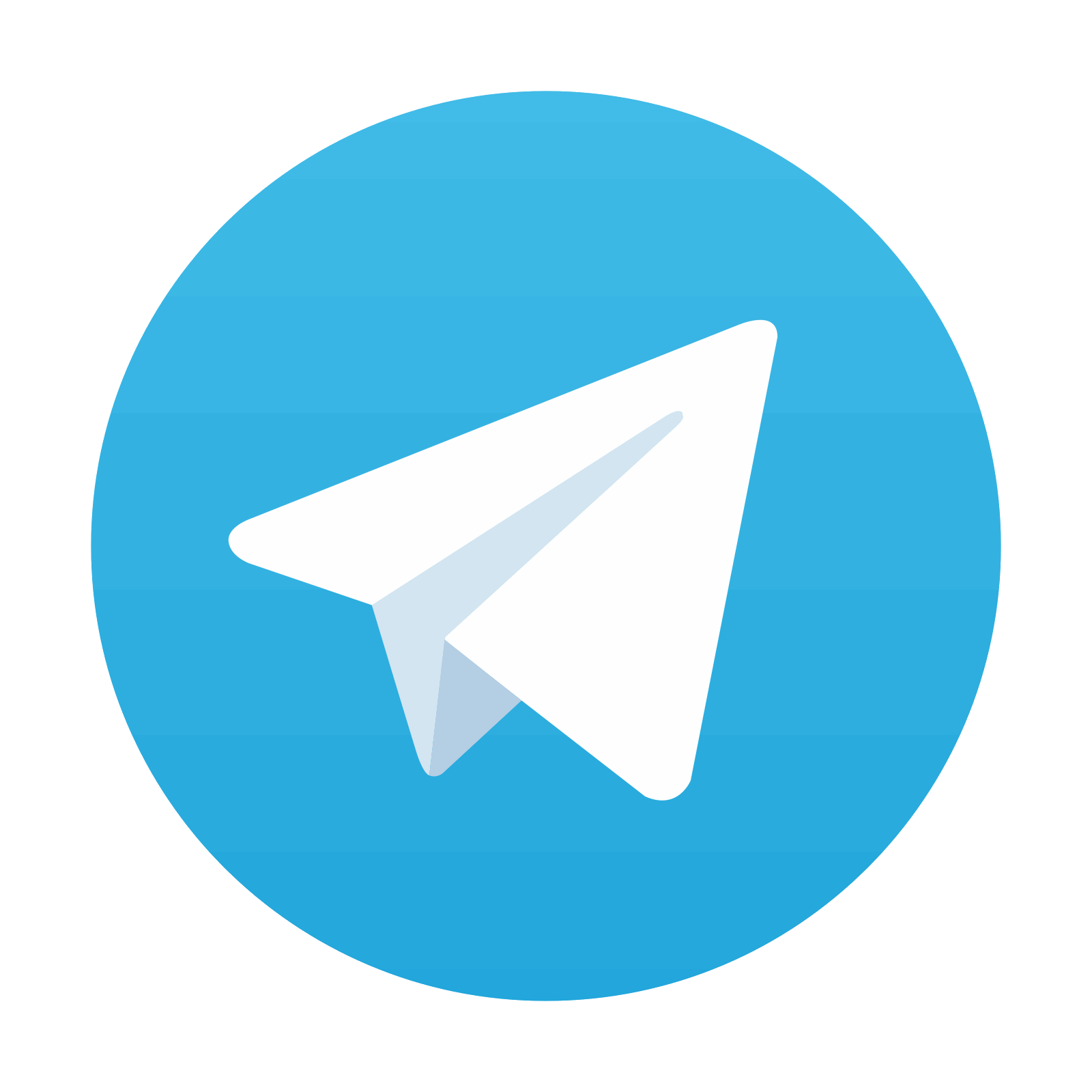
Stay updated, free articles. Join our Telegram channel

Full access? Get Clinical Tree
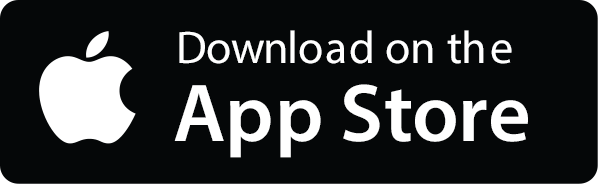
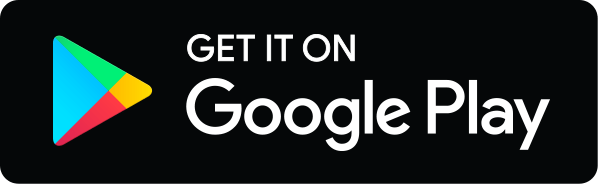