Fig. 6.1
Properties of a FRET VSD dye pair. (a) Most FRET VSDs incorporate an immobile donor (purple) and a mobile anion (orange). A variety of molecules can be used for this purpose. Oxonol derivatives are a versatile choice for the mobile anion. When the donor fluorophore is excited (purple arrow), it will either emit blue photons when the mobile anion is near the intracellular surface of the membrane (blue arrow in the right diagram) or produce FRET when the mobile ion is near the extracellular surface (orange arrow in the left diagram). (b) The structure of a FRET donor, CC1-DMPE, a coumarin-labelled phospholipid. (c) The excitation and emission spectra for the CC2-DMPE and DiSBAC4(3) dye pair. The overlap between donor emission and acceptor excitation determines the FRET efficiency; the more complete the overlap, the more efficient is FRET. The emission spectra for these two dyes are well separated, making them a useful dye pair. (d) Emission spectra of the two dyes as DiSBAC4(3) was loaded into cell membranes. Increased concentrations of DiSBAC4(3) progressively quenched the CC2-DMPE emission. Panels (a) and (b) are reproduced with permission from Gonzalez and Tsien 1997, and panels (c) and (d) are reproduced with permission from Gonzalez and Maher 2002
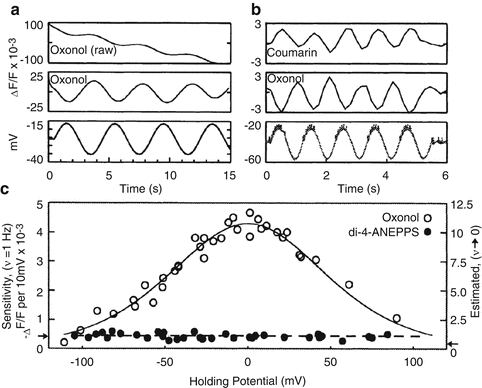
Fig. 6.2
Voltage dependent signals of FRET VSDs. (a) Leech neurons were voltage clamped and the membrane potentials was sinusoidally varied about a holding potential (bottom trace). The raw oxonol trace (top trace) was filtered (middle trace) to remove the slow bleaching artifact visible in the top trace. (b) Sequential recordings of the coumarin and oxonol emission wavelengths to the same sinusoidally varying intracellular stimulus demonstrating that, because the two dyes produce out-of-phase signals, the sensitivity can be enhanced by measuring the ratio of the two signals. (c) The sensitivity of the FRET signal is a function of the membrane potential measured at various holding potentials. The data are well fit by the expected redistribution of oxonol across the membrane during changes in membrane potential (open circles). FRET VSDs in leech ganglia are significantly more sensitive than other VSDs such as di-4-ANEPPS (filled circles). Panels reproduced with permission from Cacciatore et al. 1999
FRET-based dyes are sometimes called ‘slow’ dyes, which is true relative to electrochromic dyes that have typical response times of a few μs (Ebner and Chen 1995; Baker et al. 2005), but FRET dye time constants actually span a large range, from 400 μs to 500 ms, depending on the properties of the mobile anion. While not the fastest, FRET dyes have yielded some of the largest observed fractional fluorescence changes, ranging from 10 to 20 % per 100 mV in intact tissue (Cacciatore et al. 1999) to 100–300 % per 100 mV in isolated cells (Gonzalez and Maher 2002).
Following a brief account of the development of FRET VSDs, we discuss their temporal resolution, sensitivity, and phototoxicity. We then present examples of how these dyes have been used to image neurons. Finally, we provide detailed staining protocols as a reference and starting points for use in other systems.
2 Development of FRET Dye Pairs
Gonzalez and Tsien were first to image voltage-dependent responses from a FRET-based VSD (Gonzalez and Tsien 1995). They used negatively charged hydrophobic oxonol derivatives, bis-(1,3-dialkyl-2-thiobarbiturate)-trimethineoxonol, (DiSBACx(3), where x refers to the number of carbons on the alkyl group and three refers to the number of polymethine carbons), as the mobile voltage sensor. Negatively charged oxonol molecules such as DiSBAC2(3) and bis-(1,3-dibutylbarbituric acid) trimethine oxonol [DiBAC4(3)] have previously been used as redistribution VSDs (Rink et al. 1980; Ebner and Chen 1995). They move between intracellular and extracellular compartments in response to membrane potential changes with very slow kinetics (seconds to minutes). An impermeant oxonol has also been used as a fast absorption dye (Grinvald et al. 1981; Wu et al. 1994a; Momose-Sato et al. 1999). Fluorescence changes result from the fact that oxonols are non-fluorescent in water but are highly fluorescent in hydrophobic environments such as membranes. An anion was chosen, rather than a cation, to take advantage of the dipole potential generated from the polar lipid head-groups, presumably from the ester carbonyl groups, which greatly speeds anion translocation. The rate differences for isostructural borates and phosphonium ions are orders of magnitude faster for the negatively charged borates (Flewelling and Hubbell 1986). Although the mobile anion can in principle serve as either the FRET donor or acceptor, it is most commonly used as the FRET acceptor. As discussed below, there are theoretical and practical advantages for the reverse configuration. The FRET donor was initially chosen to be fluorescein-labeled wheat germ agglutinin (FL-WGA) that bound to N-acetylglucosamine groups on the extracellular face of plasma membranes (Gonzalez and Tsien 1995). The same study showed that using longer chain alkyl groups not only increased the oxonol hydrophobicity but also resulted in faster membrane translocation speed, which improved the FRET temporal resolution, presumably by burying the oxonol deeper into the low dielectric region of the membrane. The optimal chain length was six and DiSBAC6(3) had a translocation time constant ~2 ms.
The original dye pair was improved by replacing FL-WGA with a fluorescent phospholipid, N-(6-chloro-7-hydroxy-2-oxo-2H-l-benzopyran-3-carboxamidoacetyl)-dimyristoylphosphatidylethanolamine [CC1-DMPE] (Gonzalez and Tsien 1997) (Fig. 6.1b). A second chlorocoumarin phospholipid CC2-DMPE is commercially available from Life Technologies (formerly Invitrogen); it has similar properties to CC1-DMPE. The coumarin has a high quantum yield (indistinguishable from 1) and makes an excellent FRET partner with trimethine thiobarbiturate oxonols. The fluorescence emission maxima are separated by 100 nm (Fig. 6.1c), thus enabling efficient collection of both donor and acceptor photons. Another key property of chlorocoumarin phospholipid donors is that they have two negative charges at physiological pH. This is important because it anchors the fluorescent head-group at the extracellular surface and prevents probe translocation across the plasma membrane which would greatly degrade the voltage-sensitive FRET signal. In addition to the phosphate charge, the chloro-group reduces the pKa of the hydroxy coumarin (Fig. 6.1b) to ~5, which results in a second negative charge at physiological pH. A pyrene phospholipid has also been developed as a pH insensitive FRET donor that is compatible with low pH conditions used to activate acid sensitive channels (Maher et al. 2007). The use of a phospholipid placed the donor fluorophore closer to the membrane-water interface, thereby decreasing the minimal distance between donor and acceptor, which may account for the increased voltage sensitivity with the fluorescent impermeant phospholipid (Gonzalez and Tsien 1997). In addition to the original oxonol dye DiSBAC6(3), longer wavelength pentamethine oxonols were developed (the oxonol shown in Fig. 6.1a has n = 2) (Gonzalez and Tsien 1997). Increasing the polymethine chain length from trimethine oxonol [DiSBAC6(3)] to pentamethine oxonol [DiSBAC6(5)] increases charge delocalization, thereby further lowering the activation energy for translocation. This change yielded a time constant for DiSBAC6(5) of ~0.4 ms.
The FRET-based VSD strategy has more recently been used in hybrid voltage sensor systems, incorporating fast anions, such as dipicrylamine (DPA) or DiBAC4(5), with a genetically expressed donor fluorophore such as farnesylated enhanced GFP (eGFP-F) (Chanda et al. 2005). DPA is an absorption dye that does not fluoresce; it serves only to quench the fluorescence of the donor molecule when FRET occurs. The structure of the donor fluorophore has been additionally modified to be able to record action potentials (DiFranco et al. 2007; Sjulson and Miesenbock 2008).
Together these developments and optimizations of FRET-based VSDs demonstrate the versatility in choosing the donor and acceptor for a variety of applications. Most importantly, the source of the voltage sensitivity is well understood making the rational design of future improvements possible.
3 Response Time of FRET Indicators
Because the lifetime of FRET is short, on the order of nanoseconds, the time constant of FRET VSDs is determined by the rate that the hydrophobic anion equilibrates across the membrane when the membrane potential changes (Gonzalez and Tsien 1995). As mentioned above, increasing the length of the alkyl side chains of oxonol increases hydrophobicity and therefore increases its response speed. For example, DiSBAC2(3) and DiSBAC6(3) have time constants of 500 and 2 ms, respectively (Gonzalez and Maher 2002). However, increased hydrophobicity comes at the cost of reduced aqueous solubility, limiting loading concentrations (Table 6.1). Using pluronic F-127 and ß-cyclodextrin brings the more hydrophobic oxonols into contact with cells, which helps to load these dyes. The optimal loading concentration for any FRET acceptor should be high enough to quench a large fraction of donor fluorescence (Fig. 6.1d), to be sure that the acceptor is in the same membrane as the donor, but not so high that it adds a large capacitance to the cell membranes. In practice, loading concentrations for DiSBAC2(3) and DiSBAC4(3) oxonol should not exceed 20 μM (Cacciatore et al. 1999), although the effect of voltage sensitive anions on membrane capacitance should always be assayed with intracellular electrical recordings when applied to a new preparation. For example, concentrations of DPA above 2 μM inhibit action potentials in Drosophila neurons (Sjulson and Miesenbock 2008).
Table 6.1
Oxonol derivatives that can be used as the mobile anion and their associated time constants and measured sensitivities
Commerc. available | Pluronic loading | λex (nm) | λem (nm) | Tc (ms) | Vm sensitivity % ΔR per mV | |
---|---|---|---|---|---|---|
DiSBAC2(3) | Yes | Optional | 540 | 560 | 500 | 1–3 |
DiSBAC4(3) | Yes | Suggested | 540 | 560 | 20 | 0.6–1 |
DiSBAC6(3) | No | Required | 540 | 560 | 2 | 0.4–0.8 |
DiSBAC2(5) | No | Optional | 640 | 660 | 50 | 0.5–2a |
DiSBAC4(5) | No | Suggested | 640 | 660 | 2 | <0.4a |
DiSBAC6(5) | No | Required | 640 | 660 | 0.40 | <0.2a |
Increasing charge delocalization by changing the polymethine chain length of oxonol from trimethine to pentamethine increases the translocation speed 2- to 20-fold (Table 6.1). DiSBAC6(5) has a time constant of ~0.4 ms compared to 2 ms for DiSBAC6(3) (Gonzalez and Tsien 1997). The time constant of DPA used in hybrid voltage sensor systems is ~0.5 ms (Chanda et al. 2005). All the quoted oxonol time constants were measured at 20 °C; increasing temperature to 29 °C speeds up the translocation rate two to threefold (Gonzalez and Tsien 1995).
4 Sensitivity of FRET Indicators
The efficiency of FRET depends on the Förster distance, Ro, the distance of at which FRET is 50 % efficient. To ensure that FRET occurs selectively between the donor and acceptor on the same side of the membrane, ideally Ro needs to be less than the width of the intra- and extracellular binding sites of the membrane (3–5 nm). One way to decrease Ro while maintaining strongly absorbing fluorophores with high quantum yield is to decrease spectral overlap between the donor emission and acceptor excitation spectra (Fig. 6.1c). For the original fluorescein-labeled wheat germ agglutinin (FL-WGA) and oxonol pairs, the measured fluorescence changes where more than 20-fold less than expected from a Nernst equation-based mechanism, meaning the voltage sensitivity of oxonol was not efficiently transduced into a usable FRET signal (Gonzalez and Tsien 1995). Bringing the donor closer to the membrane and decreasing the donor and acceptor spectral overlap, by replacing FL-WGA with CC1-DMPE reduced the minimal distance between the two fluorophores, increasing FRET efficiency and, thereby, the sensitivity of the measurement.
The modification of DiSBAC6(3) to DiSBAC6(5), in addition to increasing translocation speed, shifted the absorption spectrum of oxonol to longer wavelengths by 100 nm (Gonzalez and Tsien 1997). The decreased overlap between the CC1-DMPE emission and DiSBAC6(5) absorption spectra further reduced Ro to 3.7 nm. The reduction in FRET due to the decreased spectral overlap is compensated by an increase in FRET selectivity for oxonol molecules near the extracellular face of the membrane. Separating the spectral overlap between donor and acceptor has the added benefit of limiting inadvertent illumination of the acceptor. Unfortunately, the pentamethine oxonols bleach much faster than trimethine oxonols and this imposes a significant limitation on using this faster oxonol.
The voltage sensitivity of FRET VSDs is maximal when the acceptor can quench most of the donor emission when the two molecules are in close proximity. Thus, the stoichiometry of the loading concentrations of the donor and acceptor needs to be optimized depending on cell type. In theory, it is preferable to have the mobile anion serve as FRET donor because efficient FRET relies on high acceptor concentrations. Lowering oxonol concentration limits photodamage, electrostatic repulsion between oxonol molecules, and the capacitive load on cells. A proof of this principle used DiSBAC6(3) as the mobile FRET donor and Cy5-labelled DMPE as the immobile acceptor for which ratiometric sensitivities of 5–15 % per 100 mV were recorded (Gonzalez and Tsien 1997). Using the mobile ion as the donor and lowering its concentration, however, produced rapid bleaching. Nevertheless, because there are multiple benefits to using the mobile voltage sensor as FRET donor, further development of this approach is warranted (Dumas and Stoltz 2005), including the development of a more photostable mobile fluorescent anion.
The loading concentrations of DiSBAC2(3) and DiSBAC4(3) oxonols are limited to <20 μM for reasons discussed above. For more hydrophobic oxonols, such as DiSBAC6(3) which have been used primarily in cell culture, the loading concentrations are much lower (<2 μM) due to their lower aqueous solubility and higher membrane partitioning. Loading concentrations of CC1-DMPE are limited to <20 μM in leech neurons because higher concentrations lowered the input resistance of the neurons (Cacciatore et al. 1999). The use of the modified compounds, CC2-DMPE or CC3-DMPE, allows concentrations of up to 160 μM to be used without significantly reducing membrane resistance.
The voltage dependence of the sensitivity can be fit by the Boltzman statistics for a two-state system under the influence of a potential (Fig. 6.2c) (Gonzalez and Tsien 1995; Cacciatore et al. 1999). The most linear and sensitive region of this curve lies within the physiological range. As with all VSDs, measured sensitivities vary between cell type and experimental preparation. The largest ratiometric sensitivities of 100–300 % per 100 mV have been measured in isolated cell lines using CC2-DMPE and DiSBAC2(3) (Gonzalez and Maher 2002). The use of faster, more hydrophobic oxonols, DiSBAC4(3) and DiSBAC6(3), yield sensitivities of 60–100 % per 100 mV and 40–80 % per 100 mV, respectively (Table 6.1). This reflects the tradeoff between speed and sensitivity as the hydrophobicity of the voltage sensor increases. Sensitivities are reduced for intact tissues such as a leech ganglion due to background staining. Optimal ratioed sensitivities of leech neurons using CC2-DMPE and DiSBAC2(3) are 10–20 % per 100 mV (Fig. 6.2c) (Cacciatore et al. 1999; Briggman et al. 2005). By comparison, the styryl VSD di-4-ANEPPS had sensitivities in leech neurons of around 0.5 % per 100 mV.
5 Genetically Encoded FRET Sensors
Two major limitations of synthetic membrane potential dyes are poor tissue penetration and indiscriminate cellular staining, both of which produce reduced signal above background. A specific challenge for neuroscience applications is labeling target neurons in among non-neuronal cells such as glia. To address these issues, several approaches have been explored and developed that utilize genetically encoded fluorescent proteins (FP). Initial efforts involved creating fusion proteins between FP and voltage-gated ion channels and then expressing the construct in the cell or tissue of interest. One general strategy is to turn membrane potential changes into conformational changes in voltage-dependent ion channels, which is then transduced into optical changes. Examples of such voltage-sensitive proteins include: Shaker potassium channels (Siegel and Isacoff 1997; Guerrero et al. 2002); a sodium channel (Ataka and Pieribone 2002); and the voltage sensor domain (VSD) of Kv2.1 potassium channel (Sakai et al. 2001; Knopfel et al. 2003). Although detectable, the voltage-sensitive fluorescence changes are small and often have complex kinetics resulting from multiple voltage-dependent conformational changes of the VSD, channel, and FP. With the goals of creating ratiometric probes and increasing the voltage-sensitive fluorescence change, constructs were made to express FP FRET pairs attached to voltage-sensitive proteins. These efforts in combination with using the VSD from a voltage sensor containing phosphase from Ciona intestinalis (Ci-VSD) have led to development of new FRET-based genetically encoded probes VSFP2.x (Dimitrov et al. 2007) and Mermaid (Tsutsui et al. 2008). These probes have improved voltage-sensitive fluorescence changes with ratiometric changes of ~10 % per 100 mV (Mutoh et al. 2009). Using a VSD rather than modified channel proteins has the advantages of (1) not having a channel pore, with it’s own ionic fluxes, and (2) VSDs are much smaller proteins, which should make them easier to express in cells.
Another FRET or energy transfer approach is a hybrid system that uses a genetically encoded membrane-bound donor FP that undergoes voltage-sensitive energy transfer with a hydrophobic anion. A hybrid approach has the potential to offer both cellular targeting of the photon acceptor along with the high sensitivity inherent in using a mobile voltage sensor anion. A FRET hybrid approach between Lyn-domain targeted GFP and DiSBAC oxonols produces large voltage-sensitive FRET response in mammalian cells and is compatible with high-throughput screening (Tsien and Gonzalez 2002). Recently, a hybrid voltage sensor (hVOS) was constructed that uses a membrane-targeted GFP as the donor and dipicrylamine (DPA) as the acceptor; this combination has a high sensitivity (34 % dF/F) in neurons (Chanda et al. 2005). DPA translocates across membranes with sub-millisecond speed and is not fluorescent, so it functions as a quencher so that all fluorescence changes are from the FP (Blunck et al. 2006). Although high sensitivity is possible, the optimal DPA membrane surface densities occur at such high concentrations that they cause toxicity or introduce a huge capacitive load to the Drosophila antennal lobe neurons (Sjulson and Miesenbock 2008). A second hybrid combination used farnesylated FP and the fluorescent oxonol acceptor DiBAC4(5) to measure membrane potential changes in the transverse tubular system of skeletal muscle fibers of mice (DiFranco et al. 2007). Both combinations increase the capacitance significantly, although action potential signal were observed with DPA. Progress in molecular biology and FPs has catalyzed the search for genetically encoded fluorescent reporters of membrane potential. Still the technical constraints of recording millisecond changes in intact neuronal systems without significantly perturbing the biological system are very demanding constraints that have yet to be met.
6 Phototoxicity of FRET Indicators
Because oxonols readily photobleach, they are the primary source of bleaching during extended voltage-sensitive FRET recordings, even when they serve as FRET acceptors. One contribution to photobleaching is the photochemical reaction between excited states of the dyes and oxygen to generate reactive singlet oxygen. Because the mobile anion is located within the plasma membrane, singlet oxygen causes cellular toxicity by reacting with unsaturated lipids and proteins within the cell membrane. Evidence that oxonol is the major contributor to photodynamic damage comes from experiments in which cardiomyocytes (Gonzalez and Tsien 1997) and leech neurons (unpublished observation) stained only with oxonol. Phototoxic effects can be partially mitigated by incubating cells with astaxanthin, a lipid-soluble carotenoid free radical scavenger (Palozza and Krinsky 1992; Gonzalez and Tsien 1997). When applied to cardiomyocytes, astaxanthin increased the usable imaging time by a factor of 10. Astaxanthin has similar beneficial effects to reduce photodamage in second harmonic imaging (Sacconi et al. 2006). A key challenge is to develop additional anti-oxidant compounds that can more readily be loaded into cells.
7 Applications of Synthetic FRET VSDs
FRET VSDs have been used in a variety of cell types with applications including high-throughput pharmacology screens, imaging of single neurons, population imaging of intact nervous systems, multilayered keratinocyte cultures (Burgstahler et al. 2003) and pancreatic islets (Kuznetsov et al. 2005). The primary limitations to using FRET VSDs in complex tissue is being able to deliver uniform and sufficient penetration of the two dye molecules to the target cells while minimizing the nonspecific background staining of non-target cells. Therefore, the most successful applications of FRET VSDs have been in preparations with relatively unhindered access to the cell bodies.
7.1 High Throughput Drug Screening
The use of FRET VSDs has been particularly useful for the characterization of pharmacological compounds on the activity of ion channels and transporters in isolated cell lines (Adkins et al. 2001; Weinglass et al. 2008). Isolated cells are easily stained with the more hydrophobic, fast oxonol derivatives such as DiSBAC6(3), in part, because they do not need to penetrate into tissue. Because the fast fluorescent dyes are very hydrophobic with essentially no aqueous solubility, they bind to the first membrane they encounter. The combination of high throughput ratiometric FRET detection in microtiter well plates and parallel electrical stimulation has many potential applications, including the rapid characterization of hundreds of voltage-gated sodium channel antagonists (Bugianesi et al. 2006; Huang et al. 2006). Recently, FRET VSDs have been shown to be compatible with the highly miniaturized 1,536 well plate format with an assay for an inward rectifying potassium channel (Solly et al. 2008).
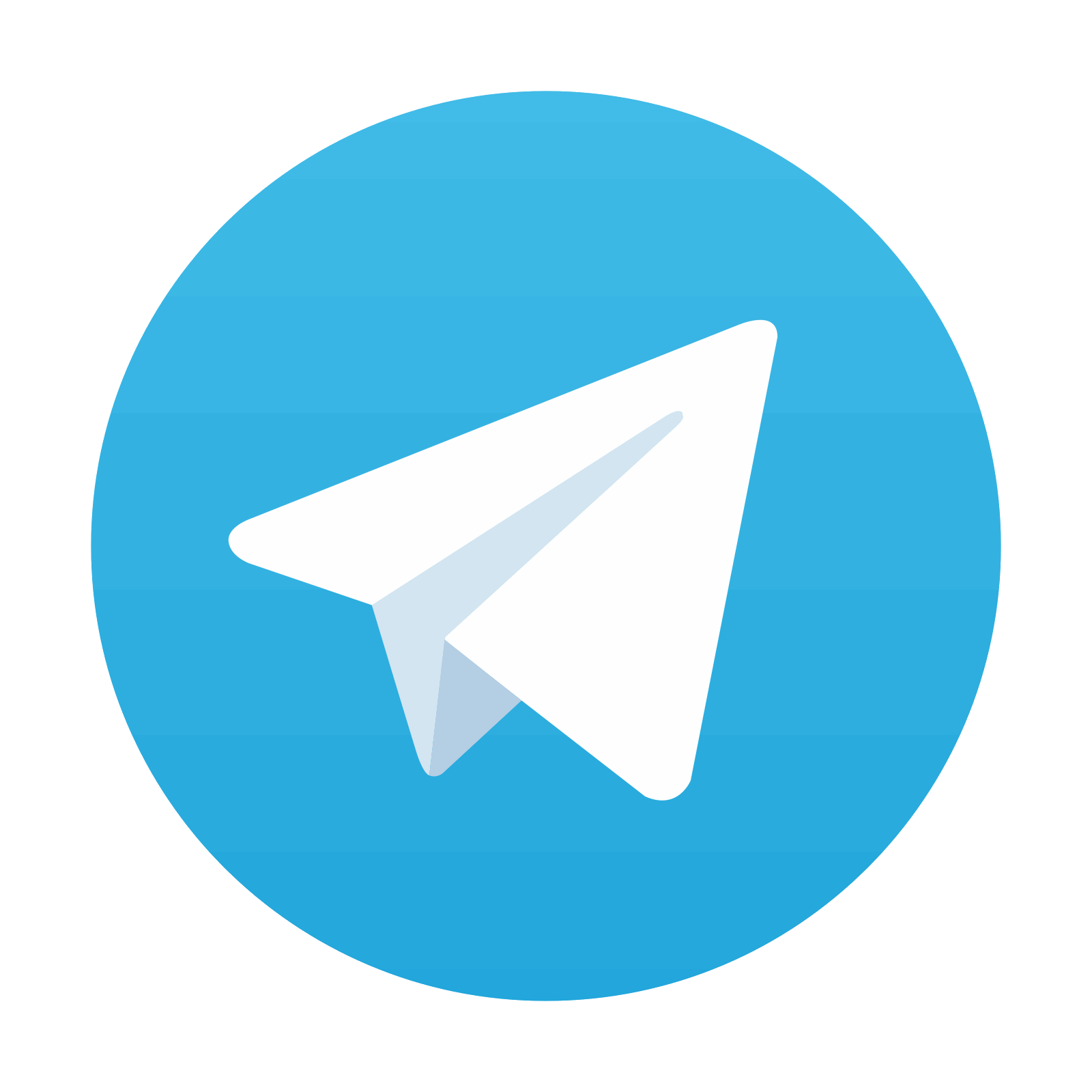
Stay updated, free articles. Join our Telegram channel

Full access? Get Clinical Tree
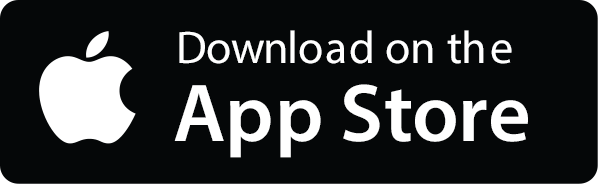
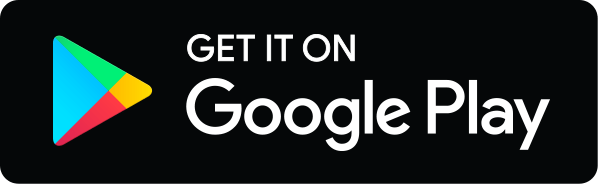