The spine is one of the most commonly imaged body parts for all age groups, with indications ranging from congenital abnormalities at birth to degenerative pathology toward the end of life. Because of the importance of softtissue contrast when imaging the spine, MR imaging has become the most important modality in its evaluation. One of the key challenges in the clinical analysis of spinal MR imaging lies in the wide range of so-called normal variability. The goal of this article is not to provide the reader with an exhaustive atlas detailing the appearance of every known variant. Instead, the authors review the MR appearance of the most important variants and provide a logical and, they hope, memorable framework for assimilating this information into practice. To understand why these variations occur, the authors examine the aberrant pathways of embryology, growth, and development that lead to their formation.
The spine is one of the most commonly imaged body parts for all age groups, with indications ranging from congenital abnormalities at birth to degenerative pathology toward the end of life. Because of the importance of soft-tissue contrast when imaging the spine, MR imaging has become the most important modality in its evaluation. One of the key challenges in the clinical analysis of spinal MR imaging lies in the wide range of so-called normal variability. The goal of this article is not to provide the reader with an exhaustive atlas detailing the appearance of every known variant. Instead, the authors review the MR appearance of the most important variants and provide a logical and, it is hoped, memorable framework for assimilating this information into practice. To understand why these variations occur, the authors examine the aberrant pathways of embryology, growth, and development that lead to their formation.
Before beginning this task, it is important to remember that the definition of normal variant anatomy is itself somewhat arbitrary and vague. For example, normal variants, such as transitional lumbar vertebra, may set the stage for accelerated degenerative arthritis later in life. Similarly, many variants that are normal in isolation can be seen in association with more complex and distinctly pathologic congenital malformations of the spine and other organs. For the purposes of this article, the authors consider normal those variants that do not cause immediate pathology by early adulthood, with the understanding that in the future, as medical knowledge advances, these variants may eventually be considered pathologic and may even be addressed prophylactically.
Normal embryology and development
As a practicing radiologist, it is easy to conceive of the spine as a fait accompli and take for granted the miracle by which the majority of this intricate structure is formed within a matter of weeks beginning with a single cell. The spine is a marvel of form and function, providing key mechanical support while protecting its most delicate and essential neural structures. It is not surprising that considerable variability should arise from such complexity.
Formation of the Vertebral Column
The embryologic precursors of the spine appear in the third week of gestation during gastrulation when the primitive streak forms on the bilaminar embryonic disc, heralding the migration of epiblastic cells through the streak to form a new layer of mesodermal cells interspersed between the layers of the bilaminar disc, thus forming the trilaminar embryonic disc ( Fig. 1 A, B). Along the cranial end of the primitive streak is the primitive node. Cells migrating through the node give rise to the prechordal plate and the notochordal process. It is the notochordal process that eventually becomes the notochord, the embryonic structure most directly responsible for vertebral development. The notochord itself consists of a mucoid matrix with sparse polygonal cells and acts as a kind of scaffold upon which mesodermal cells will organize to give rise to the vertebral column.
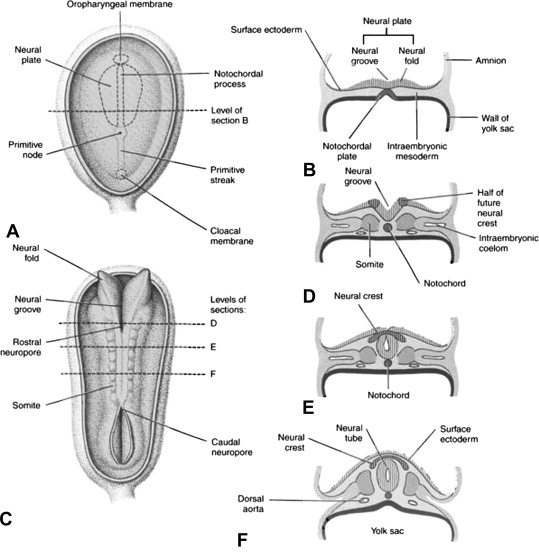
On either side of the notochord, the mesoderm differentiates into 3 parts, one of which is called the paraxial mesoderm. The paraxial mesoderm forms the somites, also called the primitive segments, which are masses of bilaterally symmetric regularly spaced cells (see Fig. 1 C–F). The somites themselves are composed of a sclerotome consisting of cells that will ultimately form the vertebral column, and a dermomyotome consisting of cells that will ultimately form the overlying muscles and skin. Also around this time, the cells along the ectodermal side of the notochord undergo the process of neurulation, whereby the neural plate involutes and fuses to form the neural tube, the precursor of the central nervous system comprising the brain and the spinal cord.
During the fourth week of gestation, the sclerotomes migrate and surround the notochord and the overlying neural tube. Each sclerotome then separates into 2 distinct layers, forming a cranial area of loosely packed cells and a caudal area of densely packed cells ( Fig. 2 ). Between these 2 areas is a cell-free space that will eventually become the site of the intervertebral disc as some mesenchymal cells later migrate into this space to form the annulus fibrosis, leaving the remnants of the notochord to form the nuclear pulposus. The centrum, which will eventually form the vertebral body, is then formed by the cranial, dense area of one somite fusing with the caudal, loose area of the somite immediately cranial to it. Thus, the formation of a normal vertebral body is the result of an intricate union between portions of 2 adjacent somites. The portions of the sclerotomes that surrounded the neural tube go on to form the neural arches, which form the posterior bony elements of the spinal column.
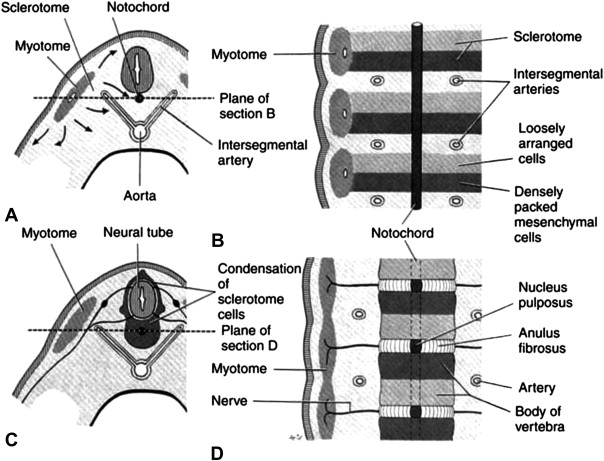
During the sixth week of gestation, signals from the notochord and neural tube lead to chondrification, which ultimately leads to ossification, at which point the notochord disintegrates. There are 3 main ossification centers within each vertebra, one in the centrum and one on each side of the neural arch ( Fig. 3 A). Complete fusion of the 2 portions of the posterior elements is not complete until 6 years of age; whereas, fusion of the vertebral body with the posterior elements is typically not complete until 5 to 8 years of age. Five additional ossification centers form after birth (see Fig. 3 B), one for each transverse process, one for the spinous process, and one each for the superior and inferior vertebral body endplates.
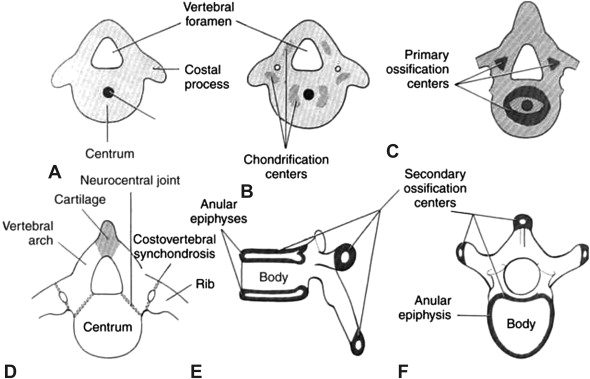
Several notable exceptions to the classical segmentation pattern occur at the occipitocervical junction. The cranial portion of the first cervical sclerotome, sometimes called the proatlas, undergoes complex and unique segmentation and contributes to the occipital condyles, the dorsal cranial articular processes of C1, and the tip of the odontoid process. The caudal portion of the C1 sclerotome forms the anterior and posterior arches of C1 as well as contributing to the odontoid process. The C1 anterior ossification center typically does not ossify until 1 year of age and complete fusion of the anterior with the posterior arches to form the C1 ring is not complete until 7 years of age. The axis is the most complex of all vertebrae and contains 6 ossification centers in utero derived from 2 sclerotomes. Three are derived from the C2 sclerotome, a bilaterally symmetric pair that correspond to the centrum of C1 and the apex of the odontoid, which is derived from the proatlas. The odontoid process will fuse with the body of C2 between 3 and 6 years of age, although a remnant of the apophyseal fusion line can persist up to 11 years of age. A secondary ossification center appears cranial to the odontoid process, termed the ossiculum terminale, at 3 years of age and does not fuse until approximately 12 years of age.
The final developmental aspect of the spine to consider is its curvature. The normal curvature of the fetal and newborn spine is kyphotic. The cervical and lumbar lordosis emerge after birth and are thought to be functional in origin: the cervical curvature resulting primarily because of the infant holding his head upright and the lumbar lordosis emerging later in development as the infant/toddler assumes an erect posture.
Formation of the Spinal Cord
Formation of the spinal cord is classically divided into 2 main movements: primary and secondary neurulation. Primary neurulation begins in week 3 when the newly formed notochord induces the adjacent ectoderm to form 2 ridges that fuse to form the neural tube, the structure that will ultimately give rise to the brain and spinal cord. Fusion begins at or near the fourth somite and proceeds in both directions, typically complete by day 26.
The process of secondary neurulation refers to the formation of additional caudal elements of the spinal cord and is further divided into canalization and retrogressive differentiation. During the process of canalization, a group of undifferentiated cells at the caudal end of the neural tube develops cysts or vacuoles that merge with one another and eventually with the central canal of the caudal end of the cord to further elongate the neural tube. Retrogressive differentiation refers to the process whereby the caudal cell mass regresses, eventually leaving only the conus medullaris, ventricular laminalis, and the filum terminal. The process of primary neurulation accounts for structures as caudal as somite 31, or the vertebral level of S2. Vertebral bodies up to S2 are thus derived from the notochord whereas those below S2 derive from the caudal cell mass.
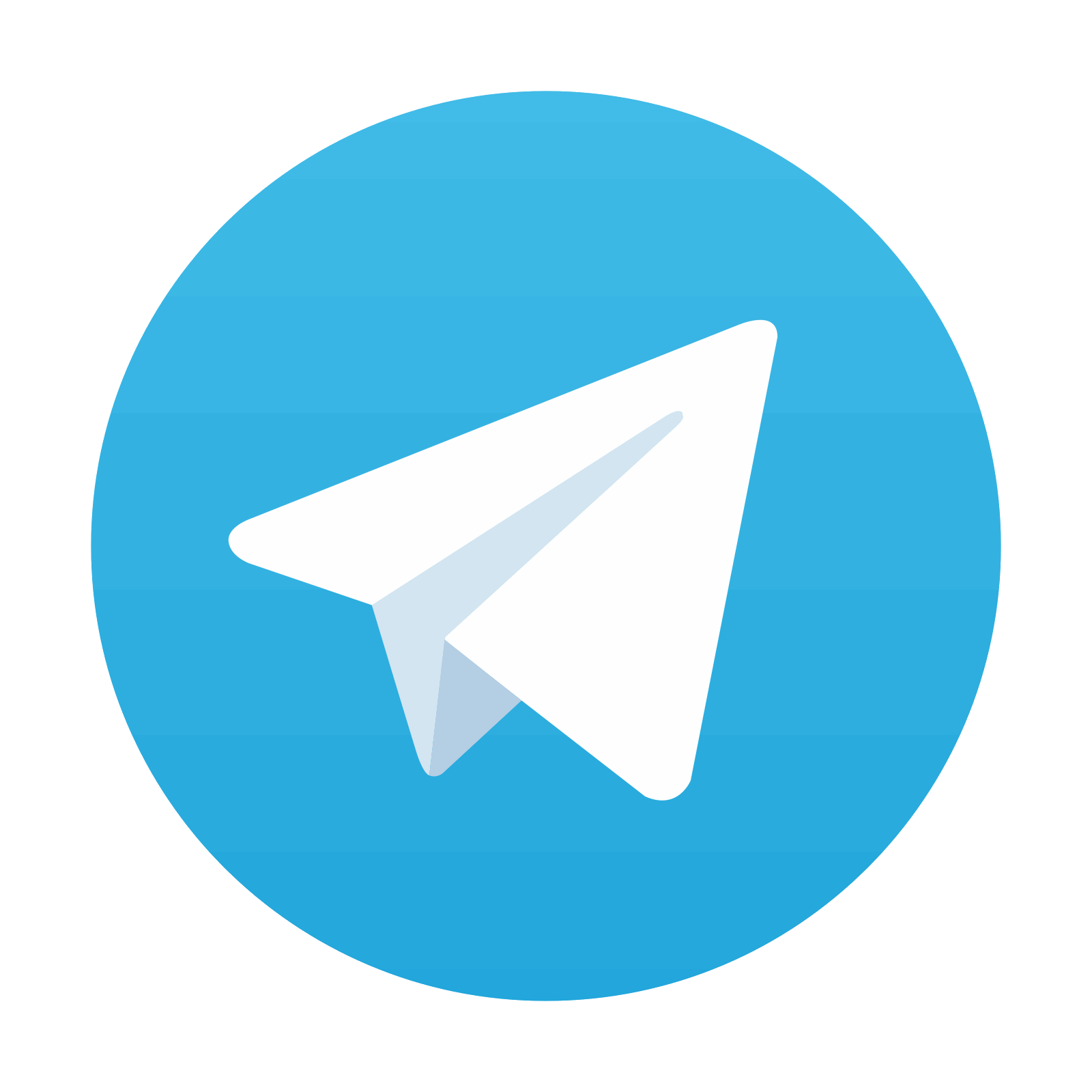
Stay updated, free articles. Join our Telegram channel

Full access? Get Clinical Tree
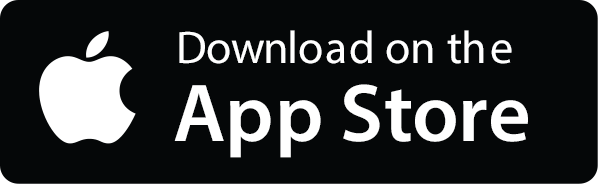
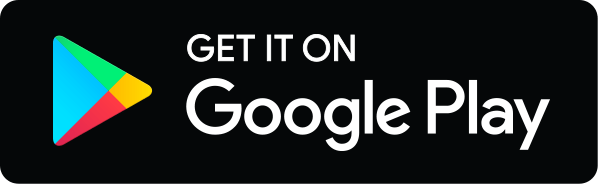