Fig. 21.1
Axial (a), sagittal (b), and coronal (c) T2-weighted images of the prostate in a 66-year-old with increasing PSA levels. The images were acquired at 1.5 T, with an endorectal coil for staging, and diagnosed as stage T2a prostate adenocarcinoma, with a PSA of 2 and Gleason score of 7 (3 + 4). The suspicious lesion (arrows) presents as a hypointense region in the left posterolateral aspect of the mid-gland and base, extending almost to the apex
An extremely valuable functional imaging complement to T2-weighted imaging is diffusion-weighted imaging (DWI). DWI usually uses a lower-resolution echo-planar imaging acquisition sequence, which can be prone to distortion and susceptibility artifacts from nearby air-tissue or metal interfaces. Large gradients are applied that strongly attenuate freely diffusing water molecules in tissue. Prostate carcinoma is often characterized by high cellularity, which restricts water diffusion and results in hyperintensity on DWI. Fluid regions, such as cysts, have extremely high signals to start with; thus, the residual signal may also appear hyperintense on DWI. To remove this “T2 shine-through” effect, maps of the apparent diffusion coefficient (ADC) are generally constructed using two or more weighting values (“b-values”), generally of 0–1,000 s/mm2. Most modern scanners automatically generate these maps as a separate series to the DWI if the option is purchased and turned on in the protocol. These ADC maps are reflective of the diffusion coefficient itself; therefore, contrast is inverted with respect to DWI (i.e., restricted diffusion lesions appear hypointense on ADC maps). Although these derived ADC maps may appear noisier than DWI, they can be essential to the proper interpretation of DWI findings (Fig. 21.2).
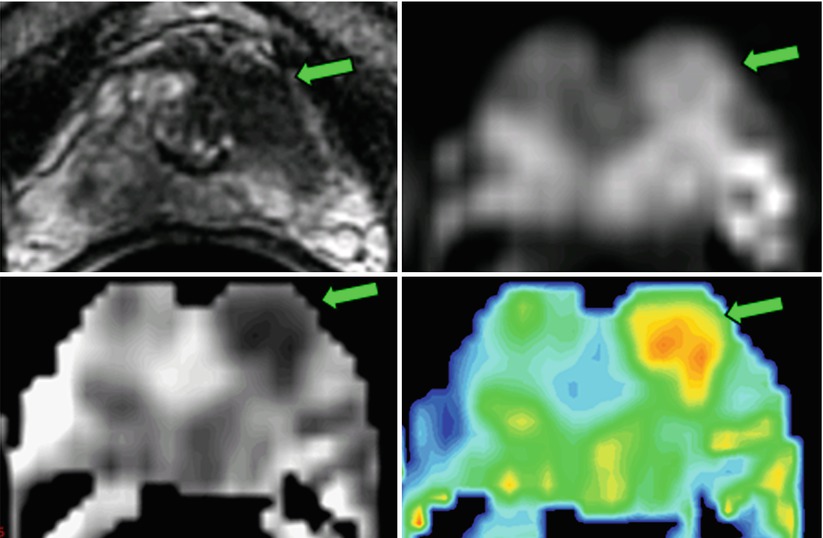
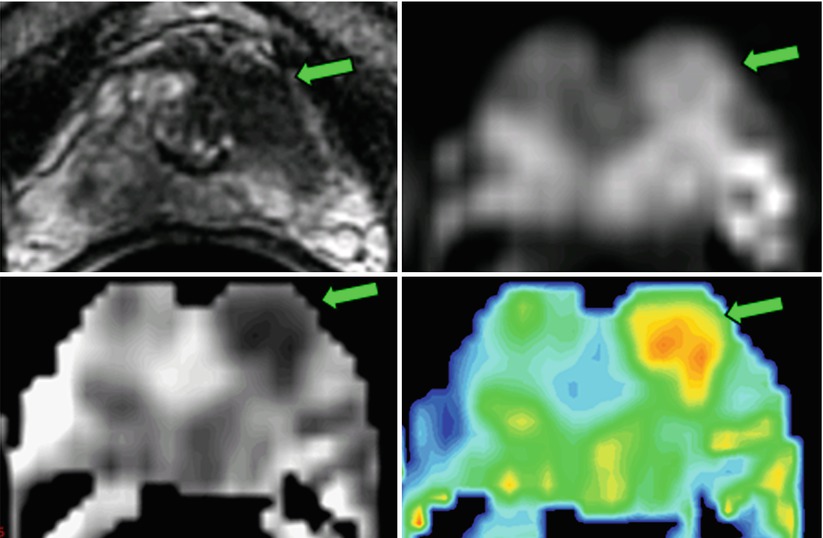
Fig. 21.2
Diffusion-weighted imaging (b = 0,700 s/mm2) of the prostate in a 74-year-old, acquired at 1.5 T with an endorectal coil. The lesion, which had a Gleason score of 7 (3 + 4), was in the transition zone 12 weeks after TRUS-guided biopsy. The T2-weighted images demonstrate the lesion (arrow) as an abnormally hypointense region. The lower-resolution diffusion-weighted image (700 s/mm2) reveals a coinciding region of hyperintensity (arrow). The calculated apparent diffusion coefficient (ADC) map depicts a region of reduced diffusion (0.80 × 10−3 mm2/s) on the left (arrow) versus the contralateral side of the gland (1.6 × 10−3 mm2/s). The ADC can be presented as an “exponential ADC” map to mimic the positive contrast characteristics of the DWI image, but without the T2 “shine-through” effects
T1-weighted images do not have a similar level of anatomical or zonal contrast, and the gland tends to be isointense. Hemorrhage often appears hyperintense against this background, making T1-weighted images useful for interpreting regions of hypointensity on T2-weighted images. Because of this and because of the usefulness of T1-weighted images in evaluating regional lymphadenopathies, periprostatic structure invasion, and bone lesions, T1-weighted imaging is usually performed in only one plane, with a larger field of view for diagnostic imaging (Fig. 21.3).
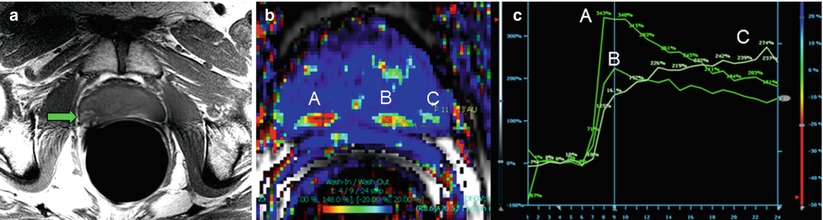
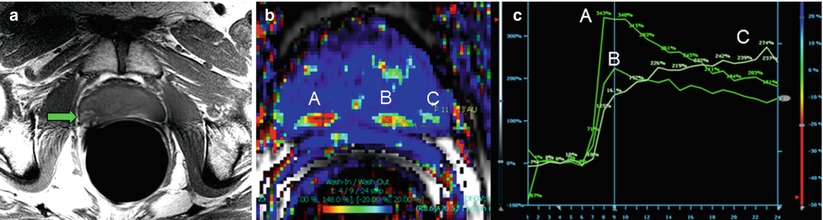
Fig. 21.3
T1-weighted, fast spin-echo imaging is useful for depicting hemorrhage, which appears hyperintense against the isointense prostate gland (a). In a different patient, dynamic contrast-enhanced imaging using a fast gradient-recalled echo technique reveals contrast kinetics in the prostate that can be used in conjunction with T2-weighted or DWI to aid in localizing potential lesions (b). The false color map in (b) shows rapid washout as red. The points at several suspicious locations in the peripheral zone (A, B, and C) can be plotted to visually assess the kinetics (c)
Static pre- and post-contrast T1-weighted imaging is not useful for tumor evaluation because of nonspecific contrast uptake in the gland [27, 28]. Dynamic contrast-enhanced (DCE) MRI of the prostate provides a means to observe contrast uptake kinetics via the change in the T1-weighed signal over time (Fig. 21.3) [29]. The time course of the signal is usually analyzed using a workstation with dedicated software that also generates metrics on the basis of the contrast kinetics in the tissue. Tissue with rapid uptake and contrast agent washout are suspicious for malignancy [30]. Although techniques vary widely and the practice is evolving, acquisitions usually incorporate a rapid (<8 s per volume), 3D gradient-recalled echo technique after an injection of gadolinium-based contrast agent (~3 ml/s). In a manner analogous to incorporating color and power Doppler in conventional TRUS-guided biopsy, DCE-MRI may play a role in biopsy cases, aiding in further increasing the sensitivity of the technique and reducing the number of samples required to obtain a diagnostic result [31]. However, post-biopsy changes are still difficult to interpret, with some investigators suggesting the use of MR spectroscopy to aid in localizing suspicious regions [32].
MR spectroscopy of the prostate is a 2D or 3D acquisition technique that suppresses water and lipid signals to observe alternative metabolites that have signal-producing protons (Fig. 21.4). Each metabolite resonates at a slightly different frequency on the basis of its chemical environment; thus, results are often shown in terms of a spectrum. The acquisition is of low spatial resolution, and 15–20 min is usually required to acquire a low-resolution 3D volume through the prostate. Maps of certain metabolites may be generated by vendor software by quantifying the signal in windows that approximately isolate these metabolites. Citrate (~2.6 ppm) is a by-product of normal gland metabolism that should dominate in the normal gland but is often highly attenuated in tumors. Choline (~3.2 ppm) is a by-product of cell membrane construction and maintenance that tends to increase with the proliferative cell activity associated with prostate carcinoma, whereas creatine (~3.0 ppm) does not. The variable amount of spermine in the prostate is associated with polyamine (~3.15 ppm) metabolite signals between choline and creatine that tend to make quantification of choline alone difficult on clinical scanners. Therefore, a common metric for characterizing prostate spectroscopy is the ratio of the choline-to-creatine signal to the citrate signal. The larger this ratio becomes, the more suspicious the voxel is for cancer. However, despite the recent success of numerous single center trials, a rigidly controlled, prospective multicenter study of 110 patients (mean PSA level = 5.9 ng/ml and median Gleason score = 7) conducted by the American College of Radiology Imaging Network determined there was no quantifiable benefit of combined endorectal MRI and MR spectroscopic imaging at 1.5 T versus endorectal MR imaging alone for sextant localization of peripheral zone prostate cancer [24]. Although the authors reasoned that this could be partly due to selection bias, it is important to realize that endorectal coil MR spectroscopy was still an emerging technology when these studies were conducted. Both the sensitivity and resolution of spectroscopy increase at 3 T. This, coupled with advancements in the number, fidelity, and dynamic range of modern multichannel systems, as well as more widespread use of higher order shimming and advanced spectroscopy analysis packages, are promising.
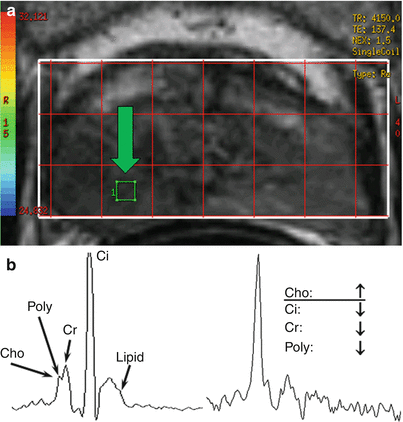
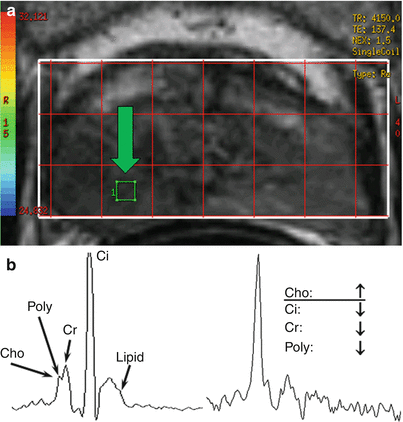
Fig. 21.4
1.5 T MR spectroscopy using an endorectal coil is a low-resolution technique that provides relative metabolite concentrations in the region of interest. 3D MR spectroscopy is usually prescribed from an axial anatomical image. The spectrum from each voxel in the grid (a) can be analyzed independently or displayed as a montage of spectrums; alternatively, specific metabolite maps can be derived and shown. A typical prostate MR spectroscopy voxel has components of choline (Cho), polyamines (Poly), creatine (Cr), and citrate (Ci) and may have lipid contamination (b). Voxels containing tumor (arrow in (a)) exhibit altered metabolism, often characterized by increased levels of choline and decreased levels of other metabolites (c)
MRI Guidance for Prostate Biopsy
Because of its ability to visualize suspicious lesions for targeting, substantial interest has developed in the use of MRI guidance for prostate biopsy, particularly in patients with negative TRUS biopsies and persistent clinical indicators for prostate cancer [22, 33]. Several research groups have evaluated the value of MRI versus TRUS-guided biopsy and have found that MRI can enhance or is superior to the predictive capabilities of TRUS-guided biopsy [34–36]. A review of the recent medical literature revealed an accuracy rate of 69–82 % for T2-weighted imaging and 86–89 % for DWI using endorectal coil MRI at 1.5 T [37]. There is a trend toward incorporating a multimodal approach that includes T2-weighted imaging with DWI and sometimes DCE or MRS to localize prostate carcinoma [38].
Indications and Patient Selection
Because of early detection with modern screening, most patients do not present with clinical symptoms of advanced prostate cancer, such as urethral obstruction, but instead tend to be asymptomatic. General indications for a prostate biopsy include an abnormal PSA test on the basis of patient age and abnormal findings on a DRE. Repeat biopsy may be indicated when the sample is not satisfactory for pathological analysis, contains too little tissue, or is of insufficient quality. Repeat biopsy may also be indicated for patients with a previous normal biopsy and persistently abnormal or increasing PSA levels and patients with a suspicious previous biopsy result, such as high-grade prostatic intraepithelial neoplasia or atypical small acinar proliferation. High-grade prostatic intraepithelial neoplasia is associated with undetected cancer in 50–80 % of cases [39, 40], whereas atypical small acinar proliferation is associated in 40–50 %.
The role of MRI-guided prostate biopsy is currently being investigated, in patients with one or more negative TRUS-guided biopsy results [34]. Repeatedly negative TRUS biopsies in patients with persistently abnormal or increasing PSA levels can be difficult for patients and urologists. Subsequent conventional TRUS biopsies result in low detection rates, which have been reported to be 14–22 %, 10–15 %, 5–10 %, and 4 % for first, second, third, and fourth repeated biopsies, respectively [41, 42]. The results of subsequent studies in men with abnormal PSA levels (4–10 ng per 100 ml) indicate that TRUS has an approximately 40–45 % detection rate for a standard 10–12-core transrectal approach [43, 44]. Although third and fourth repeated biopsies often result in a lower grade and stage, first and second repeated biopsies detect localized cancers of equivalent stage and grade as in the initial biopsy [45]. Saturation biopsies have been suggested to increase sensitivity and are associated with more accurate Gleason scores [46]; however, they do not significantly increase detection rates on first biopsy [47, 48]. Most recent studies of MRI-guided prostate biopsy have included patients with high PSA levels (>4.0 ng/mL) and one or more negative TRUS biopsy results.
Contraindications to MRI-Guided Prostate Biopsy
Prostate biopsy may be contraindicated in patients taking oral anticoagulants or anti-inflammatories and in those with thrombocytopenia. For transrectal approaches, periproctal abscesses and rectal tumors are contraindications, as is acute, painful perianal disorder and hemorrhagic diathesis. Prostate biopsy is also contraindicated in patients with suspected acute bacterial prostatitis within 6 weeks of intervention because of the potential complication of seeding the bacterial infection in adjacent organs, which may result in gram-negative sepsis.
Any contraindications for MRI preclude MRI-guided biopsy. Patients should be carefully screened for contraindicated implants and devices. A surgically absent rectum, severe hemorrhoids, or previous colorectal surgery may preclude the use of an endorectal coil or transrectal route. Contraindications for intravenous gadolinium administration and anesthesia and the effect of other urinary or medical conditions should also be considered.
Potential Complications
The complications associated with prostate biopsy include the risk of infection (sepsis or urinary tract or prostate infection), hemorrhage at the biopsy site, and urinary retention [49]. TRUS-guided transrectal or transperineal biopsies of the prostate are generally well-tolerated procedures, with minor pain and morbidity. Minor complications are reported in 3–5 % of patients [10]. Routine post-biopsy complications that manifest acutely include rectal bleeding and mild to severe hematuria, whereas delayed hemorrhagic complications include hematospermia and mild hematuria. Repeat biopsies performed at least 6 weeks later do not appear to be associated with any significantly different complications. Of the minor hemorrhagic complications, only hematospermia tends to demonstrate a significant positive correlation with the number of cores taken (6 versus 10) [50]. A prospective study of morbidity and quality of life after 6- versus 12-core approaches found no substantial increase in morbidity [51, 52].
MRI-Guided Approaches
Early approaches to adapting MRI for prostate interventions were generally performed using low magnetic field systems (i.e., 0.2–0.5 T), allowing for significant patient access and resulting in fewer biopsy equipment artifacts [53, 54]. However, to achieve the image quality needed for optimal prostate visualization and targeting, higher fields were required. Many early studies of MRI-guided biopsy were performed using these systems, which often substituted T1-weighted imaging for guidance over T2-weighted imaging. Generally, there has been a transition toward performing prostate interventions at standard clinical field strengths (i.e., 1.5–3.0 T) to obtain better image quality. However, it is important to note that the availability of a prior diagnostic MRI performed with an endorectal coil is invaluable when the goal is to target a specific suspicious area, as it can save additional imaging time, allowing faster sequences to be used for targeting.
Most current approaches to MRI-guided prostate biopsy use phased-array radiofrequency coils to obtain appropriate reception for pelvic imaging without the need for an additional endorectal coil, although commercial biopsy systems that incorporate endorectal coils are being developed [55]. Given the location at which most radiofrequency coils attach to the MRI scanner, the most appropriate position of the patient will often be feet first. The patient may lie supine or prone, primarily on the basis of the approach. Practitioners considering transrectal or transperineal approaches should note that in this position the ability to work from the back of a short-bore MRI scanner can be critical to patient access.
Transrectal Approach
In the transrectal approach to MRI-guided prostate biopsy, an applicator is placed directly into the rectum under MRI guidance, and a needle is passed directly through the rectal mucosa for biopsy sampling. A recent review of TRUS-guided biopsy studies reported that most urologists prescribe a cleansing enema for bowel preparation to reduce the bacterial load in the rectal mucosa [49], despite little evidence of effectiveness [10]. However, this procedure also reduces discomfort and image quality issues associated with the presence of fecal matter if an endorectal coil is used.
Antibiotics, such as fluoroquinolone, may be prescribed as early as 30–60 min prior to the biopsy and up to 12 h after the biopsy aid in infection control [10, 56]. Most transrectal biopsy infections are caused by Escherichia coli or Enterococcus species.
Cessation of anti-inflammatory or anticoagulant therapies (e.g., aspirin or warfarin) 7–10 days prior to the procedure is a common practice. However, it should be noted that research indicates that such practices have a negligible effect on hemorrhagic complications [57–59].
One benefit of the transrectal approach is the reduced need for general anesthesia. Varying local anesthesia techniques have been reported in the medical literature, but many studies have found that periprostatic lidocaine is superior to intrarectal lidocaine [10, 56], and that peristalsis can be suppressed with the intravenous administration of butylscopolaminebromide and an intramuscular administration of butylscopolaminebromide and glucagon [60].
Patients are positioned supine in the MRI, in a partial lithotomy position. Because of the difficulty of manually maintaining the position of the needle guide during the entire navigation process, robotic assistance in the form of an articulating arm is often necessary. An example of a commercially available device designed for safe MRI use is shown in Fig. 21.5. With this approach, the patient can be positioned supine or prone on the table after undergoing a DRE to verify an unobstructed approach to the prostate (Fig. 21.5).
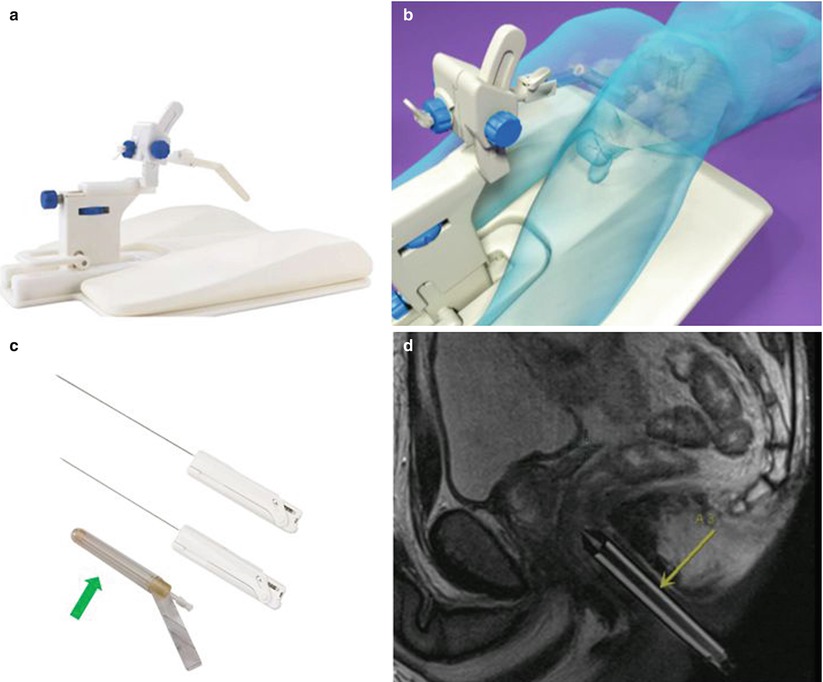
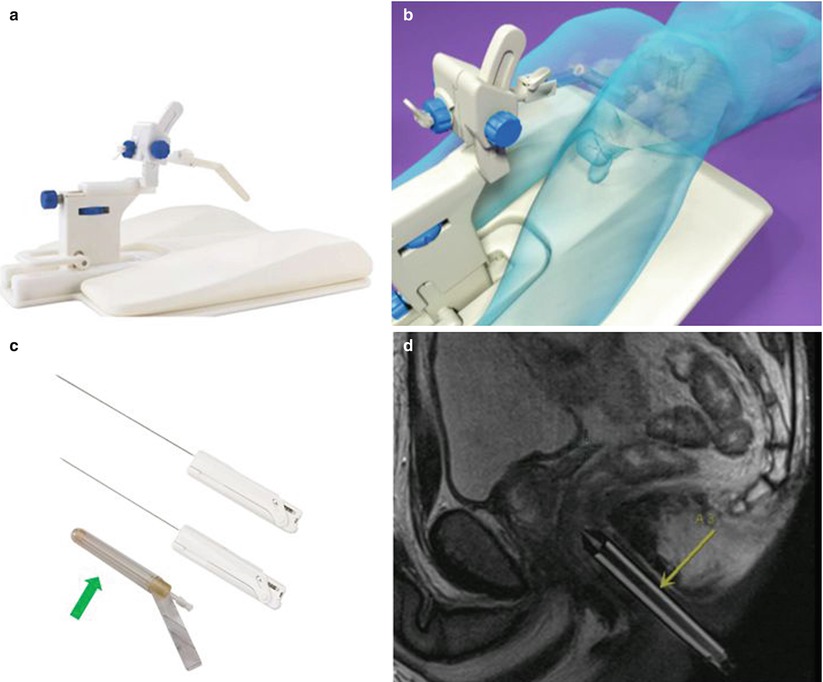
Fig. 21.5
An example of a commercialized system designed for transrectal MRI-guided prostate biopsy is illustrated. An articulating arm, clamped to a baseplate (a), is used to hold an MRI-visible needle sleeve (arrows), which is positioned in the patient’s rectum (b). The disposable needle sleeve (c) is used as both a fiducial and a guide for the biopsy needles. An example of two forward throw, automatic 18-gauge biopsy guns (15 and 17.5 cm) is shown in (c). A sagittal MRI illustrates the needle sleeve in a patient for planning (d) (Courtesy of Invivo Corporation)
In the transrectal approach, the patient sits atop a reusable padded polyoxymethylene baseplate to which an articulating arm is clamped (Fig. 21.5b); their weight helps secure the position of the system on the table. A disposable, MR-visible needle sleeve filled with a gadolinium-based contrast agent is inserted gently into the rectum and attached to the articulating arm. This sleeve acts as a fiducial to illustrate the path of the biopsy needle (Fig. 21.5c, d). Sagittal planning images of the prostate, including the sleeve, are acquired, and gross adjustments are made to ensure that the needle sleeve is appropriately located near the prostate. New sagittal images are obtained, followed by axial images that better demonstrate the anatomy. For these purposes, a fast sequence with T2-like weighting is used, such as a balanced, steady-state, free-precession (bSSFP) sequence, which provides T2-like weighting, at a high resolution, in seconds. However, if the contrast of the sequence is not adequate for initial targeting, a high-resolution, T2-weighted, fast spin-echo approach, with an acquisition time of several minutes, should be used. These sequences can be used to iteratively fine-tune the position of the needle sleeve and plan the needle insertion. Because this can be a time-consuming process, software can guide the adjustments on the basis of the sagittal visualization of the needle sleeve and target region on the axial images. Once the orientation of the needle sleeve is dialed into the articulating arm, a verification image should be acquired. This procedure is followed by inserting the biopsy needle to the anticipated depth. If a guide needle is used, the location of the guide needle with respect to the throw of the biopsy gun can be evaluated. Biopsy guns suitable for use in the MRI environment (Fig. 21.5c) can then be inserted and cores acquired. No support exists for the biopsy gun; thus, a verification image of the needle location will be unavailable if the radiologist does not have sufficient access. If there is access to the patient, real-time imaging may be useful to manually fine-tune the sampling position and verify the location prior to acquiring the tissue sample. If multiple sites of interest exist, the next site of interest is planned, and the procedure is executed again.
Beyersdorff et al. [35] were one of the first research groups to report on this approach and investigated a real-time guided transrectal approach in a cylindrical bore 1.5 T scanner using a pre-market version of this device and no guidance software. Patients (n = 12) had negative TRUS biopsy results and increasing PSA levels. They were positioned prone, and the MRI-visible needle guide (Invivo Corp., Schwering, Germany) was placed rectally and connected to an MRI-compatible articulating arm mounted to a baseplate. A telescoping rod was used to facilitate needle manipulation from outside the bore under real-time MRI guidance. High-resolution, fast spin-echo, T2-weighted images were acquired for initial planning, and a T2-weighted, single-shot, fast spin-echo acquisition was used to guide the needle during the procedure. In five patients, MRI-guided biopsy was performed immediately after a diagnostic endorectal coil MRI. Seven patients returned after <2 weeks for a separate MRI-guided biopsy session that did not include additional imaging. In all cases, MRI guidance for biopsy used torso array coils but no endorectal coil. An eight-core biopsy strategy was used, with sampling being directed at the most suspicious area of each sextant on the basis of the targeting MRI. Procedure durations of <60 min were reported. Approximately 50 % of biopsies directed at the most suspicious locations on T2-weighted MRI were diagnosed as prostate cancer, versus 33 and 3.5 % of biopsies in moderately and not suspicious areas, respectively. Overall, prostate cancer was diagnosed in 42 % of patients.
Engelhard et al. [61] used a similar transrectal MRI-guided biopsy methodology in a clinical closed-bore 1.5 T scanner using the same device. In this study, patients (n = 37) were positioned supine in a closed-bore clinical 1.5 T MRI. Endorectal coil-based diagnostic T2-weighted images were acquired prior to biopsy. Localization of the needle sleeve was performed using fast bSSFP sequences to guide and document the needle position prior to sampling. The 4–9 MRI-directed locations per patient took up to 150 min and resulted in a prostate cancer diagnosis in 37 % of patients. Another similar study in 27 patients by Anastasiadis et al. reported prostate cancer detection in 55 % of patients [62]. Hambrock et al. evaluated a large cohort of patients (n = 71) with increasing PSA levels and two or more negative TRUS biopsy results; using a clinical 3 T scanner and a multiparametric imaging approach, they found a 59 % detection rate with a median of four cores [63].
Investigators at the National Institutes of Health and Johns Hopkins independently designed and demonstrated the feasibility of a transrectal biopsy system that facilitates real-time needle guidance in a standard cylindrical bore 1.5 T scanner via a robotically assisted transrectal approach [55]. A dedicated endorectal coil was developed that could accommodate transrectal biopsy, enabling simultaneous diagnostic-quality MRI via a transrectal approach. Instead of passive-contrast fiducials, active tracking coils were embedded. The primary goal of the study was fiducial placement in previously diagnosed patients for radiotherapy, but three patients underwent a biopsy procedure to demonstrate the system. Patients were positioned prone; the procedure was performed using local anesthesia and lasted a mean of 76 min. The needle (14 gauge) placement accuracy was reported to be 1.8 mm. This system has been further developed into a complete system by Sentinelle Medical, and further results are anticipated.
Transperineal Approach
In the transperineal approach, the needle does not pass directly through the rectum; therefore, fecal contamination is not as critical an issue as with transrectal biopsy (Fig. 21.6). If an endorectal coil is not used, an enema is not necessary, as it is in transrectal approaches. This approach is also useful for patients in whom a rectal approach is contraindicated.
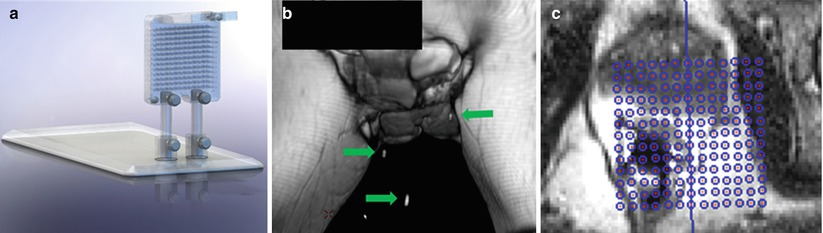
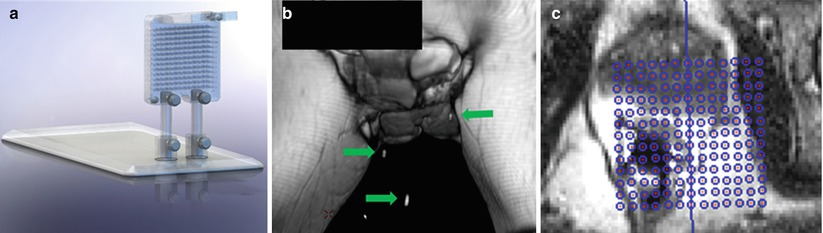
Fig. 21.6
Perineal grids (a) for the MRI environment are commercially available to facilitate transperineal approaches to prostate biopsy. The grid above has integrated MRI-visible markers (arrows) that can be visualized by fast T2-weighted MRI acquisition; (b) the marker coordinates are fed into proprietary vendor software, operating on a stand-alone workstation, to provide a planning grid (c) (Courtesy of Visualase, Inc.)
A recent study of TRUS-guided transperineal approaches recommended antibiotic prophylaxis in patients at high risk for infection, those of poor general health, and those with cardiac valve problems, prosthetics, an indwelling catheter, or high post-voiding residual urine [49]. As with the transrectal approach, fluoroquinolones are the antibiotic of choice. Lidocaine has been locally administered in transperineal approaches [64] that rely on one or two penetrations; however, general anesthesia is preferred. This is particularly true in the MRI environment because of the potential duration of the procedure and the potential for multiple cores or targets to be sampled, particularly if stereotactic grid targeting and planning is used.
Transperineal TRUS-guided biopsy has been found to detect prostate cancer at a rate of 45 % in men with elevated PSA levels using a 12-core technique [65], 36 % using a 14-core technique [66], and 43 % using a saturation technique (9–33 cores) [67]. Similar detection rates have been found for secondary biopsies, with reports of 43 % [68] and 42.2 % [69]. In a recent study, Taira and colleagues found a 75 % detection rate for primary biopsy versus 55.5 %, 41.7 %, and 34.4 % in men with negative first, second, and third biopsies, respectively, when using a template technique that identified 24 locations, with 1–3 cores taken per location on the basis of prostate size [70]. Most of the cancers were multifocal, with 61.1 % of the men diagnosed having ≥5 positive cores and 25.3 % having ≥12 positive cores.
The transperineal approach is amenable to a single point of entry, followed by a “fan” sampling approach under real-time guidance. However, using a template-based grid approach in cylindrical bore magnets allows the incorporation of stereotaxy, in conjunction with intermittent imaging, in place of real-time imaging, such as in cases in which patient access is limited or specific site targeting is desired. Several research groups have had success with this approach. A potential source of error during needle placement is prostate motion or rotation, which has been shown to be substantial [71].
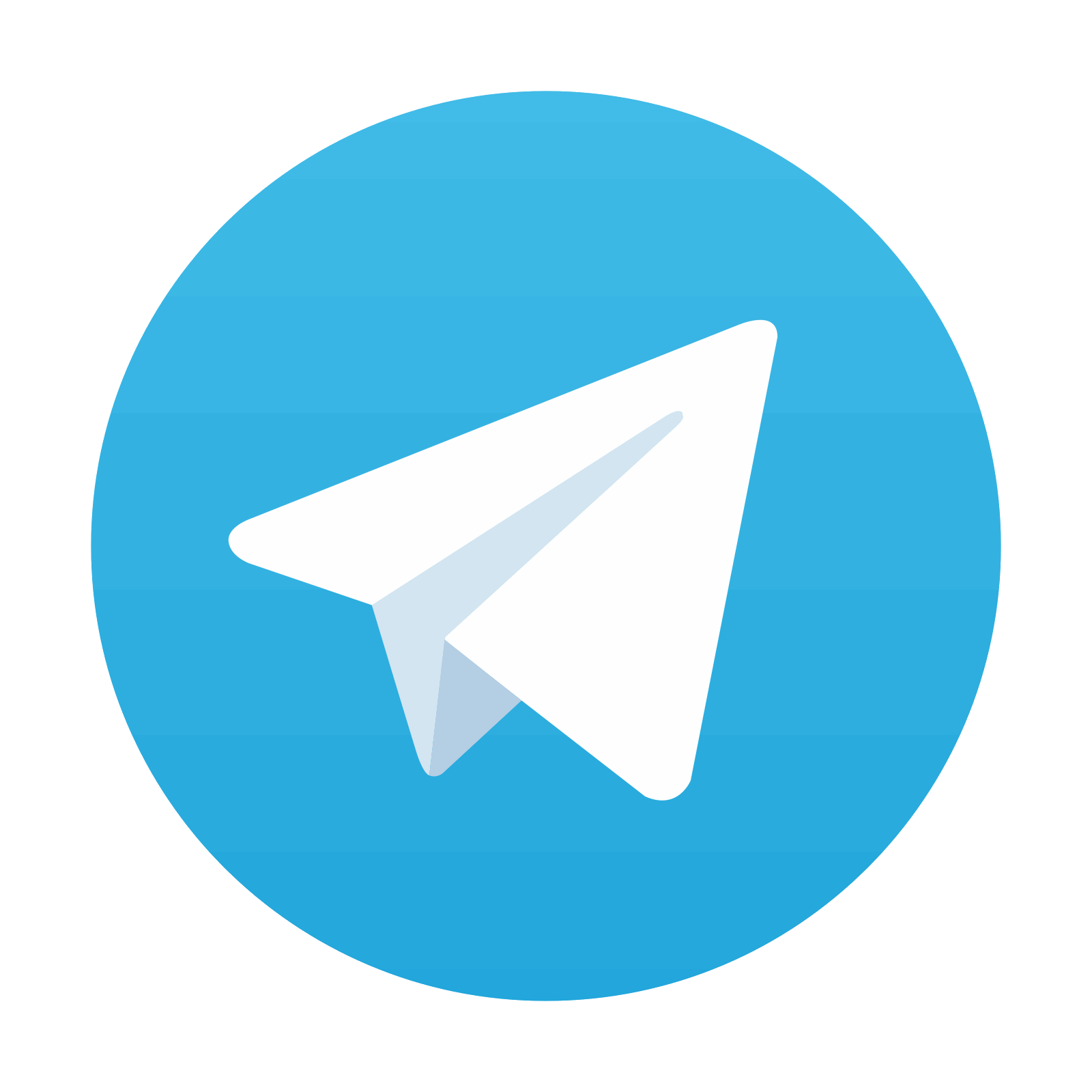
Stay updated, free articles. Join our Telegram channel

Full access? Get Clinical Tree
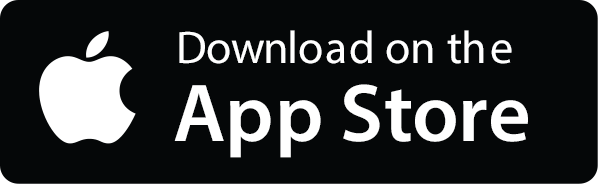
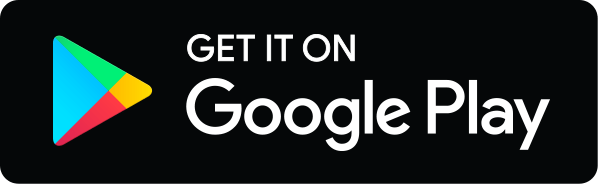