The rotator cuff muscles and tendons are important stabilizers of the shoulder. These structures provide 80% of the external rotation force and up to half of the abduction mechanism. Injury to the rotator cuff leads to shoulder pain and dysfunction.
Rotator cuff disease can be related to a number of factors that include degenerative, vascular, traumatic, and mechanical causes. The most common cause of rotator cuff pathology is primary degeneration of the rotator cuff tendons with wear and aging. External and internal impingement, microinstability of the shoulder, trauma, overuse associated with athletic and occupational activities, underlying systemic disorders that weaken the tendon such as diabetes, renal, and collagen vascular disease, steroid use, and smoking contribute to rotator cuff pathology. Identification of the problem and the origin of the disease can often be augmented by the use of MRI. This chapter focuses on the capabilities, pitfalls, and limitations of MRI for evaluation of the rotator cuff.
Brief Anatomic Review
The rotator cuff complex is a functional–anatomic unit composed of the supraspinatus, infraspinatus, teres minor, and subscapularis tendons and muscles along with the capsular covering between the supraspinatus and subscapularis known as the rotator interval. On sagittal MR images, the muscles and tendons of the rotator cuff are seen in cross-section (Fig. 4.1). When considering musculotendinous position with respect to the humeral head, the supraspinatus lies superior, the infraspinatus posterosuperior, and the teres minor posteroinferior. The rotator cuff muscles are dynamic stabilizers of the glenohumeral joint. They provide anterior stability to the glenohumeral joint in the mid- and end ranges of motion. The supraspinatus and subscapularis muscles stabilize during extension; the infraspinatus, subscapularis, and latissimus dorsi muscles stabilize during flexion; and the subscapularis muscle stabilizes during external rotation.
![]() Figure 4.1 Normal rotator cuff muscles in cross section on a sagittal T1-weighted image at the level of the coracoid. SS, supraspinatus; IS, infraspinatus; TM, teres minor; SUB, subscapularis. |
The supraspinatus consists of two muscle bellies and three longitudinal tendon bands. The anterior portion is a larger, more fusiform muscle that originates from the supraspinatus fossa and continues as a thick tendon that extends from within the muscle to attach primarily onto a layer of uncalcified and calcified fibrocartilage at the superior (horizontal) and middle facets of the greater tuberosity just behind the biceps groove (Fig. 4.2). The anterior portion the tendon is stronger than the posterior portion and is the main functional portion of this tendon. The larger anterior supraspinatus muscle places more force on the anterior tendon compared with the middle and posterior portions of the tendon. The posterior tendon originates from the scapular spine and glenoid neck and extends as a flat, thin tendon comprising the posterior 60% of the tendon (1). It is smaller, unipennate, and thinner in cross-section than the anterior and middle thirds. The attachment of the entire supraspinatus tendon complex to the greater tuberosity is broad and is called the “footprint.” Another anterior and small portion of the supraspinatus tendon attaches to the lesser tuberosity. This attachment allows for internal arm rotation. Both supraspinatus tendons come together with an orientation approximately 50 degrees to the coronal plane of the shoulder. The anterior portion of the supraspinatus tendon merges with the coracohumeral ligament. The posterior portion merges with the infraspinatus tendon. The region just medial to the convergence of the posterior fibers of the supraspinatus and anterior fibers of the infraspinatus tendons has been called the “posterior rotator interval.” The supraspinatus muscle and tendon abduct and rotate the humerus. This muscle is innervated by the suprascapular nerve.
On axial MR images, the supraspinatus tendon is seen superiorly on sections just below the acromion and clavicle (Fig. 4.2A). On coronal MR images, the musculotendinous junction of the supraspinatus usually lies near the 12-o’clock position above the humeral head, far lateral to the glenoid margin (Fig. 4.2B). The subacromial–subdeltoid (SA-SD) bursa lies above the supraspinatus muscle and tendon and surrounds the cranial and anterior surfaces of all of the rotator cuff muscles. It can contain a few milliliters of fluid in some asymptomatic shoulders but is usually not directly visualized. A rim of fat may line the inferior aspect of the bursa and this fat can be seen in many symptomatic and asymptomatic shoulders (Fig. 4.2B).
The infraspinatus muscle arises from the infraspinatus fossa of the scapula and the bipennate tendon inserts onto the middle facet of the greater tuberosity just posterior to the supraspinatus tendon (Figs. 4.3 and 4.4). It acts to externally rotate and center the humerus. It also functions to depress the humeral head. It resists posterior subluxation of the humeral head in internal rotation (2). The infraspinatus muscle is innervated by the distal suprascapular (subscapular) nerve at the level of the spinoglenoid notch in the posterior scapula.
![]() Figure 4.3 Normal infraspinatus muscle and tendon (IS) as well as teres minor muscle (TM) lie underneath the scapular spine on this oblique coronal T1-weighted image. |
The likelihood and degree of tendon retraction are influenced by local anatomic features. Both the supraspinatus and infraspinatus tendons may have a separate ligament running along the articular surface anteroposterior to the main direction of the tendon in a perpendicular fashion in the region of the critical zone approximately 1 to 2 cm of the footprint (Fig. 4.5). This
structure has been shown to be a ligament, termed the “ligamentum semicirculare humeri,” but is also called the rotator cable (3). The ligament has attachments to the greater and lesser tuberosity anteriorly and is an attachment site for the coracohumeral ligament (4). It is postulated to play a role in the anchoring of the biceps at the rotator interval along with the biceps pulley. Some individuals have larger cables than others and these are termed “cable dominant” tendons.” The cable is also accentuated with aging. It is located just medial to the zone of relative hypovascularity along the articular surface of the rotator cuff, the critical zone. The thinner part of the tendon that continues from the cable to the footprint has been termed the rotator crescent. The cable can also be seen with ultrasound (5). It is thought that the cable and crescent act like a suspension bridge. Most rotator cuff failure occurs in the crescent with limited tearing and retraction beyond the cable in tendons that are cable-dominant. The cable-dominant tendons are easier to repair because they have less retraction. On MRI, the cable will be seen as a normal, slight thickening of the articular surface of the tendon in the region of the critical zone, approximately 1.5 cm medial to the greater tuberosity.
structure has been shown to be a ligament, termed the “ligamentum semicirculare humeri,” but is also called the rotator cable (3). The ligament has attachments to the greater and lesser tuberosity anteriorly and is an attachment site for the coracohumeral ligament (4). It is postulated to play a role in the anchoring of the biceps at the rotator interval along with the biceps pulley. Some individuals have larger cables than others and these are termed “cable dominant” tendons.” The cable is also accentuated with aging. It is located just medial to the zone of relative hypovascularity along the articular surface of the rotator cuff, the critical zone. The thinner part of the tendon that continues from the cable to the footprint has been termed the rotator crescent. The cable can also be seen with ultrasound (5). It is thought that the cable and crescent act like a suspension bridge. Most rotator cuff failure occurs in the crescent with limited tearing and retraction beyond the cable in tendons that are cable-dominant. The cable-dominant tendons are easier to repair because they have less retraction. On MRI, the cable will be seen as a normal, slight thickening of the articular surface of the tendon in the region of the critical zone, approximately 1.5 cm medial to the greater tuberosity.
![]() Figure 4.5 Normal supraspinatus cable and crescent on an oblique coronal fat suppressed T1-weighted image from an MR arthrogram. |
On MRI, the scapular spine lies posterior to the supraspinatus and underneath it, one can visualize the infraspinatus and then the teres minor muscles and tendons (Fig. 4.6). The teres minor muscle arises from the upper two-thirds of the lateral border of the scapula and its tendon inserts onto the inferior (vertical) facet of the greater tuberosity (Figs. 4.1, 4.3, 4.4, and 4.6). The muscle adheres to the posterior glenohumeral capsule. It is an external rotator of the humeral head. The teres minor muscle is innervated by the axillary nerve.
![]() Figure 4.6 Axial T1-weighted image shows the normal attachment of the teres minor tendon on the inferior facet of the greater tuberosity at the very inferior aspect of the joint (arrow). |
The subscapularis muscle originates from the anterior surface of the scapula called the subscapular fossa, and it has nine muscle bellies and multiple tendon slips that attach to the superior aspect of the lesser tuberosity with 40% of the attachment on the humeral neck below the lesser tuberosity (Fig. 4.7). The subscapularis tendon also occasionally has an attachment to the coracoid process. This tendon also sends superficial fibers over the bicipital groove along with fibers of the coracohumeral ligament. The lateral portion of the subscapularis tendon that extends across the bicipital groove is no longer called the transverse humeral ligament (6, 7). The subscapularis tendons adhere to the anterior glenohumeral capsule. The subscapularis muscle internally rotates the humeral head. It also flexes, extends, depresses, and adducts the glenohumeral joint. The subscapularis muscle is innervated by the upper and lower subscapular nerves.
There is a space between the supraspinatus and subscapularis tendons called the rotator interval (Figs. 4.8 and 4.9). The biceps tendon lies anterior and superior in the rotator interval. In addition to the intra-articular portion of the biceps tendon, the rotator interval also contains the superior glenohumeral ligament and the coracohumeral ligament, which form a sling around the biceps before it dips into the intertubercular groove laterally in the rotator interval. This capsular region has several layers. The most superficial layer contains fibrofatty tissue. The next layer is composed of the coracohumeral ligament (Fig. 4.7B). The coracohumeral ligament is a triangular structure with two fascicles that originate from the lateral aspect of the coracoid and
attach to the lesser tuberosity of the humerus. The coracohumeral ligament restricts anterior and superior movement of the humeral head and related structures. The superior glenohumeral ligament arises from the supraglenoid tubercle and fuses with the coracohumeral ligament to insert on the lesser tuberosity. Deep to these structures lies the intra-articular portion of the long head of the biceps tendon.
attach to the lesser tuberosity of the humerus. The coracohumeral ligament restricts anterior and superior movement of the humeral head and related structures. The superior glenohumeral ligament arises from the supraglenoid tubercle and fuses with the coracohumeral ligament to insert on the lesser tuberosity. Deep to these structures lies the intra-articular portion of the long head of the biceps tendon.
![]() Figure 4.8 The rotator interval lies between the supraspinatus and subscapularis muscles and tendons. It contains the biceps tendon, superior glenohumeral ligament, and coracohumeral ligament. |
The supraspinatus tendon, subacromial bursa, and the long head of the biceps tendon lie within the coracoacromial outlet (Fig. 4.10). The subacromial bursa serves as a buffer between the rotator cuff and coracoacromial arch and is located below the acromion, coracoacromial ligament, and above the rotator cuff. The superior aspect of this outlet, the coracoacromial arch, includes the acromion, which is an anterolateral extension of the scapular spine, the anterior third of the coracoid, and the coracoacromial ligament (Figs. 4.11 and 4.12). The thin, low-signal–intensity coracoacromial ligament is seen above the supraspinatus muscle extending medially to the coracoid on sagittal images (Fig. 4.12). This ligament limits anterior and superior migration of the humeral head during abduction. Some researchers believe that thickening of the coracoacromial ligament may play a role in impingement syndrome as discussed subsequently.
The acromion is viewed on sagittal images with the shape and orientation assessed lateral to the acromioclavicular (AC) joint (Fig. 4.12). On these images, one looks for the presence of anterior hooking and osteophytes, which can lead to impingement of the rotator cuff tendons. The most lateral oblique sagittal images show the low-signal–intensity tendons in cross-section (Fig. 4.13). The osseous coracoacromial arch is no longer seen.
Imaging Workup of Rotator Cuff Disease
Different imaging techniques are available for evaluation of rotator cuff abnormalities: conventional radiographs, sonography, arthrography, and MRI. Each technique has advantages and disadvantages.
Conventional Radiographs
Early diagnosis and treatment of impingement syndrome may prevent progression of soft tissue abnormalities, especially rotator cuff tears. Conventional radiography and arthrography have been disappointing in the early diagnosis of impingement. Surrounding osseous changes such as a subacromial enthesophyte, laterally downsloping or low-lying acromion, and AC joint undersurface osteophytes can be identified; however, these can also be present in the absence of rotator cuff disease.
An anteroposterior (AP) radiograph can be obtained with internal and external rotation or in the plane of the scapula (Grashey view) (Fig. 4.14). The Grashey view allows for evaluation of the glenohumeral joint without overlap between the humeral head and the glenoid. One can assess for narrowing of the joint. The presence of calcific tendinitis, also called hydroxyapatite deposition
disease (HADD), can also be determined. Adding 30 degrees of caudal angulation of the central ray to an AP radiograph best demonstrates anterior acromial enthesophytes (8) (Fig. 4.15). A modified transcapular lateral view obtained at 10 to 15 degrees of caudal angulation, termed the “supraspinatus outlet view,” is often used to show the anterior inferior aspect of the acromion (9) (Fig. 4.15). This view is helpful for identifying a type III hooked acromion and subacromial enthesophytes, which have a higher predictive value for rotator cuff pathology. Fluoroscopy can also be helpful for detection of subacromial enthesophytes (10). They are best seen on spot films obtained with the patient standing and leaning forward toward the image intensifier.
disease (HADD), can also be determined. Adding 30 degrees of caudal angulation of the central ray to an AP radiograph best demonstrates anterior acromial enthesophytes (8) (Fig. 4.15). A modified transcapular lateral view obtained at 10 to 15 degrees of caudal angulation, termed the “supraspinatus outlet view,” is often used to show the anterior inferior aspect of the acromion (9) (Fig. 4.15). This view is helpful for identifying a type III hooked acromion and subacromial enthesophytes, which have a higher predictive value for rotator cuff pathology. Fluoroscopy can also be helpful for detection of subacromial enthesophytes (10). They are best seen on spot films obtained with the patient standing and leaning forward toward the image intensifier.
![]() Figure 4.15 Normal supraspinatus outlet view allows one to evaluate the shape of the acromion and the acromioclavicular joint (arrow). |
The conventional radiograph is somewhat limited in evaluation of the rotator cuff. It can be pathognomonic
for a chronic tear if there is elevation of the humeral head with either acromiohumeral articulation or an acromiohumeral distance of 6 mm or less (11) (Fig. 4.16), but this is not commonly seen in patients with acute rotator cuff tears. Concavity of the undersurface of the acromion as a result of molding by the humeral head, called “faceting” or “acetabularization,” is also suggestive of a rotator cuff tear. Well-circumscribed lytic lesions and sclerosis of the greater tuberosity occur frequently with rotator cuff tears, but these findings are nonspecific.
for a chronic tear if there is elevation of the humeral head with either acromiohumeral articulation or an acromiohumeral distance of 6 mm or less (11) (Fig. 4.16), but this is not commonly seen in patients with acute rotator cuff tears. Concavity of the undersurface of the acromion as a result of molding by the humeral head, called “faceting” or “acetabularization,” is also suggestive of a rotator cuff tear. Well-circumscribed lytic lesions and sclerosis of the greater tuberosity occur frequently with rotator cuff tears, but these findings are nonspecific.
Conventional Arthrography
Glenohumeral joint arthrography is an invasive study limited to demonstration of partial-thickness undersurface and full-thickness tears of the rotator cuff and does this with high sensitivity and specificity (12). It cannot demonstrate partial-thickness tears in the substance or along the superior surface of the tendon and cannot directly evaluate important soft tissue structures in and around the glenohumeral joint that may be causing or contributing to the symptomatic shoulder, including labrocapsular abnormalities, bursitis, and paralabral cysts (13, 14). Furthermore, arthrography does not allow for evaluation of the muscles of the rotator cuff or marrow abnormalities. At this stage, there is no real reason to perform conventional arthrography.
Ultrasound
Ultrasound is an operator-dependent technique that can demonstrate abnormalities of the tendons and muscles of the rotator cuff, bursa, and long head of the biceps tendon (15–22). It allows for noninvasive evaluation of the muscles and tendons of the rotator cuff. Ultrasound can be used with high sensitivity and specificity when scanned and interpreted by a highly experienced
sonographer using state-of-the-art ultrasound equipment. Several groups have published results that demonstrate diagnostic sensitivity and specificity above 90% for detecting partial- and full-thickness rotator cuff tears (23–26). Fatty atrophy of the muscles can also be visualized with ultrasound (27, 28). Changes in the tendon with shoulder motion as seen with subacromial impingement can be directly assessed using ultrasound (29, 30). Ultrasound is not able to evaluate many abnormalities of
the shoulder that might be causing a rotator cuff tear or contributing to shoulder pain, including lesions associated with instability such as labral and capsular disruption. It has been shown to demonstrate greater tuberosity fractures (31) but is limited in its evaluation of the deeper osseous structures. A normal ultrasound in a patient with impingement symptoms should be followed up with further imaging examinations to detect changes associated with the osseous structures of the coracoacromial arch and secondary causes of impingement, including lesions of the labrum and glenohumeral ligaments associated with instability.
sonographer using state-of-the-art ultrasound equipment. Several groups have published results that demonstrate diagnostic sensitivity and specificity above 90% for detecting partial- and full-thickness rotator cuff tears (23–26). Fatty atrophy of the muscles can also be visualized with ultrasound (27, 28). Changes in the tendon with shoulder motion as seen with subacromial impingement can be directly assessed using ultrasound (29, 30). Ultrasound is not able to evaluate many abnormalities of
the shoulder that might be causing a rotator cuff tear or contributing to shoulder pain, including lesions associated with instability such as labral and capsular disruption. It has been shown to demonstrate greater tuberosity fractures (31) but is limited in its evaluation of the deeper osseous structures. A normal ultrasound in a patient with impingement symptoms should be followed up with further imaging examinations to detect changes associated with the osseous structures of the coracoacromial arch and secondary causes of impingement, including lesions of the labrum and glenohumeral ligaments associated with instability.
Magnetic Resonance Imaging
MRI is able to demonstrate osseous and soft tissue abnormalities of the shoulder in any plane with high soft tissue contrast. The status of the tendons, muscles, marrow, and labrocapsular structures can be assessed. Normal tendons and those with a full-thickness tear are easily identified on routine noncontrast MR studies. The most sensitive and specific study, especially for partial undersurface tears of the rotator cuff, is one obtained with some native fluid, saline, or contrast in the joint. The study may be limited for this evaluation if fluid is not present in the glenohumeral joint. It is preferable to use a high-field MRI unit for evaluation of rotator cuff tears, especially partial tears. Recently, MRI of the shoulder at 3.0 T has been shown to be sensitive and specific for small full-thickness and partial-thickness tears when correlated with arthroscopy (32). Findings at MRI can help the referring clinician determine if the rotator cuff is abnormal in cases in which conservative treatment is unsuccessful.
Shoulder Impingement
Shoulder impingement is a common cause of shoulder pain in adults. It is divided into external and internal categories. External impingement is produced by structural changes outside of the joint and includes primary and subcoracoid impingement. Internal impingement, also called secondary extrinsic impingement, is caused by rotator cuff and capsular abnormalities and is divided into posterosuperior, anterosuperior, and anterior forms that are discussed more completely in the chapter on shoulder instability.
Primary Extrinsic Impingement
Primary extrinsic impingement syndrome is a common, progressively painful compression of the supraspinatus tendon, subacromial–subdeltoid bursa, and long head of the biceps tendon between the humeral head and the coracoacromial arch. The coracoacromial arch is comprised of the undersurface of the anterior third of the acromion, the coracoacromial ligament, the anterior third of the coracoid process, the AC joint, and the distal clavicle (Figs. 4.10 and 4.11). The pain occurs when the arm is raised into a position of abduction and external rotation or is elevated forward and internally rotated (33). Hypovascularity in the supraspinatus tendon, mechanical wear, acute trauma, and repetitive microtrauma all serve as potential causes or effects of primary extrinsic impingement.
This form of impingement is often seen in young athletes, especially those who engage in sports that involve overhead arm movement such as tennis, football, and baseball. It is also seen in persons whose occupations require overhead motion and in older individuals with degenerative changes of the coracoacromial arch. A classic primary extrinsic type of impingement results from entrapment of the supraspinatus tendon by the coracoacromial arch, which is caused by variations in the architecture of the coracoacromial arch, including one or more of the following: a subacromial enthesophyte, anteriorly hooked acromion, downsloping or low-lying acromion, inferior AC joint osteophytes, os acromiale, or a thickened coracoacromial ligament.
Stages of Primary Extrinsic Impingement in the Supraspinatus Tendon
In 1972, Neer (33) described the technique of anterior acromioplasty to relieve the symptoms of impingement. He hypothesized that abnormalities of the anterior third of the acromion, such as enthesophytes, along the undersurface of the acromion and excessive traction by the coracoacromial ligament result in progression of degenerative changes of the supraspinatus tendon.
The area of the tendon that is most frequently affected by impingement is called the critical zone, a region first described by Codman (34). This is an area approximately 1 cm from the tendon insertion on the greater tuberosity. It is believed that mechanical impingement to the critical zone leads to tendinosis that represents the first stage of the degenerative process.
Neer (35) subsequently described three stages of impingement involving the critical zone of the supraspinatus tendon. The first stage is that of reversible edema and hemorrhage usually seen in patients younger than 25 years of age. The second stage is fibrosis and tendinitis resulting from chronic trauma, and the third stage is degeneration and rupture of the supraspinatus tendon, often associated with osseous changes and usually seen in patients older than 40 years of age. Neer also demonstrated that a laterally placed or shallow bicipital groove caused the long head of the biceps tendon to undergo impingement by the anterior third of the acromion, resulting in eventual tendinitis and rupture.
Clinical Assessment of Primary Extrinsic Impingement
An impingement injection test, first described by Neer (35), is a tool to assess the presence of impingement. The examiner elevates the humerus of the patient with one hand while preventing scapular rotation with the other. Pain is produced when the greater tuberosity of the humeral head impinges against the acromion. The pain is relieved by injection of an anesthetic agent into the subacromial space. Caution should be taken when using this
test alone for the diagnosis of impingement, because other causes for the pain may also be relieved by the injection.
test alone for the diagnosis of impingement, because other causes for the pain may also be relieved by the injection.
Rotator cuff disease associated with primary extrinsic impingement may include tendinosis, partial-thickness tears, and full-thickness tears. MRI characterizes the degree of tendon pathology and displays tendon retraction and associated muscle atrophy or fatty infiltration. MRI demonstrates abnormal distention of the subacromial–subdeltoid bursa or subcoracoid bursa. MRI can also show damage to the rotator interval, located in the region devoid of tendons between the supraspinatus and subscapularis tendons. There may be synovitis, tears of the superior glenohumeral and coracohumeral ligaments and tendinosis, subluxation, and/or tears of the biceps tendon in this region.
Osseous and Soft Tissue Abnormalities Associated With Primary Extrinsic Impingement
The space between the humeral head and the coracoacromial arch is termed the “impingement interval” and anything that narrows this arch on abduction can produce impingement. MRI can aid in detecting osseous and soft tissue abnormalities that may predispose to or be the result of rotator cuff impingement (38, 39). The soft tissue abnormalities in the supraspinatus tendon and subacromial bursa such as tendinosis, partial- and full-thickness tears, and distension and synovitis involving the bursa are discussed in the next section. The osseous lesions include morphologic abnormalities of the coracoacromial arch, which consists of the anterior third of the acromion, the AC joint, the coracoacromial ligament, and the coracoid process. Displaced, ununited, or malunited fractures of the humeral head, especially involving the greater tuberosity, can also contribute to impingement. It is important to remember that many of these anatomic features may be present in asymptomatic shoulders, and clinical correlation is imperative (38–42). Additionally, the diagnosis of impingement is based on clinical criteria and therefore should not be made from static MRI studies.
Osseous and Cartilaginous Abnormalities
Although controversial, many believe that variations in the architecture of the coracoacromial arch, including one or more of the following, are related to this form of impingement. Osseous changes that can lead to primary extrinsic impingement are listed in Table 4.1 and include subacromial enthesophytes and osteophytes along the inferior aspect of the AC joint (33, 43). Capsular hypertrophy may accompany the AC joint osteophytes and degeneration. Type III hooked acromion (traction enthesophytes of the coracoacromial ligament at its origin from the acromion or a congenitally hooked acromion) along with anteroinferior and inferolaterally downsloping and the low-lying acromion are believed by some researchers to predispose to impingement of the supraspinatus tendon by narrowing the acromiohumeral distance and causing abnormal impaction on the soft tissues in the coracoacromial outlet (44–47). Some believe that an os acromiale can also predispose to impingement (48). Many of these anatomic features may be present in asymptomatic shoulders, and clinical correlation is imperative (38–42).
Table 4.1 Osseous abnormalities that may be associated with supraspinatus tendon impingement | |||
---|---|---|---|
|
The subacromial enthesophyte may predispose to narrowing of the coracoacromial outlet leading to rotator cuff tears. This is not a universal theory because there have been studies showing that enthesophyte formation may be a secondary phenomenon in the presence of established rotator cuff tear (37). The subacromial enthesophyte can be definitively diagnosed on MRI if downward projection of marrow signal is seen below the expected location of the cortex (Fig. 4.17). If the enthesophyte contains mostly cortical bone, it will be low signal intensity. This type of enthesophyte is more difficult to distinguish from the low-signal–intensity deltoid tendon (49) and the thickened coracoacromial ligament, which insert on the inferolateral and inferomedial acromion, respectively. It is helpful to correlate the MR findings with conventional radiographs when the diagnosis is uncertain.
A specific subset of subacromial enthesophyte is a subacromial “keel” spur (Fig. 4.18). This ossification extends from the inferior acromion and is shaped like the keel of a sailboat. It is seen more often in young and middle-aged women for unknown reasons. Both the subacromial enthesophyte and the subset keel spur can result in bursal-sided tears of the supraspinatus tendon.
![]() Figure 4.18 The “keel spur” is a specific type of subacromial enthesophyte that is shaped like a sailboat keel (arrow) seen on this oblique coronal T1-weighted image. |
In 1986, Bigliani et al. (50) studied the shape of the acromion in 140 cadaver shoulders to determine the relationship of acromial configuration to full-thickness rotator cuff tears (Fig. 4.19). They defined three different acromial shapes; type I configuration with a flat undersurface was present in 18.6% of cases, 42% had type II acromion with a curved undersurface, and 38.6% had type III acromion with an anterior hook. In studies correlating acromial shape to surgical or arthrographic results, Morrison and Bigliani (44) and Bigliani et al. (50) demonstrated that 70% and 80%, respectively, of the rotator cuff tears were associated with type III acromion. The remaining patients with rotator cuff tears had type II acromion. Only 3% of the type I flat acromions were associated with a rotator cuff tear.
A fourth type of acromial shape with a convex inferior surface was first described by Farley et al. (51) and Vanarthos et al. (52) (Fig. 4.19). This configuration is relatively uncommon and there is potential for narrowing the osseous acromial outlet near the midposterior portion of the distal acromion rather than at the anterior portion of the acromion near the AC joint. There has yet been no definite correlation between this type IV acromion and impingement.
Since Morrison and Bigliani’s study, there has been some difference of opinion regarding the significance of acromial morphology and its relationship to supraspinatus pathology (53, 54). In any case, when evaluating acromial morphology on MRI, it is best to review the images in the oblique sagittal plane on MR images located lateral to the AC joint. There can be a variation in the shape of the acromion. It has been suggested that the first and/or second image just lateral to the AC joint be used for typing of the acromion (55). In our practice, we summate the images lateral to the AC joint and then decide on the type based on finding a hook (type III) (Fig. 4.20A), a curve without a hook (type II) (Fig. 4.20B), a flat acromion (type I) (Fig. 4.20C), or a convex acromion (type IV) (Fig. 4.20D). For example, if it is flat and curved on different images, it is called type II. If
there is an anterior hook on any image, it is called type III.
there is an anterior hook on any image, it is called type III.
The hooked acromion can be congenital or acquired (44, 50). Some researchers believe that type III anteriorly hooked configuration increases in frequency with increasing age (56) and that this shape can develop as a result of mineralizaton of the acromial attachment of the coracoacromial ligament in the form of chondroid metaplasia and enchondral bone formation, which may be caused by traction loading of the coracoacromial arch by degeneration and tearing of the rotator cuff. Others have found no relationship between acromial type and age (46, 57). The study by Getz et al. (57) showed that type III acromion was more common in males and that acromial morphology was symmetric in 70.7% of 191 pairs of cadaveric acromion without an increased incidence of subacromial enthesophytes in patients with type II and type III acromion. In one study that looked at acromial morphology on oblique sagittal MR images, patients with rotator cuff tears had a significantly increased prevalence of hooked acromion compared with control patients (62% versus 13%, p <0.001) and there was a greater prevalence of hooked acromion in the group with impingement (30%, p = 0.17) (58). Farley et al. (51) also found a correlation between the anteriorly hooked acromion seen on MRI and the presence of clinical impingement. One group concluded that acromial shapes influence the degree of supraspinatus tearing but that the correlation between rotator cuff tears and an anteriorly hooked acromion is not as strong as suggested by Morrison and Bigliani. (44) Several studies have found MR assessment of acromial shape to be confusing. Haygood et al. (59) showed significant variability between MR readers in identifying a particular acromial morphology. These investigators did not use a routine location for assessment of acromial shape. Peh et al. (60) concluded that apparent acromial shape is sensitive to minor changes in MR section viewed. There was poor correlation between morphology identified during radiographic and MR assessment.
An acromion that slopes anteroinferiorly (Fig. 4.20C) or inferolaterally (Fig. 4.21) or is low-lying with respect to the distal clavicle (Fig. 4.22) has potential to narrow the coracoacromial outlet (39, 45, 61). The
lateral aspect of the normal acromion is nearly horizontal or upward-sloping from posterior to anterior. If the anterior acromion is more caudal in relation to the posterior portion, some researchers believe that there is greater risk of symptomatic anterior acromial impingement (61). This type of configuration would produce impingement primarily on the midportion of the supraspinatus tendon in the area of the critical zone. In one study, however, there was lack of association between an inferiorly sloping acromion on the oblique coronal sequence and the presence of a rotator cuff tear (51). Such downward sloping of the acromion also may cause impingement of the superior aspect of the subscapularis tendon (62, 63). An inferior–laterally sloping acromion appears to be associated with lateral supraspinatus injury near the greater tuberosity insertion, especially in patients who perform forceful abduction of the shoulder such as pitchers, divers, and swimmers (64).
lateral aspect of the normal acromion is nearly horizontal or upward-sloping from posterior to anterior. If the anterior acromion is more caudal in relation to the posterior portion, some researchers believe that there is greater risk of symptomatic anterior acromial impingement (61). This type of configuration would produce impingement primarily on the midportion of the supraspinatus tendon in the area of the critical zone. In one study, however, there was lack of association between an inferiorly sloping acromion on the oblique coronal sequence and the presence of a rotator cuff tear (51). Such downward sloping of the acromion also may cause impingement of the superior aspect of the subscapularis tendon (62, 63). An inferior–laterally sloping acromion appears to be associated with lateral supraspinatus injury near the greater tuberosity insertion, especially in patients who perform forceful abduction of the shoulder such as pitchers, divers, and swimmers (64).
![]() Figure 4.22 Low-lying acromion. The acromion is laterally downsloping as well as low-lying with respect to the distal clavicle on this oblique coronal T1-weighted image (arrow). |
Osteophytes and osseous or fibrous callus under the AC joint from degenerative change can predispose to impingement (33, 43). The mechanism is similar to sawing a log with the supraspinatus tendon simulating a log and the osteophytes cutting the tendon, especially when the patient abducts the shoulder. AC joint osteoarthrosis is not specific for impingement and can be seen with high prevalence in asymptomatic individuals (65). It is also useful to mention if the osteophyte is indenting the supraspinatus muscle and tendon (Fig. 4.23).
![]() Figure 4.23 Acromioclavicular joint osteophyte indenting the supraspinatus musculotendinous junction on (A) oblique coronal and (B) sagittal T1-weighted image (arrow). |
The os acromiale is an accessory ossification center along the outer aspect of the acromion that has failed to fuse to the acromion by the age of 22 years. It is seen in 1% to 15% of the population, but it is not known how many of these are symptomatic (66–69). The unfused segment can be united to the remaining acromion through articulation composed of fibrous tissue, cartilage (synchondrosis), periosteum, or synovium (48, 66). The os acromiale is classified as a pre-, meso-, meta-, or basiacromion based on its regions of articulation with the acromion (48) (Figs. 4.24 and 4.25). The meta-acromion is the most proximal center and the meso- and preacromion are progressively more distal. Most are of the meso- or meta-acromial type (70). The os acromiale is seen in the contralateral shoulder 60% of the time (71).
An os acromiale is more mobile than an acromion without accessory ossification centers. Motion is increased at the deltoid tendon insertion along the lateral inferior aspect of the ossification center. Contraction of the deltoid muscle can pull down on the lateral aspect of the acromion, creating a hinge effect that narrows the subacromial outlet with resultant impingement and tears of the rotator cuff. The coracoacromial ligament also commonly attaches to the unfused segment. Hyperostosis, or a stepoff, may develop along the undersurface of the os acromiale at its site of failed union, leading to impingement and eventual tear of the supraspinatus tendon (48, 68, 70, 72) (Fig. 4.26). Although in the past, the surgical and radiologic literature has confirmed the association between os acromiale and impingement and rotator cuff pathology (48, 70, 73), there is some controversy about this (72). Simply identifying the os acromiale does not
implicate it as the source of shoulder pain or rotator cuff disease.
implicate it as the source of shoulder pain or rotator cuff disease.
Careful preoperative imaging evaluation is necessary to exclude the presence of an os acromiale. This ossification center may be difficult to find on conventional radiographs (66, 68, 69, 74). It is best seen on an axillary view that displays the long axis of the acromion (Fig. 4.25). It can also be seen on an axial computed tomography (CT) or on an MR study, in which it is important to include high axial sections that demonstrate the entire acromion. The os acromiale is easiest to identify in the axial plane, although it can usually be noticed in all three planes, especially if it is larger. The radiologist should be careful not to miss the synchondrosis, which can have an appearance similar to the neighboring AC joint. This is especially easy to do on the oblique sagittal images. MRI has an advantage over conventional radiographs and CT in that it can reveal underlying, frequently associated rotator cuff tendon abnormalities and abnormal signal intensity around the os acromiale.
It is important to report the presence of an os acromiale, especially in patients who are being considered for subacromial decompression for impingement (70). The os acromiale has the potential for even more mobility and there can be further weakening of the synchondrosis after this procedure, leading to more impingement (33, 70). When an undiagnosed os acromiale is treated with acromioplasty, patients come back with continued symptoms of impingement months to years later.
In addition to possibly causing primary extrinsic impingement of the supraspinatus, an unstable or stressed os acromiale can cause shoulder pain, even in children or adolescents. On MRI, it is common to see abnormal marrow signal with T2 hyperintensity around the synchondrosis (Fig. 4.27). The synchondrosis may also be abnormally elevated in signal intensity (Fig. 4.27). If the pain does not diminish, small ossification centers are often excised with reattachment of the deltoid; larger or unstable ones may be fused to the rest of the acromion.
Other osseous causes of primary extrinsic impingement include prominent greater tuberosity caused by fracture, malunion, or nonunion and Paget disease. Paget disease can produce enlargement of the acromion, resulting in narrowing of the coracoacromial outlet (75
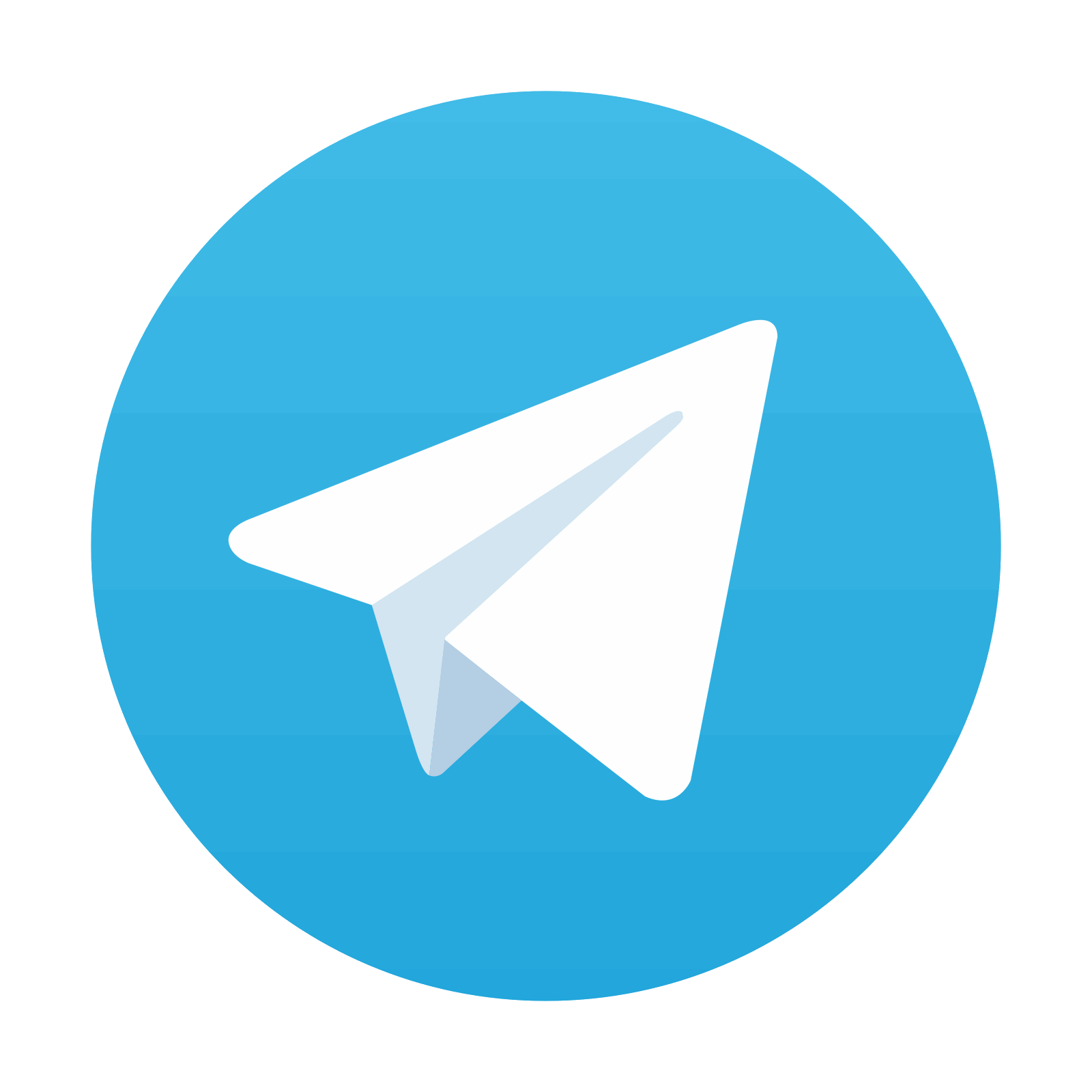
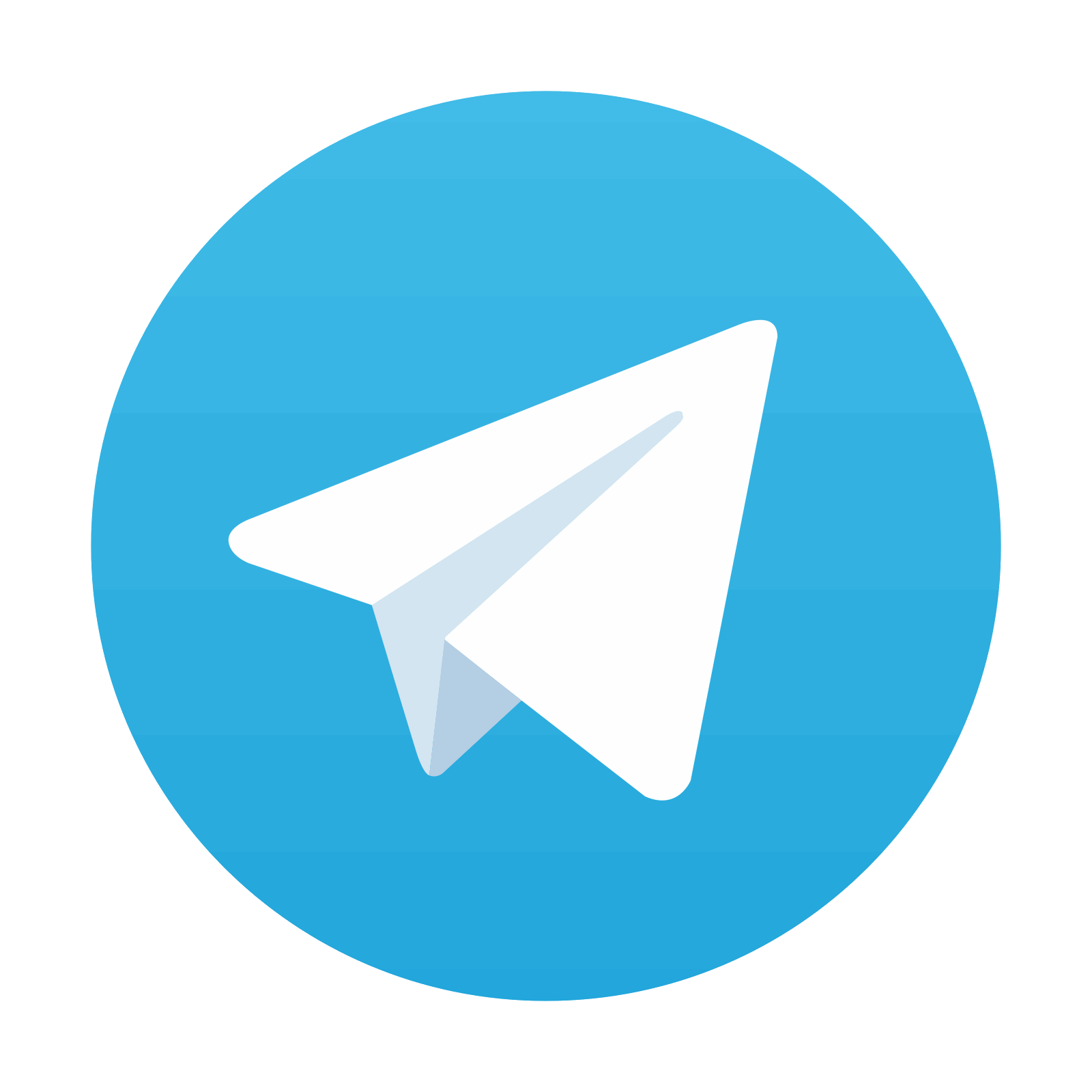
Stay updated, free articles. Join our Telegram channel

Full access? Get Clinical Tree
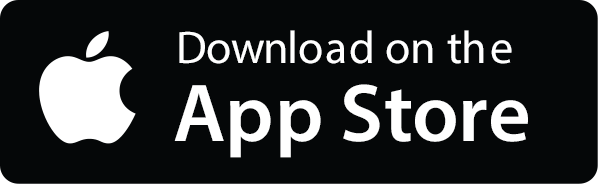
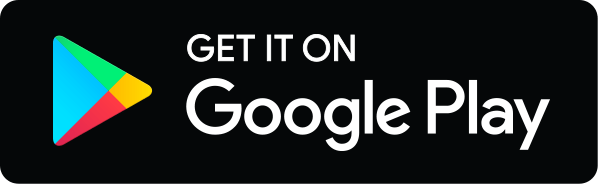
