The scope of clinically relevant myocardial ischemic disease renders it one of the most important problems facing modern medicine. Risk stratification, diagnosis, management, and monitoring of patients with suspected or known ischemic disease heavily depend on imaging. Broadly speaking, the goals of imaging in ischemic heart disease can be categorized as anatomic or functional evaluation. Coronary computed tomography (CT) angiography has repeatedly been shown to have excellent diagnostic capabilities in the anatomic assessment of ischemic heart disease, specifically the detection of coronary artery atherosclerosis and stenosis. The physiologic relevance of coronary artery disease, however, is generally considered more important in establishing prognosis and management plans. Although myocardial morphology provides diagnostic clues in ischemic disease, dedicated myocardial evaluations generally focus on functional assessments, with specific goals depending on the clinical situation.
The diagnosis of acute and chronic ischemia can be made with myocardial functional imaging; more important, differentiation of viable from nonviable tissue is available and extremely important in guiding clinical management. Several strategies are used to determine tissue viability, depending on the clinical scenario; however, most of these rely on time-resolved imaging, myocardial perfusion imaging, delayed enhancement imaging, or a combination thereof. Therefore, CT has lagged behind other modalities in dedicated myocardial analyses. Temporal resolution and contrast resolution are inferior to those of other modalities, and the inevitable exposure to iodinated contrast material and ionizing radiation poses risks to the patient not associated with some techniques. However, the rapidly increasing rate of CT scans encompassing the heart, whether in a dedicated cardiac protocol or otherwise, necessitates knowledge of typical CT imaging findings in ischemic disease; furthermore, emerging techniques are showing progress in expanding the role of CT in physiologic assessments. Future refinement of these techniques could lead to a single-modality integrative evaluation of cardiac structure and function, the ultimate goal of cardiac imaging.
This chapter reviews the spectrum of imaging findings of myocardial ischemic disease in established CT applications, including routine thorax CT and dedicated cardiac CT angiography studies. In addition, technical considerations, imaging findings, and potential applications of experimental and emerging techniques are explored.
Noncardiac Computed Tomography Examinations
Although the value of non–electrocardiogram (ECG)-gated CT studies in assessing ischemic heart disease is limited, the sheer volume of such studies combined with the prevalence of the disease in current society necessitates knowledge of the possible imaging manifestations of acute and chronic ischemic disease. In addition, advances in scanner technology have decreased scan acquisition times sufficiently to allow assessment of the myocardium on some non–ECG-gated studies. All examinations that encompass the heart should be assessed for image quality and motion artifacts, and the myocardium should not be ignored when adequate assessment is possible.
Suggestions of acute myocardial infarction on nongated CT studies are mainly found outside the myocardium itself. Pulmonary vascular congestion suggests acute left ventricular dysfunction. Assessment of cardiac chamber size is inaccurate, but a grossly enlarged left atrium in severe acute left ventricular failure may be apparent. Similarly, right ventricular failure from an isolated right ventricular myocardial infarction is rare, but a dilated right ventricular chamber, right atrial chamber, or systemic veins may be apparent, and contrast reflux deep into the hepatic or azygos veins may be present. Contrast-enhanced studies may display subendocardial attenuation deficits in normal-thickness myocardium, findings suggestive of acute infarction if the contrast medium bolus is timed correctly and motion artifacts are not severe; however, quantifying the size or severity of defects is difficult.
Major structural complications of myocardial infarction may also be apparent on non–ECG-gated scans. Left ventricular aneurysms or pseudoaneurysms may be seen as nonspecific morphologic abnormalities on unenhanced scans, whereas most contrast-enhanced studies should allow one to diagnose but not fully characterize wall deformities. Left ventricular free wall rupture or septal wall rupture may also be apparent on contrast-enhanced studies, but again this varies depending on image quality and the nature of the abnormality.
Manifestations of chronic myocardial infarction are also apparent on many noncardiac CT protocols of the thorax. Left ventricular dilation should be noted, along with other signs of remodeling and functional inadequacy, such as an enlarged left atrial chamber, pulmonary venous hypertension, enlarged hilar lymph nodes, thickening of the pulmonary interlobular septa, bronchovascular bundle thickening, or ground glass opacities in dependent portions of the lungs. Again, depending on image quality, assessment of the myocardium itself may be possible. The interpreting physician should look for and comment on focal subendocardial fatty metaplasia, linear myocardial calcifications, and myocardial thinning, all of which are especially suggestive of remote infarction if they are present in a specific vascular territory ( Fig. 30-1 ).
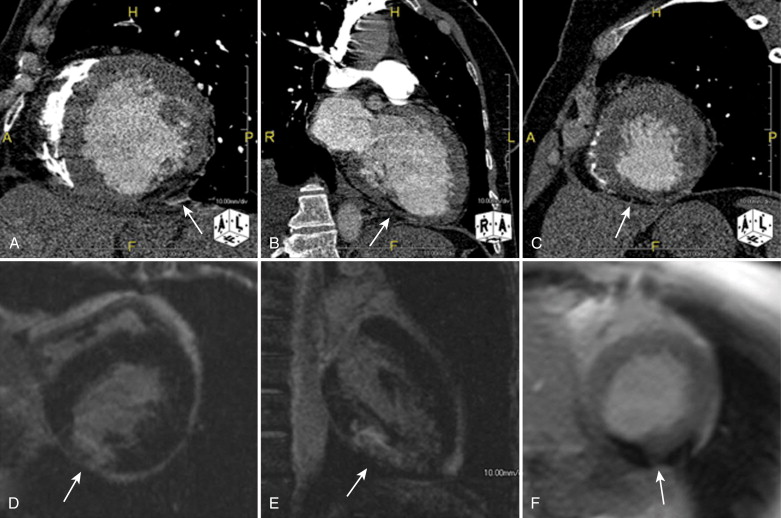
Cardiac Computed Tomography
The rapid rise of cardiac CT largely sprang from concomitant advances in the underlying technology, namely, decreased image acquisition times and increased detector coverage, the combination of which provided dramatic improvements in temporal resolution and isotropic submillimeter spatial resolution. These advances allow robust assessment of the coronary arteries, with diagnostic accuracy for the detection of stenoses that approaches that of the gold standard, invasive coronary angiography. Furthermore, the technique has other inherent advantages that make it attractive in the diagnostic workup of cardiac disease. In contrast to invasive angiography, extraluminal coronary artery morphology, such as wall remodeling, is visualized. In addition, valuable information on the myocardium itself is available, both morphologic and, to a limited extent, functional.
Arterial Phase Imaging
Early researchers in CT recognized that contrast-enhanced scans were able to show areas of decreased myocardial attenuation that could be related to decreased blood flow to that area ( Fig. 30-2 ). However, relatively poor temporal and spatial resolution limited the utility of CT for dedicated myocardial evaluation. Technical improvements combined with the increasing clinical use of coronary CT angiography revived interest in arterial phase myocardial imaging, especially given that routine coronary CT angiography scan protocols provide this information with no increase in radiation exposure, contrast dose, or study cost. The rationale behind the technique is quite simple: contrast enhancement reflects myocardial blood volume, and both animal and human studies have shown that decreased contrast enhancement reflects decreased blood flow. This characteristic may allow the identification of hemodynamically significant coronary artery stenosis, acute infarction, or chronic scarring.
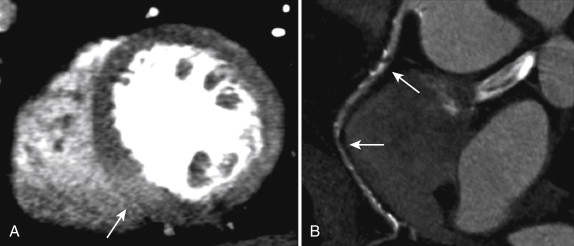
Arterial phase imaging, or first-pass perfusion, requires no modification of standard coronary CT angiography acquisition or reconstruction. Contrast material bolus timing is important, but both bolus tracking and test bolus techniques have been successfully applied. Some researchers have suggested the use of a lower energy setting (e.g., 100 kV), to increase contrast resolution. However, this should not be used unless it is in accordance with the intended coronary CT angiography protocol because myocardial evaluation is a secondary goal. Furthermore, although this technique has been shown to increase the specificity of identifying perfusion defects, it does not clearly enhance diagnostic accuracy and may decrease specificity compared with higher energy settings.
The evaluation of arterial phase myocardial CT images has not been standardized. Experienced researchers advocate starting with a narrow window and level (center, 100 to 150 Hounsfield units [HU], and width, 150 to 200 HU) and adjusting as desired. Individual diagnostic accuracy varies with the threshold used to call positive findings. In general, defects should occupy a known vascular territory. Attenuation values should be lower than 50% (in Hounsfield units) those of remote normal myocardium, although some researchers have used an attenuation difference of 20 HU to define a positive finding. Most studies have found normal myocardium to display attenuation values between 100 and 120 HU, whereas ischemic areas average 40 to 60 HU. As expected, the thickness of defects occupies a gradient between subendocardial and transmural, depending on the severity of the ischemia, and other patterns (e.g., subepicardial) should arise suspicion of nonischemic entities or artifact. Some examiners advocate comparison of end-systolic and end-diastolic images, with only those abnormalities seen on both regarded as true-positive results; this comparison is not possible in prospectively triggered studies. Finally, advanced visualization software, namely perfusion-weighted color mapping, is useful and has been shown to increase sensitivity ( Fig. 30-3 ).
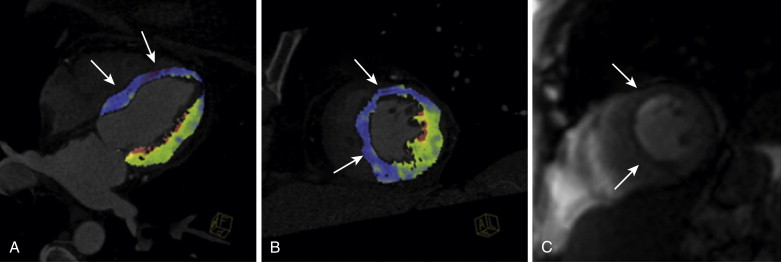
The ultimate goals of arterial phase CT are the same as other modalities used for myocardial analysis: risk stratification, prognostication, and better patient outcomes through improved clinical management decisions. These goals require appropriate characterization of defects regarded as true-positive results. Ideally, nonviable tissue should be distinguished from viable myocardium in the settings of acute and chronic disease. Unfortunately, no way exists to predict the recoverability of a given myocardial segment definitively based on arterial phase imaging alone because hibernating, stunned, vulnerable, and irreversibly damaged myocardium all may demonstrate areas of decreased attenuation. However, correlation with lesions seen on coronary artery analysis and wall motion abnormalities seen on time-resolved left ventricular functional analysis can add evidence of significant disease. In addition, the transmural extent of defects has been shown to correlate with functional recovery and should be reported. The volume of hypoperfused myocardium in relation to overall left ventricular volume may also provide useful information. Relative attenuation differences among defects can suggest acute versus chronic disease when fatty infiltration accompanies myocardial scarring. These areas may have negative attenuation values, whereas acute lesions should not display values lower than those found in unenhanced edematous myocardium. Chronic infarction is also suggested by relative thinning of the hypoattenuating segment.
Research on diagnostic accuracy is limited but has provided sensitivity values ranging from 67% to 100% and specificity values ranging from 57% to 97%. The technique has been evaluated in the setting of both acute and chronic infarction and compared with clinical, magnetic resonance imaging (MRI), and single photon emission CT (SPECT) reference standards, with similar results. Diagnostic accuracy figures highly depend on the methodology used for defining positive results by the gold standard. Several studies showed that the size of perfusion defects is underestimated in comparison with MRI and histopathologic examination; however, at least one study showed good correlation with SPECT in quantifying the volume of hypoperfused myocardium. Early work also suggested that the transmural extent of disease may be accurately assessed. Unfortunately, no currently available data suggest the appropriate integration of the technique into risk stratification or clinical management schemes; however, this integration should become possible as the clinical use of coronary CT angiography continues to expand.
Clear guidelines for the acquisition and evaluation of arterial phase myocardial CT imaging do not exist. However, the information is inherently available with any coronary CT angiography acquisition and should be considered when reporting findings. Although differentiation of viable from nonviable myocardium is often impossible without supplementary techniques (e.g., time-resolved, rest-stress, or delayed phase imaging; see later), clues to suggest acute versus chronic disease are often present, and correlation with angiographic and functional findings is useful. Future work should refine technical considerations and provide increased integration of findings into clinical decision making.
Stressed Arterial Phase Imaging
The rationale behind myocardial CT assessment under stress is equivalent to that in other modalities: to differentiate fixed from reversible myocardial perfusion defects, to guide management decisions. Technique and interpretation are identical to those of arterial phase imaging. Stress is most commonly achieved with 140 mcg/minute/kg of intravenous (IV) adenosine over 6 minutes, with rest imaging acquired 15 minutes following adenosine administration. A dose of up to 15 mg of IV metoprolol is usually given if the patient’s heart rate does not decrease to 60 to 65 beats/minute or lower. The rest images are used for coronary CT angiography analysis and usually use collimator settings with the minimum detector width, whereas stress phase acquisitions require less fine anatomic detail and may be acquired with collimation using wider detector element configurations, such as 2 × 32 × 1.5 mm, which improves contrast-to-noise ratio values at the cost of lower spatial resolution.
Fixed defects are defined as those present on both rest and stress images, whereas reversible defects are present only under stress ( Fig. 30-4 ). Combined assessments with coronary CT angiography and rest-stress CT myocardial perfusion imaging have shown good diagnostic accuracy in comparison with other combined assessments, namely, invasive angiography and nuclear medicine myocardial perfusion imaging. Prognostic and clinical value, however, has not been evaluated. Whether this technique is equivalent to dual energy CT (DECT), myocardial perfusion imaging, or time-resolved dynamic perfusion imaging (see later) is also unclear. As with all experimental techniques, institutional experience and additional radiation exposure should be strongly considered during patient selection.
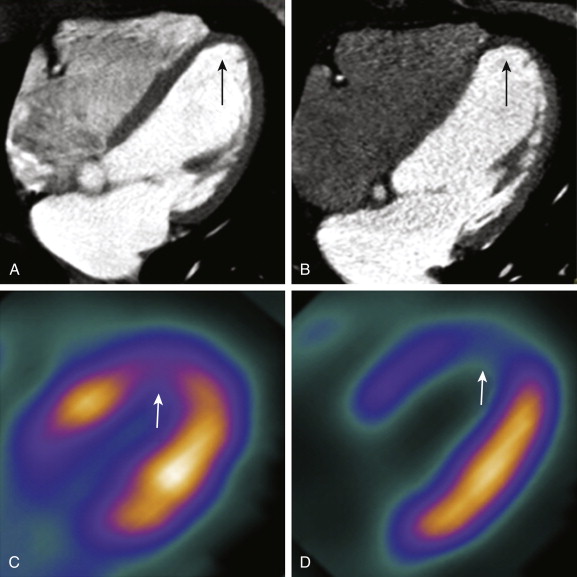
Left Ventricular Function Analysis
The prognostic value of global left ventricular functional status is well established in patients with known ischemic myocardial disease, especially following myocardial infarction, in which global cardiac function is considered the strongest determinant of left ventricular pump failure and death. In addition, regional myocardial motion can be a valuable addition to coronary artery anatomy in routine coronary CT angiography examinations by providing clues to the hemodynamic significance of visualized stenoses. Information on global and regional function of the myocardium is available from all retrospectively ECG-gated examinations without any additional radiation or contrast dose. More recent prospective ECG-triggering techniques have been important in limiting ionizing radiation to the patient, but information across the entirety of the cardiac cycle is lost, thus disallowing left ventricular functional analysis. ECG gating with dose modulation, another trend in radiation dose reduction, may provide a compromise because it has been shown to provide adequate image quality to retain analytic capabilities while still decreasing radiation exposure by 30% to 50%.
The decision whether to perform left ventricular function analysis must take into consideration patient factors, institutional experience, and the clinical scenario. No guidelines currently exist to guide protocol decisions, but a reasonable approach is to place more weight on the value of left ventricular function analysis in patients with a higher likelihood of disease, in those in whom radiation is less of a concern, and in institutions at which this analysis is integrated into routine cardiac CT protocol. In addition, CT may have some role as a primary test for cardiac functional evaluations in those patients with suboptimal examinations or contraindications to other, preferred modalities, such as echocardiography or MRI.
Few variations are required in the acquisition of cardiac CT data sets capable of functional analysis. The only prerequisite is a data set acquired across the entire cardiac cycle. However, several considerations optimize the value of subsequent interpretation. The most important technical parameter is temporal resolution, which must be approximately 100 msec or lower to provide adequate image quality for most heart rates. Some investigators argue that resolution of 30 to 50 msec is optimal, whereas temporal resolution as low as 19 msec may be necessary for true motion-free imaging. Temporal resolution has been improved by performing multisegment reformations (which can provide resolution down to 50 msec) and with the development of dual source machines with increasingly fast gantry rotation speeds. These dual source machines can provide resolution to 75 msec with monosegment reconstructions that, in comparison with multisegment reformations, allow an increased pitch (lowering radiation burden) and provide greater spatial resolution (allowing better delineation of epicardial and endocardial borders) while suffering less image quality degradation from heart rate variability. Dual segment reconstructions with these newer scanners may provide even greater gains in temporal resolution in the future.
Patient-specific factors also weigh heavily in the final quality of functional examinations. High and irregular heart rates affect quantitative, time-resolved imaging disproportionately. Yet the administration of beta-blockers, routine in some institutions, alters hemodynamics and can confound results. In most cases, functional analysis should be considered a secondary goal of cardiac CT examinations, and beta-blockers should be administered as required, with the knowledge that functional parameters will be affected (see later). In addition, proper breath-hold technique is vital, and patients should be instructed accordingly.
Adequate image quality for functional assessment with dose modulation techniques has been shown with tube current reductions up to 80%, typically in the systolic portion of the cardiac cycle. However, the ability to perform functional analysis with reductions greater than this is not established. Otherwise, standard scan parameters may be used. Although routine contrast material delivery protocols have also shown adequacy, some evidence indicates that multiphase delivery may improve the accuracy of some quantitative measures, especially myocardial mass, by providing better delineation of the left ventricular septum from the right ventricular cavity.
Images are reconstructed across the cardiac cycle in a double oblique short-axis plane, from the level of the mitral annulus to the left ventricular apex. Although 20-phase reconstructions, at 5% intervals across the R-R cycle, have been proposed as possibly more accurate, especially in regional cine analyses, several studies showed that 10-phase reconstructions, at 10% intervals, provide equivalent results with advantages in processing time and system requirements.
Two main methods are used for global function analysis. Semiquantitative techniques using the Simpson method have been available for some time. This method uses two-dimensional planimetric measurements from contiguous 8-mm short-axis reformations along the length of the ventricular long axis that are summed to provide left ventricular volume. Software using this method is subject to problems in the reconstruction of the short-axis plane, delineation of endocardial and epicardial contours, and determination of the most basal and apical left ventricular segments; these errors often necessitate extensive and time-consuming manual intervention. This technique also fails to account for myocardial trabeculations and papillary muscles when blood volumes are quantified. Newer software systems use a three-dimensional segmentation technique based on attenuation differences between the contrast-filled left ventricular cavity and the myocardium (the region-growing approach). This method may provide several advantages over semiquantitative techniques, not the least of which is decreased postprocessing time, from 5 to 10 minutes to less than 3 minutes. In addition, trabeculations and papillary muscles are excluded from left ventricular volumes, and this may provide more accurate values. Software using this method is variable in the required amount of manual intervention; some systems provide automatic detection of basal and apical boundaries, whereas others do not. These techniques may also require adjustment of the attenuation threshold or endocardial contours to provide optimal results. However, even with these requirements, the three-dimensional region-growing approach is significantly faster than are semiquantitative techniques. Either method is used to quantify left ventricular myocardial mass, end-systolic and end-diastolic volumes, stroke volume, ejection fraction, and cardiac output.
Regional myocardial function is most commonly evaluated subjectively using multiphase cine reconstructions with 0.5- to 2-mm reconstruction width and increment. Left ventricular myocardial motion should be segmentally evaluated in accordance with the American Heart Association 17-segment model of the myocardium, excluding the apex. Regional wall motion is characterized, as with other modalities, as normal, akinetic, hypokinetic, or dyskinetic. Correlation with findings from coronary CT angiography analysis is vital to improving the predictive value of the cardiac CT examination as a whole.
The diagnostic value of CT left ventricular functional analysis has been well validated, with certain caveats. Both global assessment methods have excellent interobserver agreement and reproducibility, although the region-growing approach has slightly better values. The tendency has been for CT to overestimate end-systolic volume and subsequently underestimate ejection fraction in comparison with MRI. However, this tendency has been attributed in part to the use of beta-blockers and the inclusion of trabeculae and papillary muscles when chamber volumes are calculated. More recent studies using three-dimensional segmentation decreased this trend, and phantom studies suggested that CT may even be more accurate than MRI under ideal circumstances (i.e., slow and regular heart rates) because of its superior spatial resolution. Subjective regional wall motion analysis has also shown good correlation with echocardiography and MRI; in addition, more recent attempts to quantify regional motion with CT-based myocardial strain mapping have shown promise.
Future work should help guide optimal scan acquisition and postprocessing. The promise of greater radiation dose reductions with advanced acquisition and reconstruction methods also exists. The prognostic and clinical value of CT functional analysis has not been studied and will require validation. Furthermore, normalized sex- and gender-specific morphometric data should be established to aid interpretation of quantitative parameters.
Delayed Phase Imaging
The current gold standard for detecting myocardial viability is delayed phase MRI for the detection of late contrast enhancement. Late enhancement patterns are well established tools that provide clinically valuable information in the setting of acute and chronic ischemia. The same principles provide the rationale for delayed phase CT myocardial imaging. Both gadolinium-based and iodine-based contrast agents remain in the extracellular space. Acute ischemia causes membranous disruption in affected myocytes, which are normally tightly packed. This structural insult, along with accompanying edema, increases the volume of contrast agent distribution in infarcted areas and causes a relative increase in concentration and delayed washout compared with normal myocardium ( Fig. 30-5 ).
