Abstract
The differentiation between infarcted, non-viable myocardium and myocardium that is still viable has been an area of extensive research and debate over several decades. Despite this, controversy still exists as to the definitions of viable myocardium, the best non-invasive imaging method to use for viability assessment and whether viability is clinically important or not. This chapter will define viability in its different forms, compare and contrast the non-invasive imaging modalities most commonly used, and discuss the clinical context where knowledge of viability may inform clinical decisions at a patient level. Areas of ongoing research and emerging new imaging methods will be highlighted and discussed.
Keywords
Myocardial viability, Stunning, Hibernation, Myocardial infarction, Revascularisation
Acknowledgements
The authors would like to thank The Royal Brompton Hospital CMR Unit, Dr Stephan Nekolla, Dr Filip Zemrak, and Dr Navtej Chahal for assistance with images.
11.1
Background, terms, and definitions
11.1.1
Introduction
With advancing technology, non-invasive imaging has gone through exponential growth and myocardial viability assessment is now possible using several different imaging modalities with numerous different techniques. Echocardiography, computed tomography (CT), single-photon emission computed tomography (SPECT), cardiovascular magnetic resonance (CMR), and even positron emission tomography (PET) imaging have become much more widely accessible, meaning patients may now undergo a number of different imaging studies prior to decisions on invasive treatments and particularly revascularisation therapy. The differentiation between infarcted, non-viable myocardium and regions that are still viable has been an area of extensive research and debate over several decades; however, the clinical role of viability information is still controversial. This chapter will define viability in its different forms, compare and contrast the non-invasive imaging modalities most commonly used, and discuss the clinical context where knowledge of viability may inform clinical decisions at a patient level. Areas of ongoing research and emerging new imaging methods will be highlighted and discussed. We are able to gather huge quantities of information from non-invasive imaging studies of viability, and by doing this we hope to limit the risks of invasive procedures and treatments to those who are likely to gain benefit and reduce the number of unnecessary or unsuccessful interventions. Increasingly, cardiac surgeons and physicians are required to be able to assimilate information from several different forms of viability studies and it is important to understand the strengths and weaknesses of the different modalities ( Table 11.1 ), as well as the usefulness of this data at a patient level.
Imaging technique | Main physical property imaged | Advantages | Disadvantages | LV segmental recovery | Global LV functional improvement | ||
---|---|---|---|---|---|---|---|
Sensitivity | Specificity | Sensitivity | Specificity | ||||
LDDSE | Contractile reserve | Relative low cost, availability, few contraindications | Image quality variable, operator dependent | 79% | 78% | 57% | 73% |
SPECT | |||||||
Thallium-201 | Myocyte membrane integrity | Availability, many years experience | Limited resolution, radiation dose | 87% | 54% | 84% | 53% |
Technetium-99 sestamibi or Tetrofosmin | 83% | 65% | 84% | 68% | |||
PET | |||||||
FDG | Glucose metabolism | High accuracy | Radiation dose, cost, limited availability, patient preparation | 92% | 63% | 83% | 64% |
CMR | |||||||
LGE | Scar/interstitial space | High accuracy, reproducible, lack of radiation | Limited availability, gadolinium relatively contraindicated in severe renal dysfunction, contraindicated with metallic implants | 84% | 63% | – | |
LDD | Contractile reserve | Good image quality, no contraindication in renal dysfunction | Relatively long procedure, claustrophobia | 81% | 91% | – |
11.1.2
Ischaemic heart disease and heart failure
Deaths from coronary artery disease (CAD) have been falling in the Western world over the past few decades due to progressive advances in primary and secondary prevention as well as improvements in acute treatments of myocardial infarction (MI). However, CAD remains the cause of death in 1 in 6 Americans [ ] and 1 in 5 Europeans [ ], and although survival is improving, approximately 20% of over 65-year-olds will subsequently develop heart failure within 5 years of a first MI [ ]. Approximately two-thirds of cases of systolic heart failure are caused by CAD [ ], and the overall mortality for people diagnosed with heart failure remains 50% at 5 years [ ]. Therefore, it is clear that there is still significant room for improvement in the treatment of this group of patients. The ability to predict who will benefit from revascularisation therapies is an attractive prospect that may help target interventions appropriately. An assessment of myocardial viability has become integral to this process, although the ideal method of assessment remains controversial.
11.1.3
Viable myocardium
Viable myocardium is commonly used as an umbrella term that may describe many different myocardial entities, including normal, myopathic, ischaemic, or partially infarcted. However, the term “viable myocardium” is particularly important when that area is dysfunctional, and implies capacity for improvement if the insult (usually ischaemia) is corrected and differentiates from areas that are non-viable and will not recover function. The identification of viable and non-viable myocardium should allow the direction of therapies to provide most benefit for the risk of the intervention. Of course, how to decide whether myocardium is viable, and what intervention may be of benefit, are not always straightforward questions to answer and will be discussed in this chapter.
11.1.4
Myocardial cell death
Ischaemia is the most common cause of myocyte death. Myocyte dysfunction occurs very early following the onset of ischaemia and irreversible cell death occurs in a matter of minutes. During an MI the progression of injury is described as a “wave front” proceeding from endocardium to epicardium [ ] (see Chapter 10 ). In theory, in the absence of reperfusion, all myocardium subtended by the occluded artery will become infarcted – a transmural MI. However, in chronic coronary arterial disease, the development of extensive collateral vessels may allow a large vessel to occlude with minimal resultant infarction. Increasingly, reperfusion therapy is administered, aborting the MI, leaving an infarct which has progressed only partway between endo- and epicardium. It is most commonly in these cases that an assessment of viability is required but is also most challenging. Whether or not to offer revascularisation to a dysfunctional and partially infarcted myocardial territory is the key question that non-invasive imaging attempts to answer.
11.1.5
Myocardial stunning
As discussed above, myocardial ischaemia does not always result in cell death. “Stunning” of the myocardium represents myocardial dysfunction which persists following a period of non-lethal myocardial ischaemia, despite restoration of coronary blood flow. Therefore there is a “mismatch” between the normal perfusion and abnormal contraction. Stunning may also occur following exercise-induced ischaemia in the setting of critical coronary stenosis or following cardiac surgery and cardioplegic cardiac arrest [ , ]. Importantly, this form of myocardial dysfunction will resolve over time. The exact mechanism remains to be elucidated but it is thought that oxygen-derived free-radical formation, transient intracellular calcium overload, and excitation–contraction uncoupling result in contractile dysfunction which recovers gradually [ , ].
11.1.6
Myocardial hibernation
This situation arises in the face of chronic ischaemia or repeated episodes of stunning where the myocardium adapts to enter a low energy usage state as a protective mechanism from infarction [ ] but which results in contractile dysfunction (see Figure 11.1 for some of the postulated pathophysiological mechanisms of myocardial stunning and hibernation). In contrast to early definitions, resting flow in hibernating myocardial segments may not be decreased to the extent that would account for the degree of contractile dysfunction. In most cases, there is impairment of myocardial perfusion reserve (MPR), with reduction of resting perfusion seen in only the most severe cases ( Figure 11.2 ). Hibernation describes a spectrum with chronic repetitive stunning showing normal or near normal resting perfusion and impaired MPR at one end and reduced resting perfusion at the other. In order to recover function in hibernating myocardium, coronary revascularisation is required which restores an adequate MPR.
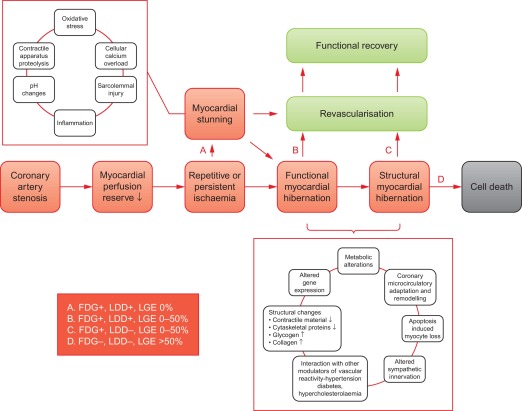
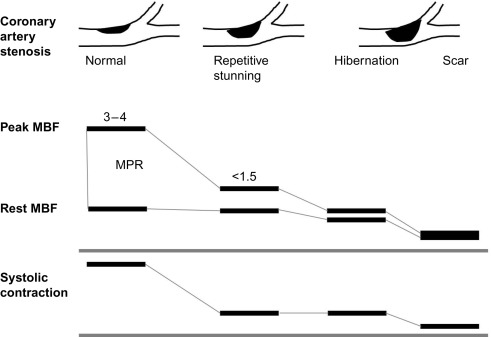
11.1.7
Clinical setting
In the clinical arena, away from the controlled conditions of the laboratory, stunning and hibernation may be encountered together or separately in a number of conditions. For instance, even if an acute MI is treated rapidly with primary percutaneous coronary intervention or thrombolysis, significant LV dysfunction may result. This will be composed of an established infarct which will not be salvageable and probably a component of myocardial stunning in surrounding tissue which will recover over hours to days. In the more chronic presentation of heart failure due to ischaemic heart disease, there is likely to be a contribution from established infarction and, due to ongoing persistent ischaemia, other areas will have entered a hibernating state. Other conditions may include congenital defects leading to ischaemic myocardium, such as anomalous left coronary artery from the pulmonary artery or occasionally severe valvular disease. On a case-by-case basis, it is the diagnosis of the underlying pathology and then the clarification of the relative contributions of viable (stunned or hibernating) and non-viable myocardium by multimodality imaging which may guide future therapy and evaluate associated risks and benefits.
11.2
Multimodality imaging for myocardial viability assessment
Since the discovery that a MI did not have to result in complete tissue death [ ], the concept of viable and non-viable areas of myocardium has become increasingly important. The search for reliable markers of viability predates this, however, as the clinical question of viability is relevant not only in the acute phase of a MI but also with chronic ischaemia. Early studies in cardiac surgery demonstrated improvement in LV function following revascularisation even in some akinetic territories thought to be completely infarcted [ ]. Early assessments of viability were largely based on ECG criteria – estimating infarct size with measurement of ST segment elevation height [ ] or chronically with the presence of Q waves. The use of invasive left ventriculography was also common but it was recognised that dysfunction did not always mean non-viable and detailed conclusions from this technique were limited. However, the presence of contractile reserve had been identified with an epinephrine challenge, the so-called epinephrine ventriculogram [ ]. Cardiac enzyme quantification (mainly creatine kinase release) was also used [ ]. Whilst this correlates to infarct size, and may be a useful surrogate end-point in clinical trials, it does not allow an assessment of residual viable tissue in an individual. Thereafter, the ideal non-invasive imaging technique to measure infarct size and to establish whether residual myocardium is viable or not has been pursued with vigour [ ].
11.2.1
Morphology
All imaging techniques allow some descriptive assessment of the left ventricle and some morphological features may give early clues to viability. It has been recognised for some time that myocardial cell death leads to thinning of the ventricle wall [ , ] and reduced residual viable myocardium. On this basis, severe regional thinning with contractile dysfunction maybe detected by several different imaging modalities and represents an indicator of viability. Echocardiography is often the first line investigation used (it is widely available, portable and does not require radiation) and will give the first information regarding viability. Nuclear techniques tend not to be used for morphology alone due to limited spatial resolution, whereas CMR excels in this area with excellent spatial resolution and unlimited image planes, allowing straightforward and accurate wall thickness measurement ( Figure 11.3 ). In patients where poor acoustic windows make echocardiography impossible or where an implantable cardiac device renders CMR unfeasible, cardiac CT is an alternative option. Using retrospective gating to image throughout the cardiac cycle, or prospective gating with padded acquisition windows to include end-systole and end-diastole, it is possible to acquire whole-heart data very rapidly with CT. In post-processing, regions of interest can be drawn in a similar fashion to CMR around the LV/RV endo- and epicardium at end-systole and end-diastole in order to calculate end-diastolic volume, end-systolic volume, and myocardial volume which can be converted to mass. CT measures compare favourably to that of CMR, with r = 0.95–0.97 for LV measures and r = 0.94–0.97 for RV volumes, using a 16 slice CT scanner [ ], and r = 0.89–0.96 for LV volumes and mass measures and r = 0.7–0.88 for RV volumes and mass using a dual-source CT scanner with low radiation (20%) during the majority of the acquisition time [ ]. Technically, in order to achieve good definition of the interventricular septum and RV cavity, it is important not to wash out the contrast from the RV and so a mixed saline/contrast “chaser” can be used, following the initial contrast bolus in order to avoid this.
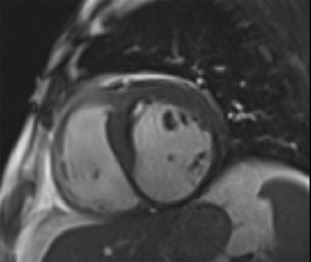
With echocardiography, chronic MI will eventually produce a thinned and “echo bright” appearance of the myocardium. In a group of 45 patients, a LV segmental wall thickness of < 6 mm gave a sensitivity of 94%, specificity of 48%, and negative predictive value of 93% for non-recovery of function following revascularisation, although the reverse was not true, as a value > 6 mm was not predictive of viability [ ]. A recent CMR study has cast some doubt on this relatively straightforward parameter. Of 201 patients studied, 18% with a thinned myocardial wall (end-diastolic wall thickness < 5.5 mm) were found to have limited scar burden in this area using late gadolinium imaging. There was poor correlation between wall thickness and scar extent ( r = − 0.22) and the likelihood of recovery following revascularisation was reliably predicted only by the scar extent and not by regional thinning [ ]. In addition, the presence of Q waves or collateral flow on angiography did not reliably predict the extent of scar tissue. Therefore, myocardial thickness may be an early indicator of viability in some patients but additional information is required in order to make clinical decisions.
11.2.2
Contractile reserve
Whilst a regional wall motion abnormality may provide the first indicator of previous myocardial injury, it is not always possible to reliably distinguish between stunned, hibernating, or completely infarcted myocardium under rest conditions. However, the response of severely hypokinetic or akinetic segments to stress may allow an assessment of viability by demonstrating whether the area has contractile reserve. Although low-level exercise stress can be used, dobutamine pharmacological stress is the most commonly used method and may be used alongside echocardiography or CMR. To avoid increasing myocardial oxygen demand and provoking more profound hypokinesis, a low dose (typically 5–10 mcg/kg/min) is used, aiming to provide inotropic effects without significant chronotropic response. If additional information regarding ischaemia is required, then doses up to 40 mcg/kg/min with additional atropine may be used, in order to reach a target heart rate of 85% of the predicted maximum for age. Echo is the more traditional modality and is particularly reliant on good acoustic windows, even more so than resting echo, as subtle changes in wall motion may be crucial. If two contiguous LV segments are incompletely visualised then the use of blood pool contrast agents is recommended [ ], which can significantly improve the endocardial border visualisation and may allow a combined perfusion assessment (see Chapter 2 ). The “classic” response of dysfunctional but viable myocardium to dobutamine stress is biphasic, with an initial increase in contractility at low dose but with a worsening of contractility at higher doses due to developing ischaemia ( Figure 11.4 ). This biphasic response is most associated with segmental functional recovery following revascularisation [ ]; however, other responses may be seen ( Figure 11.5 ).
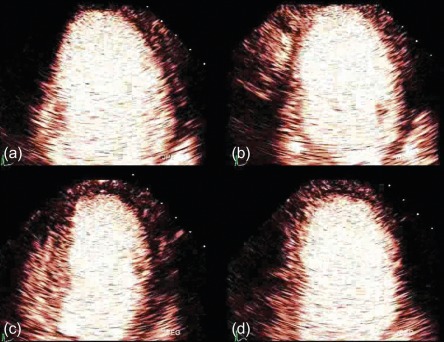
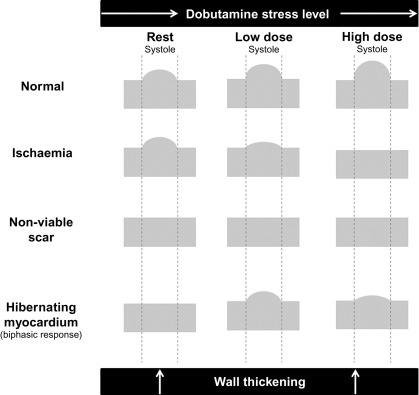
Continued functional improvement through low and high doses of dobutamine suggests a limited infarct which has produced a resting regional wall motion abnormality but is not ischaemic. No response to dobutamine may mean that the segment is non-viable and will not recover function once revascularised; however, caution should be exercised in cases of critical ischaemia, where even low doses of dobutamine may cause a further reduction in myocardial blood flow, preventing any apparent initial improvement of contraction in viable tissue. These segments often have mild reduction in resting perfusion with avid uptake of FDG or SPECT tracers and almost completely exhausted MPR which prevents the segments from increasing their oxygen consumption upon inotropic stimulation and hence from increasing their contractile function. Apart from the exhaustion of MPR, other factors, such as the presence and severity of cardiomyocytic alterations or down-regulation of beta-adrenoceptors, may also contribute to the lack of contractile reserve in apparently viable segments.
Dobutamine stress techniques evaluating contractile reserve in general have slightly lower sensitivities than perfusion modalities but with higher specificity. Pooled analysis of 1121 patients using low-dose dobutamine stress echo (LDDSE) to predict regional improvement in function following revascularisation gave a weighted mean sensitivity of 79% and specificity of 78% which improves to 83% and 79% respectively when high-dose dobutamine is used [ ]. Tissue Doppler (see Chapter 2 ) has been used with some success to try and give objective measures to viability assessment [ ]. Although increasing the sensitivity in this study, angle dependency and an increased examination time have prevented this being adopted routinely.
Low-dose dobutamine used as part of a CMR study to evaluate viability allows the attractive prospect of being able to evaluate infarct extent (see infarct imaging below), visualise un-infarcted areas and test for contractile reserve in one sitting. Technically this is performed in a similar fashion to DSE with an incremental increase in dobutamine dose from typically 5–10 mcg/kg/min. At rest, and with each dose increment, three short axis and three long axis cine images are acquired and assessed for regional wall motion. For ischaemia assessment, it is possible to administer gadolinium-based contrast agents at peak stress (40 mcg/kg/min) to assess for inducible perfusion defects with first pass perfusion. This may help improve the specificity [ ] for diagnosis of coronary disease, but whether this adds additional weight to a viability decision is unknown.
A meta-analysis of nine small studies using LDD CMR calculated the mean weighted sensitivity and specificity as 81% (95% CI: 73–86%) and 91% (95% CI: 84–95%), whereas the PPV and NPV were 93% (95% CI: 87–97%) and 75% (95% CI: 65–83%), respectively. The weighted overall accuracy for this technique in isolation was 84% (95% CI: 82–86%) [ ].
Finally, low-dose dobutamine has been combined with nitrate enhanced, sestamibi, and ECG-gated SPECT in order to assess LV regional wall motion, and has good agreement with LDDSE [ ]. Inability to assess segments with low tracer uptake limits use in some patients, but in general, wall motion and LVEF can also be accurately assessed during a viability/perfusion study by SPECT or PET [ ].
11.2.3
Membrane integrity
Myocardial perfusion scintigraphy (MPS) relies on the fact that the tracer is taken up by intact cardiac myocytes but not myocardial scar. SPECT MPS is a well-established and widely available technique. 201-Thallium is not only a perfusion agent but also a tracer of myocardial viability due to the reliance of tracer uptake on both myocardial blood flow and sarcolemmal integrity (see Chapter 3 ). It has therefore been used widely for identifying myocardial viability and hibernation. Various imaging criteria have been proposed for the identification of viable myocardium. The most commonly used is the ratio of radiotracer uptake in the relevant region to the uptake seen in the best-perfused area of the myocardium. There is a direct relationship between tracer uptake and amount of viable myocardium and an inverse relationship with the amount of connective tissue. Myocardial viability is considered clinically significant when the detected tracer uptake exceeds 50% of the maximum in the myocardium. This criterion is highly specific, if there is coexisting ischemia at rest. As thallium redistribution may be slow or incomplete in regions of reduced perfusion, the usual stress/redistribution protocol can underestimate myocardial viability and additional steps may be required. These include late redistribution imaging at 8–72 h after stress injection, reinjection of tracer at rest after redistribution imaging, and a resting injection on a separate day with both early (20 min) and delayed imaging (4 h) ( Figure 11.6 ).
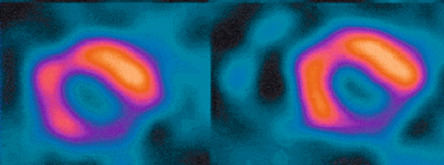
MIBI and tetrofosmin have also been used for the detection of viable and hibernating myocardium. In theory, these tracers may underestimate viability in areas with reduced resting perfusion because they do not redistribute and cannot thus allow independent distinction of perfusion and viability. Some studies have therefore found 201 Tl SPECT MPS to be a more sensitive test for the assessment of viability ( Figure 11.7 ). However, the use of sublingual nitrates improves resting perfusion and thus the detection of myocardial viability using technetium tracers. An important advantage of using MIBI or tetrofosmin SPECT is the ability to perform ECG gating and thus assess ventricular function. Whichever tracer is used, SPECT assessments have generally higher sensitivity but poorer specificity when compared with DSE for the detection of myocardial viability. Pooled analysis of 40 studies (1119 patients) using thallium-201 to predict the segmental recovery of function calculated the weighted mean sensitivity and specificity to be 87% and 54% respectively, with positive and negative predictive values (PPV or NPV) of 67% and 79% respectively [ ]. Similar analysis of 25 studies (721 patients) using technetium-99 sestamibi or tetrofosmin found a sensitivity and specificity of 83% and 65% respectively, with PPV and NPV of 74% and 76%, respectively [ ]. The generally lower specificity for regional recovery of function may not be such a critical disadvantage because other factors, such as the improvement of symptoms and prognosis after revascularisation, are more important than the exact prediction of improvement of regional wall systolic function. The higher sensitivity of SPECT is likely to be due to the reliance of tracer uptake on myocyte membrane integrity, which may be preserved even after loss of myocyte contractile reserve. The use of a 50% threshold of maximal myocardial uptake to identify viability may contribute to the poorer specificity and SPECT cannot assess transmurality of an infarct. It may also encounter difficulties when there is severe global myocardial ischaemia, as the assessment of relative perfusion relies on at least one segment with normal blood flow. This situation is uncommon but obviously important not to miss, and so other signs, such as transient LV dilatation during stress, must be considered.
