Table 6-1 Inherited Cancer Predisposition Syndromes in Which Exposure to Ionizing Radiation Should Be Minimized | ||||||||||
---|---|---|---|---|---|---|---|---|---|---|
|

It acts as a tumor suppressor gene that functions in part as a negative regulator of the RAS protooncogene (12) and of the mammalian target of rapamycin (mTOR) pathway (13).
It acts as a regulator of neural stem cell proliferation, survival, and astroglial differentiation, in addition to regulating neuroglial progenitor function (14,15). Neurofibromin is required for regulation of intracellular cyclic AMP (cAMP) generation in both neurons and astrocytes; abnormal levels of cAMP appear to be, at least in part, responsible for the abnormalities in glial and neuronal development evident in NF1 patients.
Table 6-2 The Rasopathies
NF1
Noonan syndrome (NS)
Costello syndrome
Capillary malformation-arteriovenous malformation syndrome
Cardiofaciocutaneous syndrome
Legius syndrome
NS with multiple lentigines (LEOPARD syndrome)
It regulates ERK signaling in GABA release, an important pathway involved in learning (and therefore in learning disabilities) (16).
It is involved in the maintenance of the vascular wall. Neurofibromin is expressed in the vascular endothelium and in vascular smooth muscle cells; loss of neurofibromin likely causes smooth muscle proliferation, possibly in response to nonspecific injury to the vascular wall (15), which can lead to vasculopathy (17).
It is involved in bone formation and remodeling. Neurofibromin is expressed in osteoblasts; it inhibits collagen synthesis, promotes mineralization, and regulates osteoclastogenesis (18).
It seems to be required for normal myelination by Schwann cells (18). In addition, the gene for oligodendrocyte myelin glycoprotein, a major myelin protein, is embedded within intron 27b of the NF1 gene (19). Therefore, it is not surprising that abnormalities of myelin/white matter are seen in patients with NF1.
enlargement is primarily attributable to an increase in cerebral white matter volume, with a smaller contribution from the gray matter (30). About 4% to 7% of patients have epilepsy, primarily related to intracranial masses and cytoarchitectural abnormalities (31).
Table 6-3 Criteria for Diagnosis of Type 1 Neurofibromatosis | ||
---|---|---|
|
Table 6-4 Incidence of Clinical Features of NF1 | ||||||||||||||||||||||||||||||||||||||||||||||||||||||||||||||||||||||||||||||||||||||||||||||||||||||||||||||||||||||||||||||||||||||||||||||||||||||||||||||||||||
---|---|---|---|---|---|---|---|---|---|---|---|---|---|---|---|---|---|---|---|---|---|---|---|---|---|---|---|---|---|---|---|---|---|---|---|---|---|---|---|---|---|---|---|---|---|---|---|---|---|---|---|---|---|---|---|---|---|---|---|---|---|---|---|---|---|---|---|---|---|---|---|---|---|---|---|---|---|---|---|---|---|---|---|---|---|---|---|---|---|---|---|---|---|---|---|---|---|---|---|---|---|---|---|---|---|---|---|---|---|---|---|---|---|---|---|---|---|---|---|---|---|---|---|---|---|---|---|---|---|---|---|---|---|---|---|---|---|---|---|---|---|---|---|---|---|---|---|---|---|---|---|---|---|---|---|---|---|---|---|---|---|---|---|---|
|
time to progression. Sirolimus (an inhibitor of the mammalian target of rapamycin (mTOR) pathway) (51), imatinib mesylate (Gleevec, a cMET blocking agent) (52), and MEK inhibitors (53) have shown demonstrable activity in their treatment.
significant subarachnoid tumor (Fig. 6-2C). The perineural form is tumor infiltration of the subarachnoid space (astrocytic proliferation), creating a rim of tumor around a relatively unaffected nerve; at times, both forms can coexist (Fig. 6-3B) (66,67). Postcontrast MR using fat suppression will distinguish the two architectural forms of optic nerve gliomas. When the nerve is diffusely infiltrated, enhancing tumor fills the optic nerve sheath, whereas infiltration of the subarachnoid spaces shows a rim of enhancing tumor around a minimally enhancing optic nerve. Marginal cases (characterized by development of mild thickening of the optic nerves or by transient or mild enhancement of the optic nerves) are commonly observed; it is not clear whether these truly warrant a diagnosis of OPG (60). Finally, optic nerve tortuosity (without abnormal thickening or enhancement) is commonly observed in NF1 and should not be mistaken for an optic nerve glioma (60,68).
septum pellucidum; laterally into the temporal lobes; posteriorly into the optic radiations; and inferiorly into the cerebral peduncles and the brainstem (Fig. 6-2). Rarely, tumor can extend into the lateral ventricles.
vacuolation (Fig. 6-9). This hamartomatous enlargement should not be misdiagnosed as a brainstem glioma; differentiating features from infiltrating gliomas include normal or nearly normal signal in T1-weighted images, only modest (and usually heterogeneous) signal increase on T2 images, absence of contrast enhancement, and lack of progression on follow-up scans. The T2 signal abnormalities partly regress during adolescence, though the brainstem remains enlarged. For more discussion of brainstem tumors, see Chapter 7.
Occasionally, the tectum may appear short and thick in aqueductal stenosis because of mass effect on the rostral aspect of the tectum by a dilated suprapineal recess (see Chapter 8). In these cases, the patient should be reevaluated after ventricular decompression.
![]() Figure 6-11 Sagittal T1-weighted image shows an abnormally thick corpus callosum (white arrows). The ventral pons (black arrows) is prominent. |
repair or remyelination (98). The increased diffusivity of white matter (even normal-appearing white matter) in NF1 (99,100) further supports the concept of abnormal myelin as an underlying cause.
abnormality or be located along its periphery/rim. (Calculation of T1 values shows that the frontal white matter, caudate nuclei, putamina, and thalami also have some T1 shortening (86), but it is not apparent to visual inspection in these other areas.) In some patients, the T1 shortening is observed at the time of a first MRI, prior to exposure to gadolinium-based contract agents. However, many of the NF patients with appreciable T1 bright signal changes in the globus pallidus also have bright T1 signal in their dentate nuclei and have undergone multiple contrast-enhanced MRI exams; the T1 bright signal changes in some of these cases, therefore, may be a reflection of gadolinium deposition in the brain (107), rather than a reflection of an intrinsic abnormality of the myelin.
artery, or proximal anterior cerebral artery is the most common site of involvement (Figs. 6-16 and 6-17). Moyamoya phenomenon with marked enlargement of the lenticulostriate arteries (which function as collateral vessels) is seen in many such patients (113). (Moyamoya is discussed further in Chapter 12.) Fusiform arterial dilation, cerebral aneurysms, and arteriovenous fistulas are also described, although less commonly (109,113,114).
often reveals extension into the cavernous sinus, nasopharynx, or pterygomaxillary fissure. The neck is another common location for neurofibromas, especially along the course of the vagal nerve, with an estimated occurrence of 25% to 30% in patients with NF1 (Fig. 6-20) (120). Neck masses, their appearance, and their differential diagnoses are discussed in Chapter 7.
curves are associated with a high incidence of paraspinal or other internal neurofibroma next to the vertebra. Patients with paraspinal PNs have sixfold higher odds of developing spinal curvature abnormalities compared to patients without PNs (128). Dysplasia of the vertebral bodies is common, as are hypoplasia of the pedicles, transverse processes, and spinous processes; scalloping of the posterior aspects of the vertebral bodies; and hyperplastic bone changes (120,129). It is not certain whether these bony anomalies result from a primary mesodermal dysplasia or are secondary to the effects of nerve sheath tumors. Plain film radiography is essential to demonstrate the scoliosis optimally. CT is the optimal modality for demonstrating the changes of the individual vertebrae, as it shows superior bone detail. However, most of the bony changes are well visualized with high-quality MR studies.
a threshold value of 5 cm is frequently used (140,141). Other MR findings indicative of a MPNST (Fig. 6-21) include irregular tumor shape, poor demarcation from surrounding tissues, evidence of necrosis, intratumoral lobulation, presence of high-signal-intensity foci on T1-weighted images (corresponding to intratumoral hemorrhage) and lack of target sign. ADC values tend to be lower than for benign peripheral nerve sheath tumors. Heterogeneous enhancement and a lower proportion of enhanced area have been documented (122,125,142). Of note, marked heterogeneity can be seen in benign tumors as well (124), so this is not a specific imaging sign.

begin to develop in children, symptoms are often delayed until early adulthood (late teens or 20s) when the tumors become large enough to cause symptoms. In contrast to the situation in adults, hearing loss is an uncommon presentation in childhood; seizures (caused by meningiomas) and facial nerve palsy are much more common presenting symptoms, as are neurologic symptoms related to brainstem and/or spinal cord tumors (150,153,154). Tumor load tends to be extensive when presentation is during childhood; cranial meningiomas are seen in 60% of cases, cranial schwannomas (other than vestibular) in 36%, and spinal schwannomas and meningiomas each in 80% (154). NF2-associated intracranial tumors show substantial variability in growth rate and pattern. The most common growth pattern is saltatory, characterized by periods of growth and quiescence; linear growth and exponential growth are less common (155).
mildest form of the disease with late onset. Patients have slowly growing eighth cranial nerve schwannomas and not more than one other tumor (meningioma or schwannoma). Type 2, also called Wishart’s form of NF2 or the Wishart-Lee-Abbott form, is a more severe form characterized by early onset and the presence of multiple tumors, including schwannomas, meningiomas, ependymomas, and sometimes astrocytomas. Patients with type 2 NF2 have a higher incidence of cataracts and skin tumors, as well as CNS tumors, than do those with type 1 NF2 (137). These subgroups have proved useful for prognostic purposes; however, the reader should remember that, as with nearly all such groupings, many patients with NF2 have intermediate severity of the disease and may not fall neatly into either form.
Moreover, it may fall upon the radiologist to make the diagnosis of NF2 in affected patients. The characteristic intracranial abnormalities are schwannomas of the vestibular (Figs. 6-27, 6-28, 6-30) and other cranial nerves (most commonly the oculomotor and the trigeminal nerves, Fig. 6-29) and meningiomas (often multiple, Fig. 6-30).
Table 6-5 Criteria for Diagnosis of Neurofibromatosis Type 2 | |||
---|---|---|---|
|
schwannomas can be seen (148). Ependymomas and astrocytomas of the spinal cord are sometimes difficult to distinguish by CT and MR; the presence of a centrally located, contrast-enhancing tumor with sharply marginated borders favors a diagnosis of ependymoma over astrocytoma. Ependymomas can be solitary (most often involving the conus medullaris and filum terminale), or multiple, occurring at all levels of the neural axis. Contrast-enhanced MR is the imaging modality of choice. Without the administration of contrast, ependymomas of the spinal cord may be difficult to differentiate from multiloculated syringohydromyelia. Further characteristics of intramedullary neoplasms are described in Chapter 10.
be caused by fluid secreted into the spinal cord and central canal by the tumor (175). If syringohydromyelia is seen in neurofibromatosis (either NF1 or NF2) and no extramedullary mass can be seen, a thin section, contrast-enhanced MR of the spinal cord should be performed to rule out an intramedullary lesion. Syringohydromyelia is discussed in more detail in Chapter 9.

infants presenting with infantile spasms have tuberous sclerosis (210). The infantile spasms evolve into other seizure types, most commonly symptomatic generalized epilepsy (˜60%), partial epilepsy (˜20%), or a mixture of partial and generalized epilepsy (˜20%) (211). The seizures often decrease in frequency with increasing age (212), particularly when the epilepsy is partial (213). Patients with TSC can have nearly any type of seizure; therefore, the diagnosis of tuberous sclerosis should be considered in any child with epilepsy. The incidence of intellectual disability is about 50% (214); approximately two-thirds will be moderately to severely disabled, and one-third only mildly to moderately affected. Cognitive impairment in TSC is a multifactorial condition; a link exists between cognitive disabilities and lesion load (based on the proportion of brain volume occupied by tubers and on the number of RML (215)), age at seizure onset, and history of infantile spasms (216); however, these factors explain only part of the intelligence quotient variability (186).
Table 6-6 Diagnostic Criteria for Tuberous Sclerosis Complex (Revised 1998) | |||||||
---|---|---|---|---|---|---|---|
|
present at birth and are frequently the cutaneous lesions that lead to a diagnosis of tuberous sclerosis in children with seizures (222). In lightskinned children, the depigmented nevi may be demonstrable only under an ultraviolet light. Café au lait spots are occasionally seen in patients with tuberous sclerosis, but their incidence is similar to that in the general population; their presence in isolation should not suggest a diagnosis of phakomatosis (223). Rarely, patients may develop lesions of the scalp that induce hyperostosis of the underlying calvarium; histologically, these seem to be epidermal inclusion cysts. The other common cutaneous lesions of tuberous sclerosis, shagreen patches and subungual fibromas, do not usually appear until after puberty and will not be discussed here.
CT and MR changes with the age of the patient. They are rarely calcified in the first year of life; the number of calcifications typically increases with the age of the patient (217). Thus, they may be difficult to detect on CT scans of infants (Fig. 6-36C) but become progressively easier to identify as they calcify (Fig. 6-37, also Fig. 6-40B). On MR scans, subependymal hamartomas appear as irregular SENs that protrude into the adjacent ventricle. Their appearance changes as the signal of the surrounding white matter changes (209,230). In fetuses and infants, who have unmyelinated white matter, the hamartomas are relatively hyperintense on T1-weighted images and hypointense on T2-weighted images (Figs. 6-35 and 6-36) (231); in fetuses and newborn (especially premature) infants, these may be mistaken for subependymal hemorrhages unless other lesions of tuberous sclerosis are identified. As the brain myelinates, the SENs gradually become isointense with the white matter. They are most easily visualized on T1-weighted images where they contrast with low signal intensity of the CSF. Small nodules may not be apparent on T2-weighted images. Larger SENs manifest variably low signal intensity on the T2-weighted images, depending upon the extent of calcification (209,232,233). T2*-weighted gradient echo or susceptibility-weighted images are optimal for showing the calcification because of the magnetic susceptibility differences of calcium and brain (see Fig. 6-41). After intravenous administration of paramagnetic contrast, SENs show variable enhancement; some will enhance markedly, some mildly, and some not at all (209,234,235). The presence or absence of enhancement has no clinical significance. SENs have increased diffusivity and reduced FA compared to surrounding white matter (236).
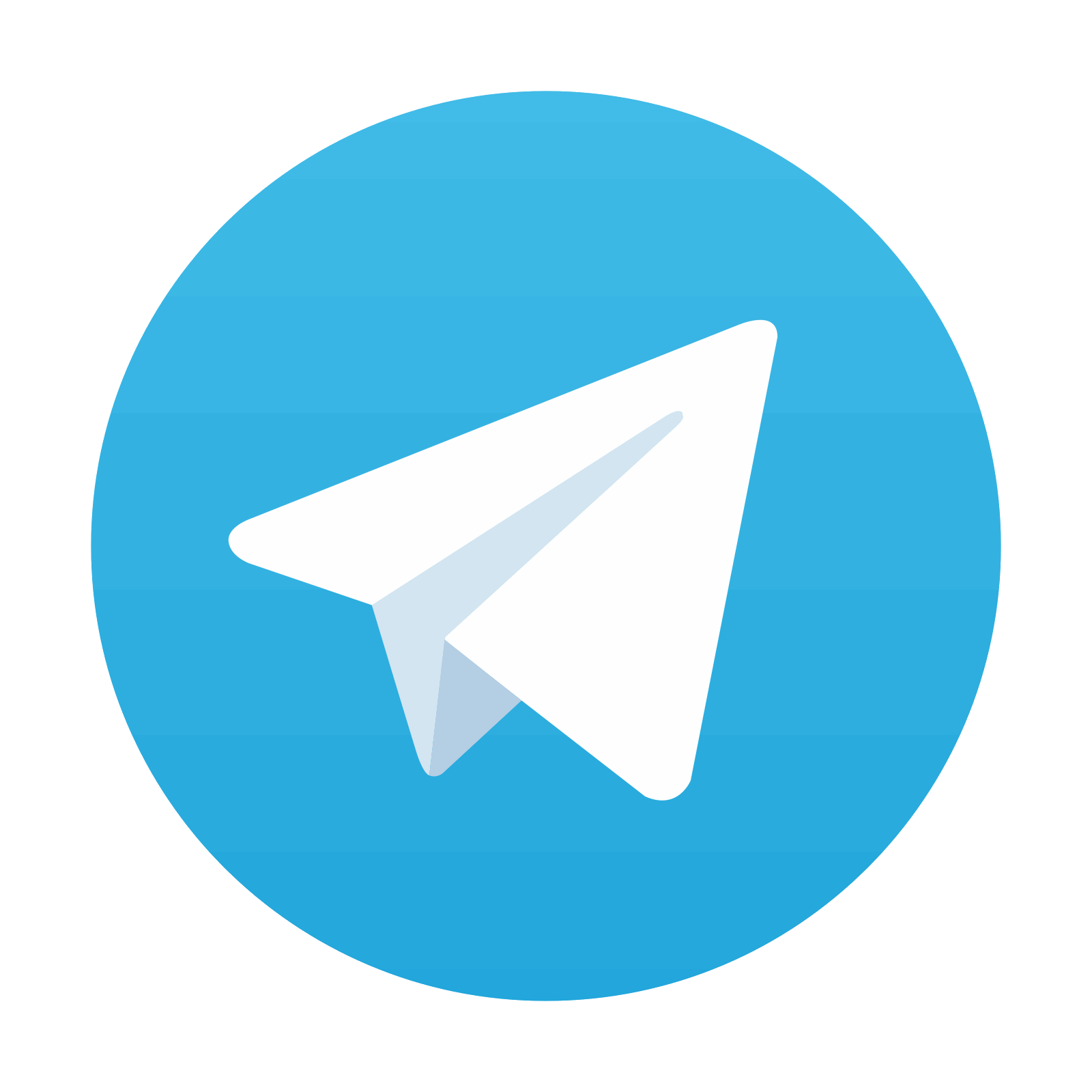
Stay updated, free articles. Join our Telegram channel

Full access? Get Clinical Tree
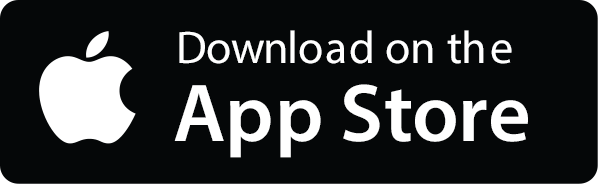
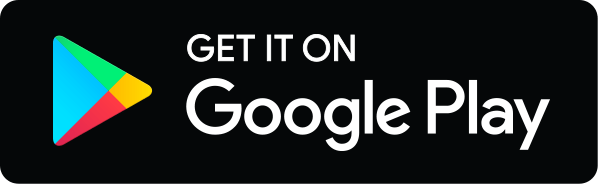
