New Dose Reduction Strategies in CT Myocardial Perfusion Imaging
Ting-Yim Lee, PhD
Aaron So, PhD
Esmaeil Enjilela, PhD
Jiang Hsieh, PhD
▪ Introduction
With the advent of fast scanning capability through the development of slip-ring technology, CT has become the imaging modality of choice in the assessment of coronary artery disease (CAD), particularly in the emergent situation of acute chest pain. Coronary computed tomography angiography (CCTA) is now widely used to image the morphology of coronary vessels to detect severe stenosis that requires revascularization.1,2 and 3 Even with its prevalence in the diagnostic armamentarium, CCTA remains an anatomical technique rather than a functional/physiologic technique for the sensitive detection of functional myocardial ischemia downstream to a stenosis.4,5 and 6 To address this shortcoming of CCTA, myocardial perfusion imaging (MPI) lately has become a focus of research for cardiac CT. In the triage of acute chest pain, the combination of CCTA and MPI promises to be the ideal one-stop shop for the comprehensive anatomical and physiologic evaluation of CAD6 before directing the appropriate patient to revascularization.
However, one main limitation of MPI with CT is the high radiation dose (approximately 20 mSv) from the necessity of repeatedly scanning the heart following injection of contrast (see next section). As such, recently a variety of methods have been developed to reduce the radiation dose of CT MPI. This chapter discusses these methods and illustrates them with examples.
▪ CT Myocardial Perfusion Imaging
To understand better how the new radiation dose reduction methods work, it is useful to describe the three different scanning protocols/methods for CT MPI. First, perfusion in ischemic myocardium is visually assessed from source CCTA images as the relative contrast enhancement with respect to normal myocardium7,8 (Fig. 3-1). Hypoattenuation relative to other parts of the myocardium is taken as ischemia. It is a qualitative assessment and depends on the time of arrival of contrast in the ischemic relative to that in the normal myocardium as well as the timing of the CCTA acquisition relative to the arterial (aorta) concentration. Second, the initial phase of time-density curves (TDCs) is acquired by scanning the heart with prospective ECG gating9,10 (Fig. 3-2). Myocardial perfusion is calculated as the front slope of the myocardial TDC normalized by the maximum value of the aortic TDC.10 Figure 3-2A-C shows an example of MPI in a dog model of acute heart attack. As shown, the method is able to detect stress- (adenosine) induced ischemia. One fundamental assumption of the upslope method is that during the time interval (10 seconds in Fig. 3-2) of its measurement, there is no outflow of contrast from the myocardium—no venous outflow. This is a rather stringent condition, and in general, it is not known whether it will be satisfied in all ischemic conditions. Third, entire TDCs are acquired by either cine-retrospective11 or prospective ECG gating,10,12 and kinetics modeling is applied to derive myocardial perfusion as shown in Figure 3-3. The application of kinetics modeling obviates the need of the no venous outflow assumption, and myocardial perfusion measurements obtained with this last method are the most accurate resulting in one-to-one correspondence with the gold standard microsphere measurements.13 The main disadvantage is the high radiation dose imparted.
As a comparison, the effective dose for a prospectively gated CCTA is about 3.7 mSv,14 while the effective dose of the kinetics modeling method for MPI of the whole heart using either cine-retrospective gating11 or prospective gating10 is 19.4 and 6 to 17.7 mSv, respectively. The upslope method, because of its shorter acquisition window compared to the kinetics modeling method (Fig. 3-2 vs. Fig. 3-3), has an effective dose that is 40% to 50% less. Given the clinical utility of accurate MPI,6 there is an impetus to develop methods to reduce the higher effective dose required by the kinetics modeling. In the following sections, a number of recently developed methods for reduction of radiation dose are presented.
▪ Current Methods for Reducing Radiation Dose in Myocardial Perfusion Imaging
Besides prospective gating that can reduce the radiation dose of MPI by two to three times relative to retrospective gating, and currently, there are three methods under investigation: (a) iterative image reconstruction; (b) principal component analysis (PCA) of TDCs; and (c) compressed sensing (CS) image reconstruction.
Iterative Image Reconstruction
The concept of iterative reconstruction (IR) as embodied in the algebraic reconstruction technique (ART)15 predates filtered back projection (FBP) for the reconstruction of CT images.16 However,
because of the limited computing power of image reconstruction computer workstations and the slow convergence of ART for noisy data, until recently FBP has replaced ART for image reconstruction in all commercial CT scanners. The current resurgence of interest in IR not only stems from the increased computing speed of reconstruction workstations but, more importantly, allows CT scanning at lower radiation dose with similar noise levels and image quality as routine dose FBP.17,18,19 and 20
because of the limited computing power of image reconstruction computer workstations and the slow convergence of ART for noisy data, until recently FBP has replaced ART for image reconstruction in all commercial CT scanners. The current resurgence of interest in IR not only stems from the increased computing speed of reconstruction workstations but, more importantly, allows CT scanning at lower radiation dose with similar noise levels and image quality as routine dose FBP.17,18,19 and 20
The core of IR consists of a loop of backward and forward projection steps that can be iterated a number of times to improve image quality as shown in Figure 3-4. To start the IR, the first step is a normal FBP of the measured (raw) projection data to reconstruct
a CT image of the object. Then, the CT image is forward-projected while simulating CT acquisition by using a priori knowledge of the characteristics of the CT system (e.g., machine geometry, size of detector, and voxel) to obtain synthesized projections. These projections are further modified by the statistics model, which accounts for photon noise, efficiency of individual detector elements, and electronic noise, before they are compared with the measured projections. The differences between the synthesized and measured projections are backward projected to generate an object update candidate (image), which is used to correct the current object estimate (image). Forward and backward projection steps are repeated a fixed number of times or until corrections become very small. GE Healthcare has implemented the full IR algorithm as described above in their Modeled Based Iterative Reconstruction (MBIR) algorithm.21 However, even with current computing speed of a multiprocessor computer (Veo), the reconstruction time for each heart volume is about 10 minutes. For now, this long reconstruction time will limit the use of MBIR for dose reduction in MPI since multiple (approximately 20 to 25) volumes of the heart following contrast injection have to be reconstructed.
a CT image of the object. Then, the CT image is forward-projected while simulating CT acquisition by using a priori knowledge of the characteristics of the CT system (e.g., machine geometry, size of detector, and voxel) to obtain synthesized projections. These projections are further modified by the statistics model, which accounts for photon noise, efficiency of individual detector elements, and electronic noise, before they are compared with the measured projections. The differences between the synthesized and measured projections are backward projected to generate an object update candidate (image), which is used to correct the current object estimate (image). Forward and backward projection steps are repeated a fixed number of times or until corrections become very small. GE Healthcare has implemented the full IR algorithm as described above in their Modeled Based Iterative Reconstruction (MBIR) algorithm.21 However, even with current computing speed of a multiprocessor computer (Veo), the reconstruction time for each heart volume is about 10 minutes. For now, this long reconstruction time will limit the use of MBIR for dose reduction in MPI since multiple (approximately 20 to 25) volumes of the heart following contrast injection have to be reconstructed.
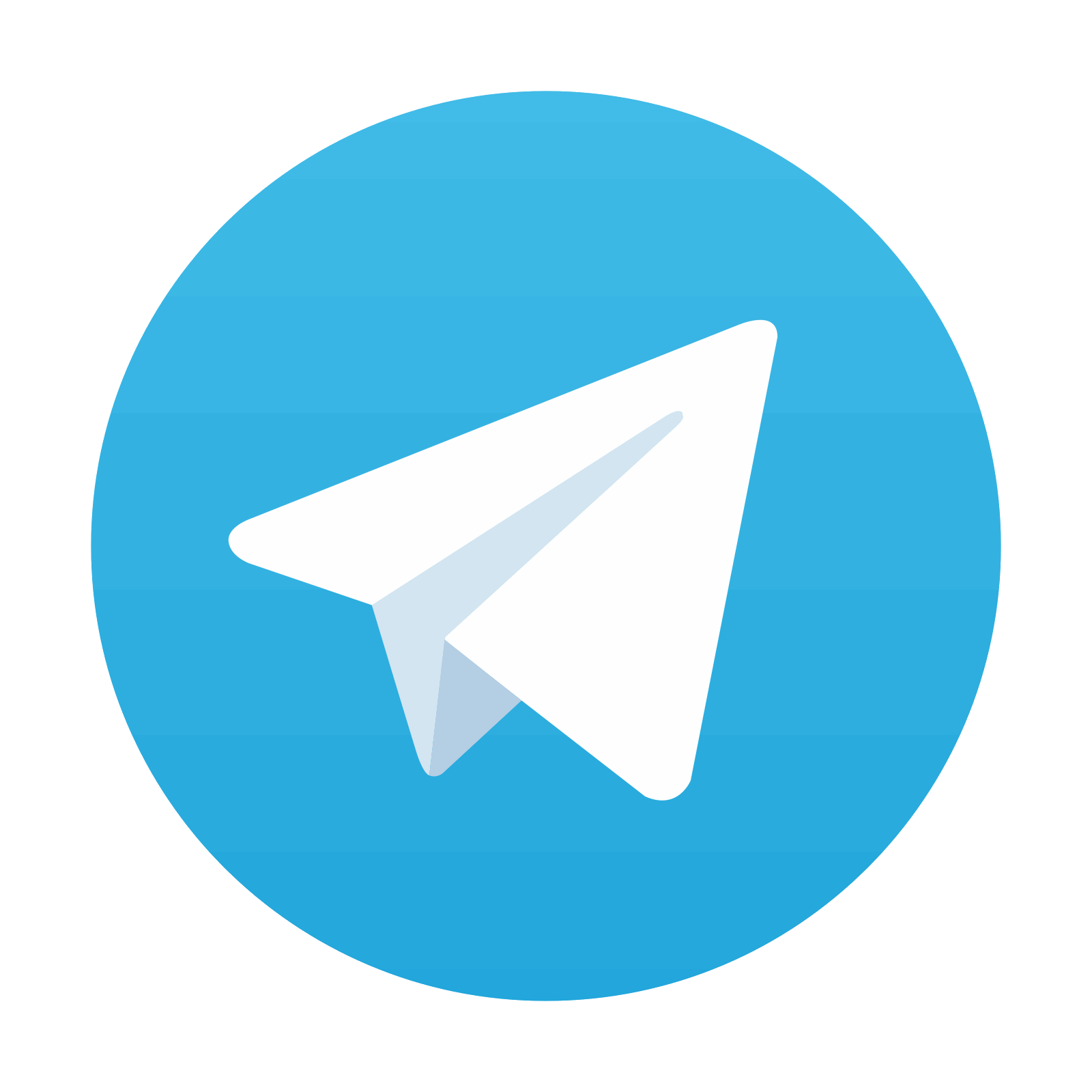
Stay updated, free articles. Join our Telegram channel

Full access? Get Clinical Tree
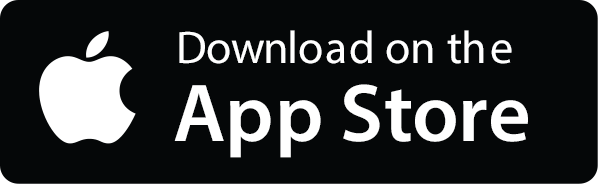
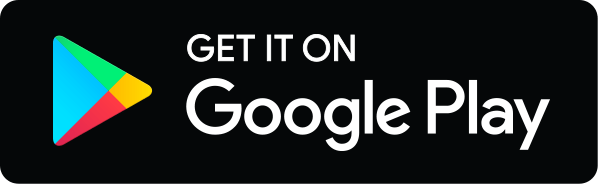