Newer Tracers for Cancer Imaging
Rodney J. Hicks
Although positron emission tomography (PET) has been recognized to have unique capabilities for the evaluation of neurological and cardiac diseases, the recent growth in clinical PET has been largely driven by its multiple roles in oncology. This clinical growth has been based almost exclusively on a single tracer, fluorine-18-fluoro-2-deoxy-D-glucose (FDG). As detailed elsewhere in this book, FDG performs admirably in a wide range of malignancies. However, despite its excellent diagnostic performance, it has recognized limitations. Broadly, these relate to the nonspecificity of glucose metabolic changes for cancer, lack of contrast between physiological and pathological uptake, and low FDG avidity of some cancer types, which limit to varying extent the accuracy of FDG PET in some cancers.
Although pattern recognition, knowledge of typical locations and routes of spread of metastases, and clinical judgment based on all historical and clinical data play important roles in differentiating inflammatory from malignant processes (1), biopsy may be necessary to characterize FDG PET abnormalities definitively. Even when cancer is known to be present, high uptake in adjacent tissues due to physiological processes can reduce lesion contrast and thereby impair the sensitivity of FDG PET for the detection of disease. This is particularly evident in the brain where high glucose use by the normal cerebral cortex can mask the presence of brain tumors (2). Similarly, high background normal organ FDG accumulation can also adversely affect test performance in the liver, bowel, stomach, kidneys, genitourinary system, and in areas of brown fat distribution.
Low FDG avidity can also occur with certain tumors, including some adenocarcinomas of the lung, breast, and prostate, reducing contrast and thereby reducing sensitivity. Despite these considerations, the diagnostic accuracy of FDG in cancer is so good in most clinical situations (3) that there is little incentive to develop new oncologic tracers. These new tracers would first have to match FDG and then be demonstrated to improve on this already high sensitivity and specificity, particularly if proposed to be a replacement for FDG as the workhorse agent for cancer imaging. However, the new tracers in development have the potential to combat the limitations of FDG and establish new applications for PET. More probably, such agents will fill important gaps where FDG’s performance is substantially deficient.
In an era when molecular profiling is identifying specific and mechanistically important genomic and proteomic alterations in diseased cells, a logical progression of PET tracer development is to go beyond the probing of basic substrate metabolism, which is simply an indicator of the extent of viable tumor cells. The trend is toward evaluation of more specific features of cancer biology including proliferation and receptor expression (4). Many conventional nuclear medicine procedures are now performed in the oncology setting that could be replaced with PET tracers and provide superior spatial resolution and contrast, greater convenience, and lower radiation burden.
Most Suitable Radionuclide for New Cancer Imaging Tracers
One of the great theoretical strengths of PET is the availability of a wide range of cyclotron and generator produced radionuclides with varying physical and chemical characteristics (5). Of these, carbon-11 ([11C]), has the greatest flexibility for labeling biological compounds, and it does not perturb the biological behavior of the chemical. However, the short physical half-life of this radionuclide poses significant logistical problems in the clinical setting. Carbon-11 requires rapid synthesis and quality assurance processes to allow administration of adequate quantities for human imaging. It also significantly constrains the number of patients that can be imaged from each synthetic run, thereby increasing the cost per unit dose. Also important is whether the short half-life of [11C] is best suited to the kinetics of the biological process that is to be addressed. Slower biological processes may not be appropriate for [11C] imaging.
Fluorine-18 ([18F]), on the other hand, has an adequate half-life to allow both synthesis and distribution of PET radiotracers, even to sites remote from a cyclotron, but is also sufficiently short to allow relatively low radiation dosimetry. The half-life of 109 minutes is well matched to many physiological processes. Medical cyclotrons involved in the production of FDG routinely produce [18F]-fluoride, and increasing experience with fluorination chemistry enables an ever-wider range of PET tracers to be produced. The low positron energy of [18F] provides high-quality images with modern
PET scanners. Based on these factors, the authors, and others, have focused on evaluating fluorinated PET tracers in oncology applications.
PET scanners. Based on these factors, the authors, and others, have focused on evaluating fluorinated PET tracers in oncology applications.
Generator-produced radionuclides such as gallium-68 may also have a role for facilities remote from a cyclotron (5), particularly for receptor imaging through chelation to peptides (6). Other long-lived radionuclides may have particular advantages in tracing biological processes with slow kinetics. An example of this is the use of iodine-124 to evaluate the localization of monoclonal antibodies in tumors (7).
Substrate Metabolites that Address Recognized Limitations of Fluorodeoxyglucose
PET metabolic imaging has traditionally focused on tracers of cellular substrate metabolism, especially FDG in oncology. Extending this paradigm, multiple radiolabeled amino acids suitable for PET imaging have been developed (8). Up-regulation of amino acid transport and enhanced protein synthesis is a hallmark of cancer. Amino acids are taken up much less avidly in inflammatory lesions, or by the brain, compared to FDG.
PET imaging of amino acid analogues has been seen as a means to address the limitations of FDG with respect to both specificity for cancer and for sensitivity of lesion detection in the brain where high background uptake can mask tumoral radiotracer accumulation. Carbon-11-Lmethionine has been shown to have a sensitivity similar to FDG for detection of various nonbrain cancers, including head and neck and lung cancers. Its specificity is somewhat higher due to a reduced tendency to accumulate in inflammatory lesions. Carbon-11-Lmethionine is one of the few radiopharmaceuticals to compete favorably with FDG in terms of diagnostic accuracy in cancer (9). It is also significantly more sensitive than FDG PET for brain tumors including glioma (10). It is clearly superior to FDG when lesions have lower or similar uptake of FDG compared to normal brain (2).
Unfortunately, as discussed above, the short physical half-life of [11C] makes this an impractical tracer for routine clinical applications. Consequently, there has been a global effort to develop fluorinated amino acids for oncology imaging. One of the most promising of these is [18F]-fluoroethyl-Ltyrosine (FET), which was developed as an alternative cancer-imaging agent (11) and was shown to have similar characteristics to [11C]-Lmethionine in comparative studies (12). However, FET has the practical advantages of using [18F] as the imaging radionuclide. Since this agent demonstrates very low uptake in the normal brain and relatively high uptake in brain tumors (12,13), it provides a more sensitive detection of disease and better characterization of the extent of tumor involvement than does FDG (Fig. 8.25.1).
Although the specificity of FET for ring-enhancing lesions of the brain is superior to that of FDG, it remains imperfect and necessitates biopsy of positive lesions (14). The experience with this agent suggests reasonable uptake in squamous cell carcinomas of the head and neck (15), but the sensitivity for other extra-cranial malignancies has been disappointing (16) (Fig. 8.25.2).
Other fluorinated amino acids are also currently in development (17,18,19). Unless the sensitivity of these tracers can be demonstrated to be comparable to FDG, the higher specificity may not be an advantage since it may falsely reassure clinicians that a negative result in patients with a positive FDG PET scan is indicative of inflammation rather than malignancy. It may also be advantageous to have radiolabeled amino acids for patients who have poor glucose control and for low-grade brain tumors. Thus, there may be a niche for such agents, even if they do not generally replace FDG in
all applications. Recently, nonmetabolizable amino acid tracers have been fluorinated and applied to human imaging, an area of considerable interest and promise.
all applications. Recently, nonmetabolizable amino acid tracers have been fluorinated and applied to human imaging, an area of considerable interest and promise.
Increased sterol synthesis is another potential cancer-imaging target based on substrate metabolism. Tumor tissues have a requirement for increased synthesis of phosphatidylcholine, an important constituent of cell membranes. Increased rates of transmembrane transport and subsequent phosphorylation of choline by the enzyme choline kinase in tumors have been demonstrated.
Carbon-11-choline (20) has been used to successfully image a variety of tumors, including prostate cancer, brain tumors, esophageal cancer, and lung cancer, but because of the short half-life of [11C] (20 minutes), fluorinated analogues are potentially more suitable for clinical studies. Fluorine-18 fluoromethyl-dimethyl-2-hydroxyethyl-ammonium or fluorocholine (FCH) is one such tracer. Preliminary studies suggested that FCH might be more sensitive than FDG for detection of nodal and bone metastases in prostate cancer (21). Subsequent studies in staging and restaging of patients with prostate cancer are continuing to define the potential clinical role of this tracer (22,23,24,25,26,27). The authors’ experience suggests that FCH is more sensitive than FDG PET for detecting sites of relapse in patients with rising prostate-specific antigen levels (Fig. 8.25.3). High uptake in the liver and kidneys is a limitation of this tracer in detecting primary or secondary lesions in these organs. This is not a major limitation for prostate cancer, which seldom spreads to these organs, but it is for breast cancer, which commonly does (Fig. 8.25.4). A discussion of the use of [11C] and [18F]-choline analogues in genitourinary cancer imaging, especially prostate cancer, is present in Chapter 8.18 of this book.
Since FDG avidity of breast cancer is variable, FCH may have a role when there is a strong clinical suspicion of recurrence but a negative or equivocal FDG PET study. Despite the high uptake of FCH in the liver, preliminary results suggest that FCH may also be a useful imaging agent for hepatocellular carcinoma (28), which has variable FDG avidity. As with amino acid analogues, FCH is not taken up significantly in the normal brain, and preliminary studies suggest that this agent might also be useful for brain tumor imaging (29).
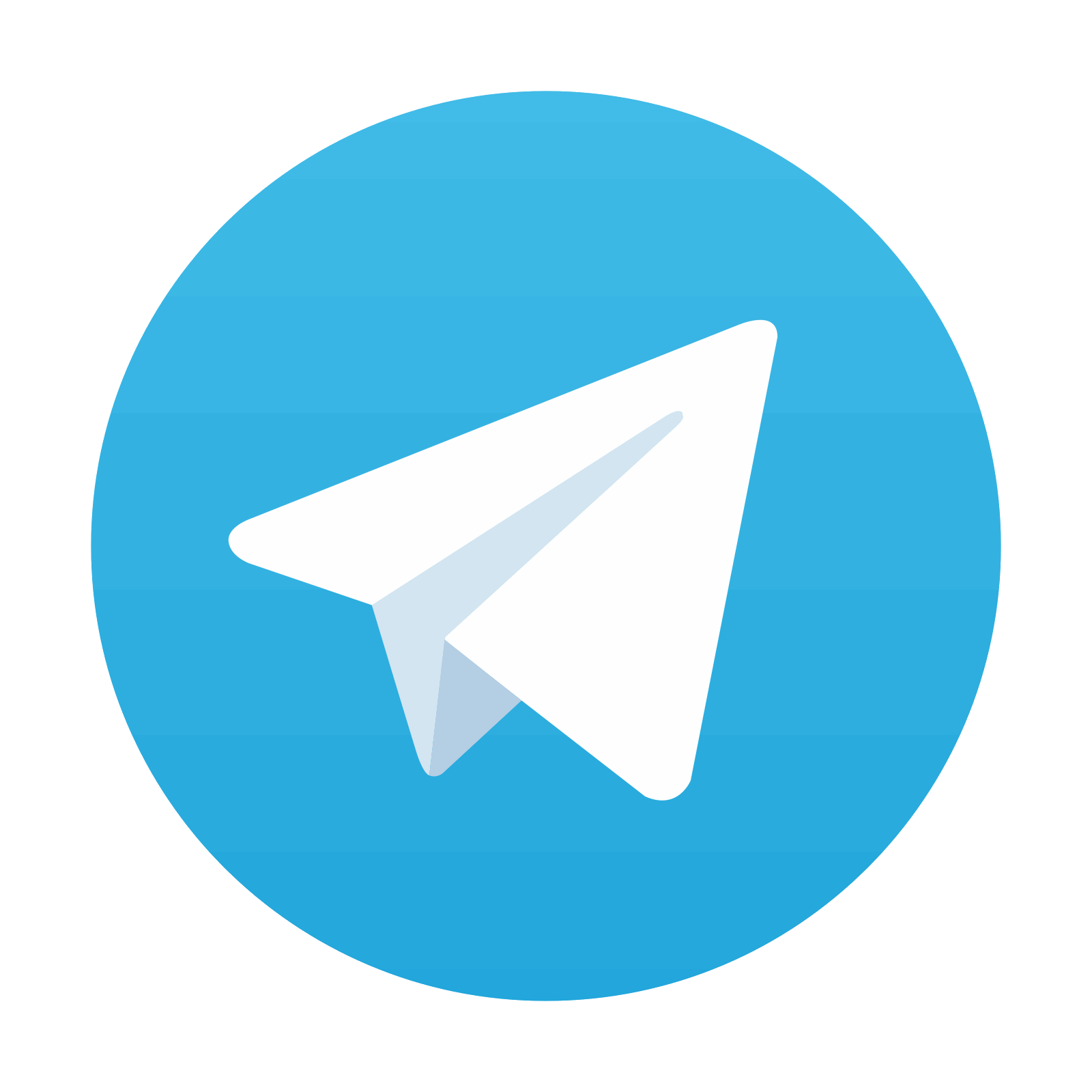
Stay updated, free articles. Join our Telegram channel

Full access? Get Clinical Tree
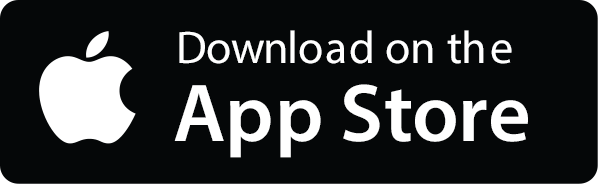
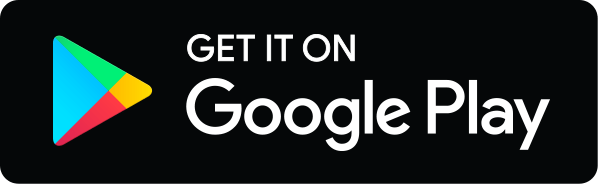