Lymph nodes filter tissue fluid and play an important role in the regulation of immune responses. Antigen sampling from the peripheral tissues is localized to the lymph nodes, where immature dendritic cells in the periphery sample antigen, mature, and migrate from the periphery into the regional lymph nodes. Sinuses within the lymph node facilitate filtering of particulate and antigenic material. Antigen and debris are removed by phagocytosis primarily by macrophages. Some antigen is trapped and processed by dendritic cells for presentation to immune cells, such as B lymphocytes. B lymphocytes that recognize the presented antigen become activated and migrate to the germinal center where they undergo transformation into plasma cells. The plasma cells then migrate into the medullary cords in the center of the lymph node where they produce antibodies, which flow out through the medullary sinuses. Thus the medullary cords are primarily involved in humoral immune responses. T lymphocytes, on the other hand, are located primarily in the paracortical zones, where the T lymphocytes interact with a variety of other immune cell subsets. Antigen specific and memory T lymphocytes in these paracortical areas regulate cellular immune responses.
The thymus is a central lymphoid organ in the mediastinum that is essential for the development of T lymphocytes and the regulation of self- versus non-self antigen recognition by these T cells via the T cell receptor. The lobules of the thymus are partially separated by connective tissue and each lobule possesses a histologically distinct cortex and medulla. (Fig 2a, b) Unlike lymph nodes, the thymus has no afferent lymphatics or nodules. The peripheral zone of dense cortical lymphoid tissue is primarily composed thymocytes or T lymphocytes, while the central medullary zone is predominantly composed of loose connective tissue and characteristic specialized groups of epithelial cells, Hassall’s corpuscles. Other, less prominent, populations of cells, including B cells, macrophages, interdigitating dendritic cells, mast cells, eosiniphils, and Langerhans cells are also found in the thymus. The process of thymic lymphocyte development results in apoptosis of the majority of lymphocytes as part of a regulatory system that eliminates potentially autoreactive T cells before they enter the systemic circulation. The thymus spontaneously involutes with age, leaving a residual of retricular cells, Hassell’s corpuscles, some lymphocytes and connective tissue. Prior to thymic involution, the thymus is the site of the development of the majority of the T cells that populate the thymus-dependent paracortical zone of lymph nodes and the periarterial sheaths in the white pulp of the spleen.
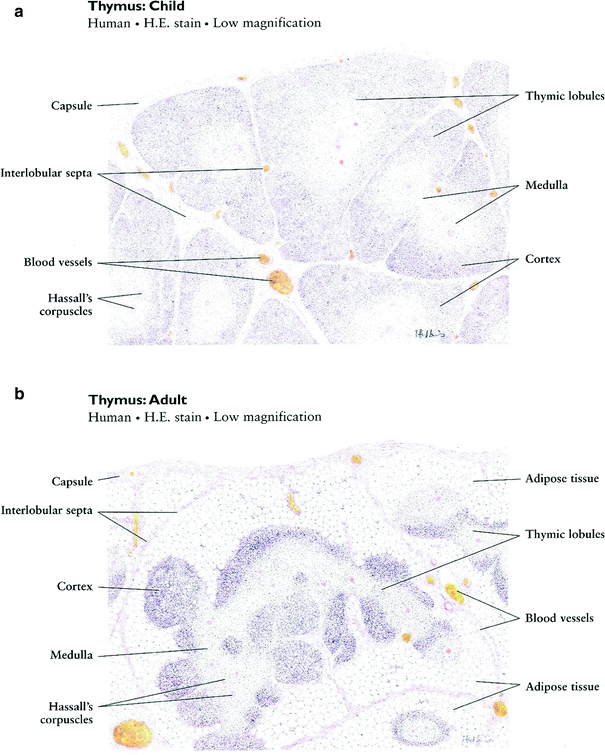
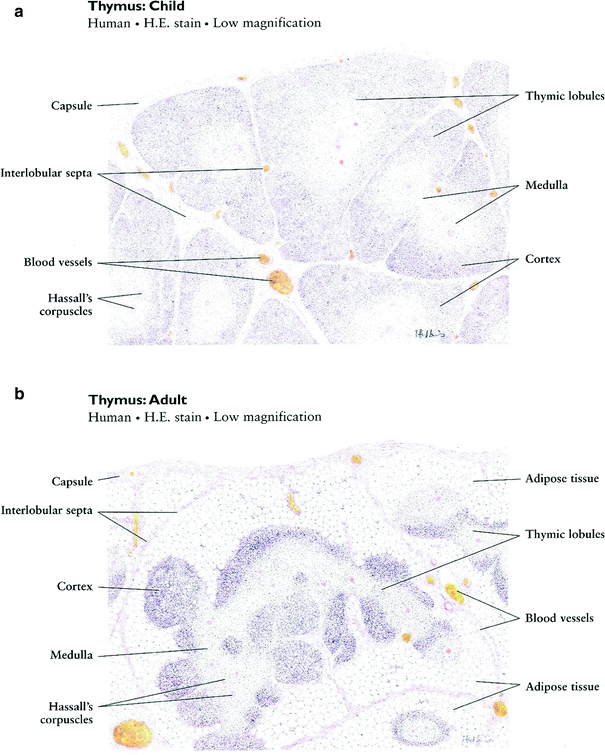
Fig. 2
Histologic structure of: a the thymus of a child, and b the thymus of an adult (with permissions from Zhang 1999)
The spleen is the largest lymphoid organ in the body and plays an important role in the production of blood cells, filtering of erythrocytes, blood storage, and generation of immune responses. The filtering functions of the spleen occur in the red pulp, while the immune functions of the spleen primarily occur in the white pulp. Beneath its thick capsule, the spleen has trabeculae that divide the parenchyma into incomplete sections with (1) lymphatic nodules in the white pulp along vessels, (2) a marginal zone with sinuses, loose connective tissue and many macrophages and dendritic cells between the white pulp and red pulp, and (3) red pulp with sinusoids. Immunity to infectious microorganisms in the systemic circulation is promoted by the interactions between B and T lymphocytes and antigen presenting cells in the white pulp. Even low doses of radiation can significantly deplete radiosensitive immune cell subsets and, to a lesser degree, affect immunocompetency.
Lymphatic vessels connect the lymphoid organs and these lymphatics are therefore critical for the systemic antigen sampling and filtering functions of the lymphoreticular system. Typical lymphatic vessels have an intimal layer (a monolayer of epithelium) with scant adventitia. Peripheral lymphatic vessels are found throughout the body. Afferent lymphatic vessels are found in lymph nodes, but not the thymus and spleen. Lymph circulates from the thymus, spleen, and lymph nodes via efferent lymph nodes. High doses of radiation can not only affect the microenvironment of lymphoid organs, but also lead to changes in lymphatic vessel structure and function. Because patency of the lymphatic channel is important for normal flow of lymph, damage to the lymphatics may lead to regional lympedema.
3 Biology, Physiology, and Pathophysiology
3.1 Cellular Dynamics and the Radiation Response
RT-induced tissue injuries in general have traditionally been divided into two phases: an early inflammatory phase and a late fibroproliferative phase. For certain cell subsets and organs of the lymphoreticular system, there may be an early phase, a recovery phase, and a late phase. The acute phase occurs within hours after radiation exposure and is primarily characterized by apoptosis of lymphoid cells. Depending on the doses and fields involved, this acute lymphoid depletion is followed by reconstitution from marrow-derived hematopoetic progenitor elements and homestatic proliferation of locally surviving immune cells. Ultimately, this process results (From Kataoka 1975) in a renormalization and homeostasis, which may be distinct from the preirradiation lymphoid state, due to long lasting changes related to regional tissue scarring and local microenvironment changes which impair complete reconstitution.
3.2 Physiology and Pathophysiology: Basic Radiobiology of the Immune System
Just as the early clinical applications of radiation preceded the scientific understanding of the cytotoxic effects of radiation, so too has the use of radiation to manipulate the immune system far out paced our understanding of the basic biology of these effects. During the 1970s and 1980s, investigators applied classical radiobiological methods to the study of recently identified immune cell subsets, such as B and T cells. In 1974, Han et al. used T and B cell lines to demonstrate that T and B lymphocytes have distinct patterns of survival after radiation, with T cells demonstrating greater radiation sensitivity than the B cells (Fig. 3a, b). Kataoka in 1975 delineated these differences in native T and B cells in spleens of mice after radiation (Fig. 3b). Radiobiologically, these investigators determined the parameters characterizing the survival curve of B lymphocytes: Do = 200 cGy and n = 1.0. The T lymphocytes, on the other hand, were shown to consist of two distinct subpopulations with respect to their radiosensitivity. The radiobiological parameters of the radiosensitive fraction of T lymphocytes were Dq = 185 cGy, Do = 195 cGy and n = 2–50. The Do value of the radioresistant T lymphocyte subpopulation was practically unmeasurable, and approximately eight percent of the T lymphocytes present in the spleen belonged to this radioresistant subpopulation. Lymphocytes are especially sensitive to radiation induced apoptosis. This correlates with the unique features of lymphocytes that allow the development of a diverse immune repertoire by NHEJ mediated DNA recombination, which is mediated by their distinct expression levels of DNA repair enzymes.
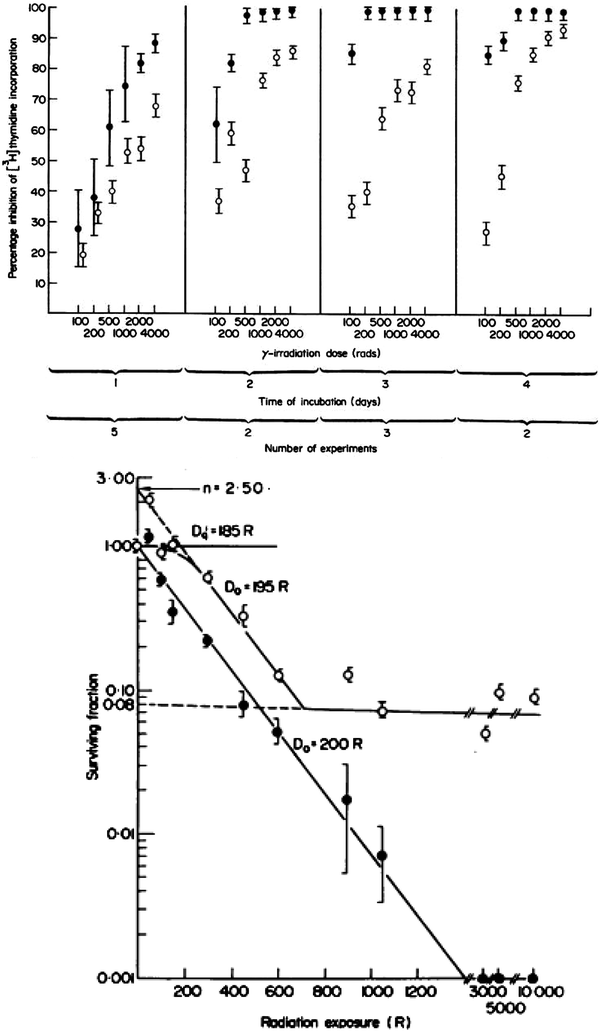
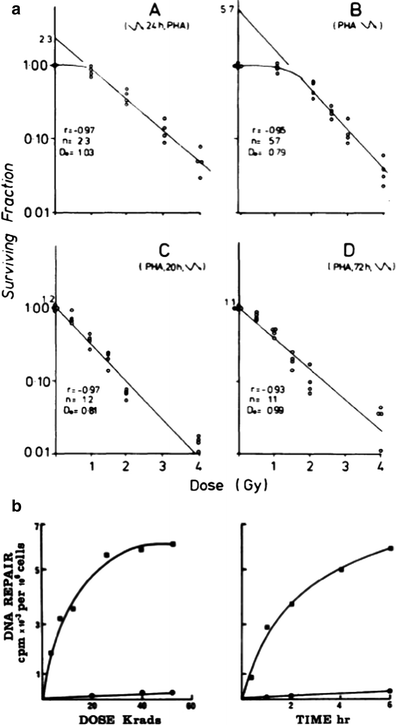
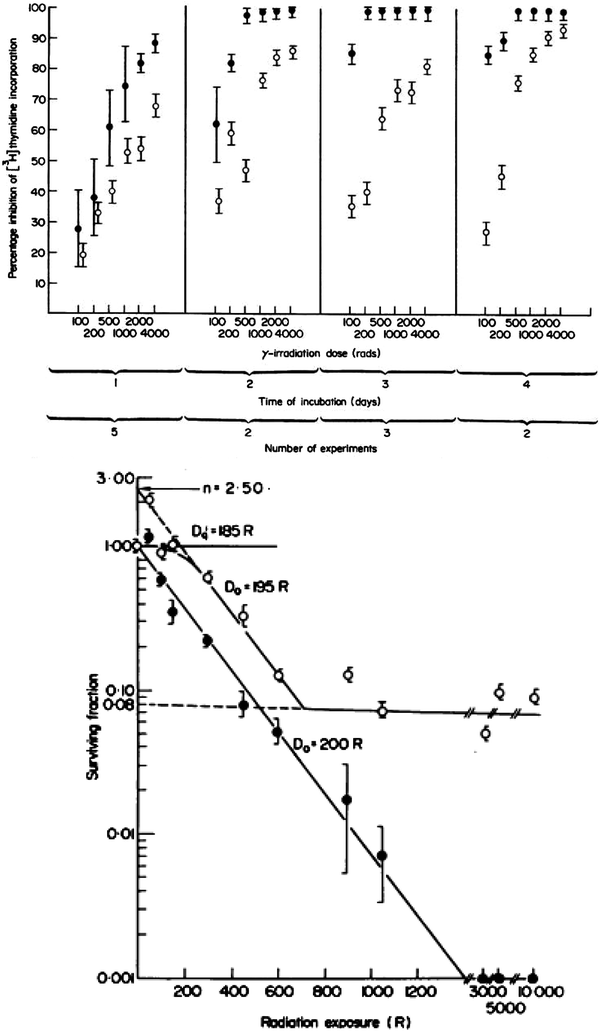
Fig. 3
a Comparison of cytotoxic effect of irradiation on human T (●) and B lymphoid cell lines (○). The mean ± s.d. is shown in each case. b Survival curves of T and B lymphocytes 3 days after various doses of γ-rays. (○) T lymphocytes. (●) B lymphocytes (From Han et al. 1974)
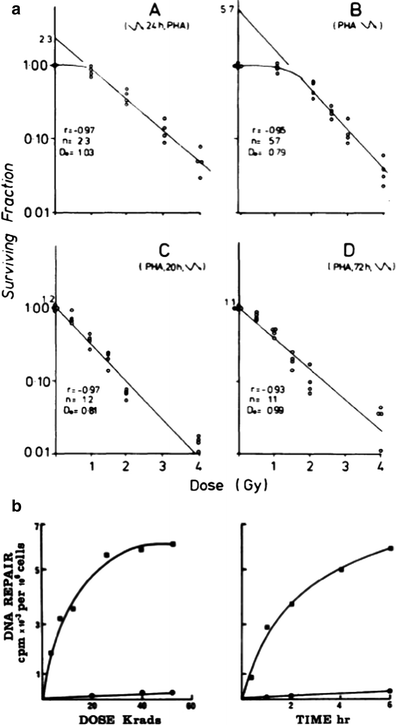
Fig. 4
a Enhancement of T cell survival after irradiation when stimulated by phytohemagglutinin. Survival curves produced for T-colony formation when the cells were irradiated at different stages of activation. (A) Irradiation 24 h prior to PHA exposure; scheme A, (B) Irradiation immediately prior to PHA exposure; (C) Irradiation 20 h after PHA exposure; scheme C, Fig. 1. (D) Irradiation 3 days after PHA exposure; Each point represents the surviving fraction. The results of four experiments are shown. b Enhancement of DNA repair in phytohemagglutinin stimulated cells. (left) DNA repair in human lymphocytes with increasing dose of ionizing radiation. Unstimulated cells (black circle), irradiation (black square). The result presented represent the mean values of 11 experiments (right). Time course of DNA repair in phytohemagglutinin stimulated lympocytes irradiated with 65 Krads. Cells were incubated with phytohemagglutinin for 5 days. Unstimulated cells (black circle), cells stimulated with phytohemagglutinin (black square) (From Kataoka 1975)
Radiation resistance in immune cells also varies with cellular activation. The figure below demonstrates that phytohemagglutinin (PHA) stimulation can enhance T cell survival after radiation (Fig. 4a). Similar enhancement of lymphocyte survival has also been reported after ConA and PWM lectin stimulation. The impact of PHA stimulation on cell survival after radiation relates to enhanced DNA repair capacity in stimulated cells (Fig. 4b). Numerous investigators have also demonstrated that these types of simple lymphocyte survival curves can be used to determine individual persons’ hereditary susceptibility to the effects of radiation, especially in persons with DNA repair deficits.
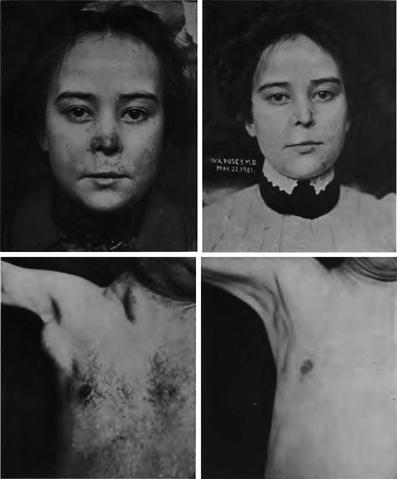
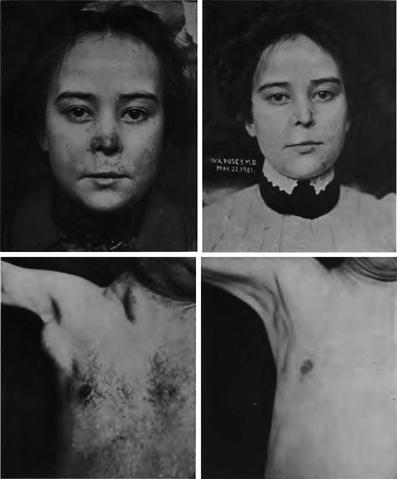
Fig. 5
Early photos of the use of radiation to treat lupus and leukemia (From Pusey and Caldwell 1904)
Early observations of immune stimulation at low doses of radiation have been confirmed in modern studies, using flow cytometry to delineate cell numbers of T, Natural Killer (NK) and NKT cells after low dose whole body irradiation (Ren et al. 2006). After low dose total body irradiation (20 cGy x 4, delivered QOD), despite an initial drop in total monocyte and lymphocyte counts at d 10, total numbers of NK, NKT, and T cells are increased, and show an increase in pro-inflammatory cytokine expression, as measured by IFN-γ and IL-12 (Ren et al. 2006). Interestingly, in addition to NKT cells, there appears to be another relatively radioresistant cell subset, a thymic medullary population, that is associated with the development of regulatory T cells. NKT radioresistance is associated with relatively lower bax:bcl-2 ratios and lower propensities to undergo apoptotic death after irradiation (Yao et al. 2009).
In addition to promoting cytokine production and stimulating cell proliferation and/or recruitment, radiation also modulates the expression of costimulatory and coinhibitory molecules, such as CD28 and CTLA-4, respectively. This change in costimulatory receptor expression is associated with shifts in cytokine expression that would be expected, i.e., IL-10 expression is enhanced concurrently with a rise in CTLA-4 expression (Usui et al. 2006). MLR responses to irradiated DC are also influenced by XRT, but this has only been shown at one dose, 30 Gy (Cao et al. 2004).
4 Clinical Endpoints
Damage to the lymphorecticular system after RT can lead to acute cytopenias and immune compromise, which can increase the risk of infection. If these changes persist long term, they could potentially compromise the ability of the immune system to recognize transformed cells and increase the risk of malignancy in these patients. Laboratory studies in such patients would demonstrate profound lymphopenia, with other cell types less affected. A subset analysis might demonstrate an alteration in the relative proportion of cells, such as CD4 and CD8 T cells.
CTC v4 provides guidelines for the assessment of side effects (Table 1). Few radiological studies have been used to assess lymphoreticular injury. Historically, lymphangiograms, and more recently, other imaging methods are being investigated, but none have demonstrated significant clinical utility. The sensitivity of these radiological modalities varies tremendously, with lymphangiograms being the most useful for showing patency of flow in lymphatic channels, as well as the size, architecture and filling properties of lymph nodes.
Table 1
CTCAE 4.02
Grade | 0 | 1 | 2 | 3 | 4 |
---|---|---|---|---|---|
Immune system disorders – other, specify | None | Asymptomatic or mild symptoms; clinical or diagnostic observations only; intervention not indicated | Moderate; minimal, local or noninvasive intervention indicated; limiting age-appropriate instrumental ADL | Severe or medically significant but not immediately life-threatening; hospitalization or prolongation of existing hospitalization indicated; disabling; limiting self care ADL | Life-threatening consequences; urgent intervention indicated |
Blood and lymphatic system disorders | |||||
Spleen disorder | None | Incidental findings (e.g., Howell-Jolly bodies); mild degree of thrombocytosis and leukocytosis | Prophylactic antibiotics indicated | – | Life-threatening consequences; urgent intervention indicated |
Blood and lymphatic system disorders – Other, specify | None | Asymptomatic or mild symptoms; clinical or diagnostic observations only; intervention not indicated | Moderate; minimal, local or noninvasive intervention indicated; limiting age-appropriate instrumental ADL | Severe or medically significant but not immediately life-threatening; hospitalization or prolongation of existing hospitalization indicated; disabling; limiting self care ADL | Life-threatening consequences; urgent intervention indicated |
Vascular disorders | |||||
Lymph leakage | None | – | – | – | – |
Lymphedema | None | Trace thickening or faint discoloration | Marked discoloration; leathery skin texture; papillary formation; limiting instrumental ADL | Severe symptoms; limiting self care ADL | – |
Lymphocele | None | Asymptomatic; clinical or diagnostic observations only; intervention not indicated | Symptomatic; medical intervention indicated | Severe symptoms; radiologic, endoscopic or elective operative intervention indicated | – |
5 Radiation Tolerance
5.1 Therapeutic Lymphoreticular Irradiation
Areas of lymphoid tissues are encompassed within most standard radiation therapy treatments, whether as a therapeutic target or neighboring structure. Instances of direct targeting of the lymphoid system include total lymphoid irradiation, total body irradiation, splenic irradiation, or the treatment of nodal tissues with or at risk for involvement by tumor.
The entire lymphoid system is irradiated with total body irradiation (TBI), as used as part of a preparatory regimen for bone marrow or peripheral stem cell transplantation. The major lymph node bearing regions and spleen are included in total or subtotal lymphoid irradiation (TLI/sTLI) fields used in transplantation protocols and occasionally for the treatment of organ rejection and severe, medically refractory autoimmune disease. The spleen may be intentionally irradiated (e.g. for the treatment of Hodgkin’s disease or severe splenomegaly in myelodysplastic syndromes), or receive dose during the course of the treatment of certain abdominal or thoracic tumors. Lastly, specific lymph node regions are often treated as part of the PTV for solid tumors or involved or extended field RT for lymphomas.
Depending upon the particular protocol, TBI and TLI fields used for hematopoetic stem cell transplantation (HSCT) conditioning or immunomodulation are usually treated to total doses of approximately 1,200–1,320 cGy and 800–1,200 cGy, respectively in 80–120 cGy fractions, that are often hyperfractionated for TBI. Treatment of Hodgkin’s disease uses higher doses (e.g. 3,000-4,400 cGy) to the spleen and lymph nodes. Fields used for the treatment of lymphomas or solid tumors are standard (total dose and fractionation) for the particular clinical indication and tumor type. Fraction sizes of 150–200 cGy are commonly used in the treatment of lymphoma, and total doses range from four Gy for the treatment of certain cases of radiosensitive and indolent lymphomas, such as follicular lymphoma, to more than 40–55 Gy for the treatment highly aggressive and radioresistant lymphomas, such as nasal NKT cell lymphomas. Irradiation of nodal volumes that are involved with or at risk for involvement with solid tumor metastases commonly follow the dose and fractionation standards for the specific solid tumor primary site. Acute and late toxicity is related to volume, dose, fractionation, and dose rate. Dose rate effects are most dramatic in the setting of TBI, with low dose rates (e.g. 6–25 cGy/min) used for routine TBI for HSCT conditioning.
5.2 Effects of Radiation on lymphoid Tissues
Most immune cells in lymphoid tissue behave as early-responding normal tissues with a high α/β ratio and steep initial slope on radiation survival curves. In contrast, connective tissue and supportive stromal elements in lymphoid tissue microenvironments behave more like late-responding tissues, with low α/β ratios and a shallow initial slope on the survival curve. These characteristics are pertinent to the tolerance of lymphoid tissues to radiation and secondary toxicity. The majority of immune cells in lymph nodes and the thymus are very radiosensitive and undergo radiation-induced apoptosis at relatively low doses, resulting in cellular subpopulation shifts, as described above, and thymic involution. Whole body exposures to doses as low as 25 cGy significantly reduce circulating lymphocyte numbers. Lymphocyte morphological and motility changes have been observed at doses of 5 R and 2 R, respectively. Animal studies demonstrate that a dose of 100 cGy is associated with a 25 % reduction in peripheral lymphocyte levels.
High doses of radiation destroy the parnechyma of lymph nodes and other organized lymphatic tissue (e.g. splenic white pulp, Peyer’s patches, appendix, tonsil, thymus), leaving the stroma, blood vessels, mature plasma cells and phagocytic cells relatively intact. Scattered, but few, lymphocytes remain in the cortex and germinal centers (Congdon 1966). In contrast to parenchyma, lymphatic vessels are relatively radioresistant and are not significantly affected by doses up to 40 Gy (Hauer-Jensen 1990; Engaset 1964; Van den Brenk 1957; Sung et al. 2006). By 7–8 days following high dose irradiation, there is marked dilation of lymphatics (Zweifach and Kivy-Rosenbery 1965). Both inflammation and direct damage to lymphatic vessels may contribute to fibrotic constriction of lymph vessels and obstructed lymphatic flow (Hauer-Jensen 1990). Lymphatic regeneration is inhibited after irradiation with doses in excess of 20 Gy (Van den Brenk 1957), but parenchyma can regenerate, providing the microenvironment is intact. Collections of cortical lymphocytes appear first, followed by germinal centers (Congdon 1966). Some evidence from adult mice suggests that functional regeneration of the lymphoid system after irradiation requires the presence of an intact thymus (Cross et al. 1964).
After splenic irradiation at doses commonly used to treat lymphoma, patients are functionally asplenic. Acutely after splenic irradaition, there is decreased celluarity, alterations in the relative composition of constituent splenic cell populations, and decreased responsiveness to mitogen (Harrington et al. 1997). Late effects of splenic irradiation were studied in an autopsy series of patients 1–8 years following a mean dose of 3,899 cGy fractionated over 5–6 weeks. Most of the irradiated spleens were small, with thick capsules, often with focal hemorrhage. There was also collapse of parenchyma, close opposition of trabeculae and diffuse fibrosis of the red pulp, with lymphocyte depletion (Dailey et al. 1981). These findings have profound implications in terms of immunocompetency, the ability to resist infection and the potential efficacy of immunization.
The effects of radiation on cellular and humoral immune parameters have been studied in some detail in the survivors of atomic bomb and nuclear accidents, especially in survivors from Nagasaki and Hiroshima followed in the adult health study samples (AHS) (Akiyama 1995). (Tables 2, 3
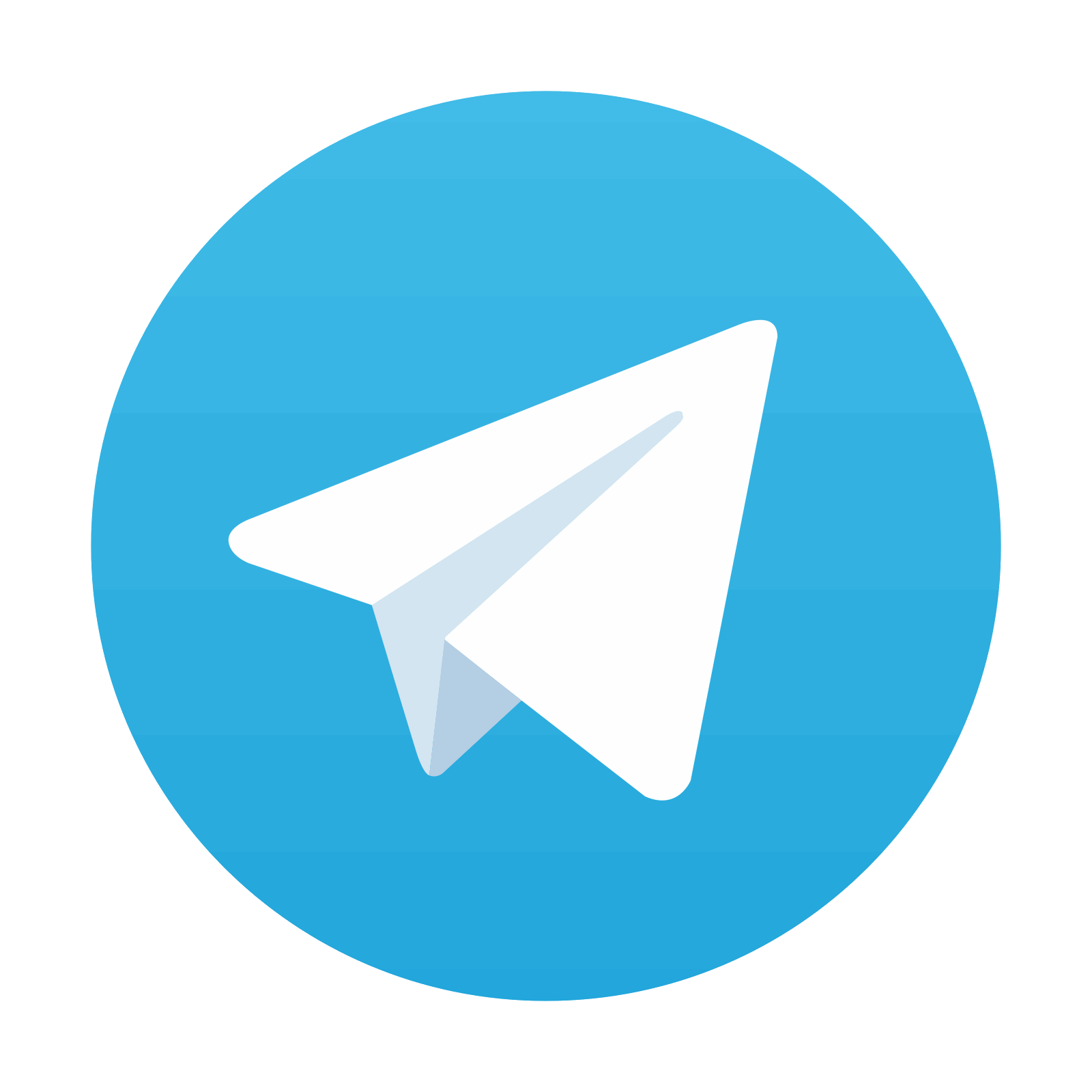
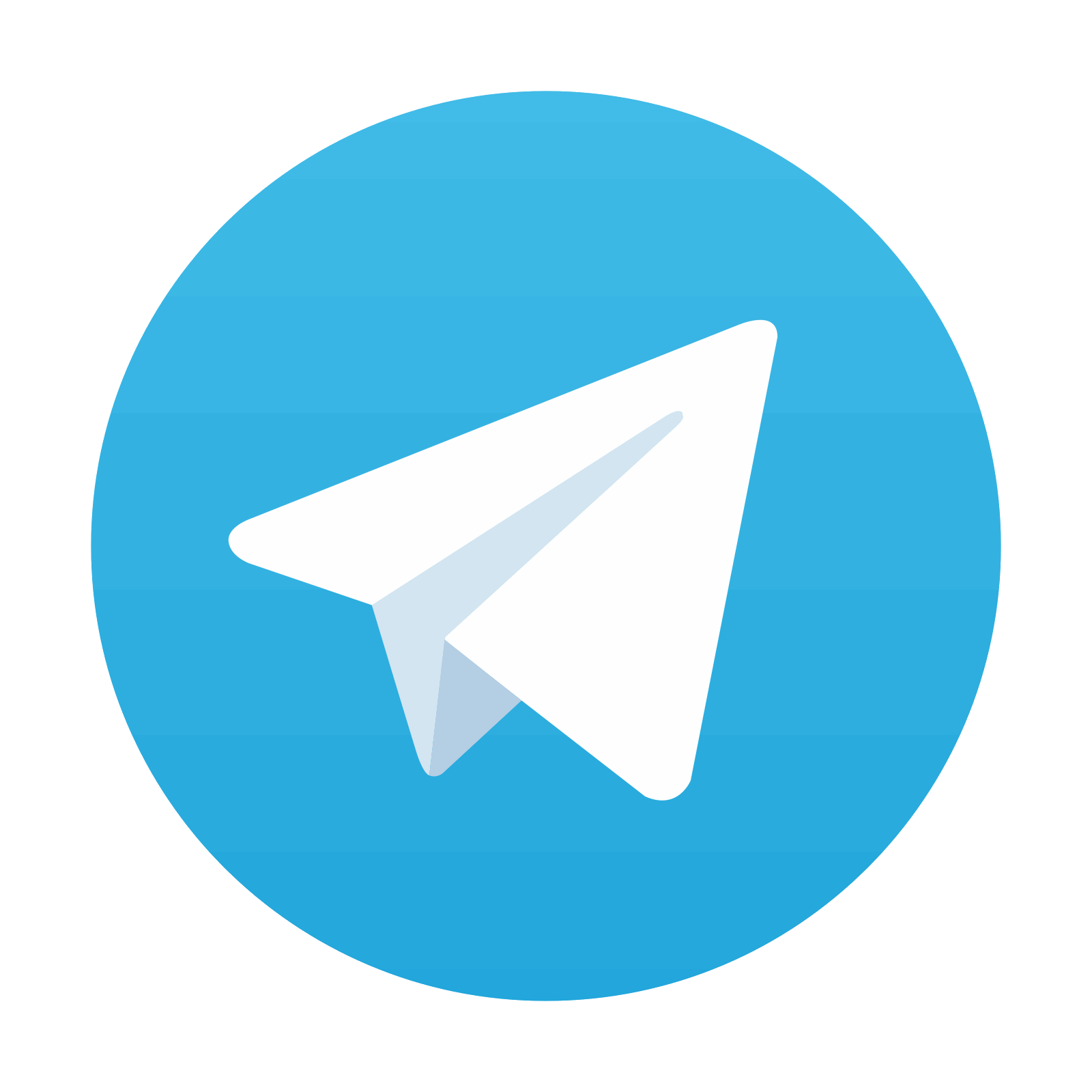
Stay updated, free articles. Join our Telegram channel

Full access? Get Clinical Tree
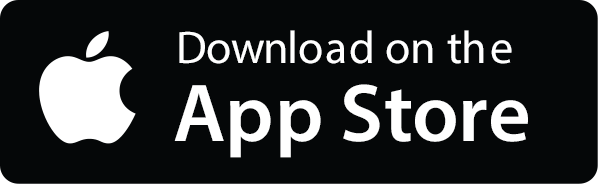
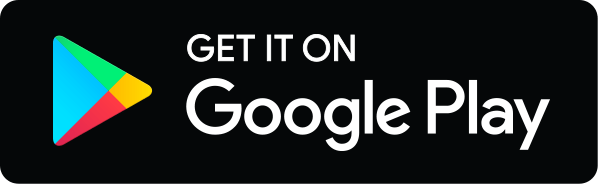