Nonhepatic Gastrointestinal Malignancies
Daniel T. Chang
Devin S. Schellenberg
Albert C. Koong
The delivery of radiotherapy (RT) has undergone enormous change over the last decade. Intensity-modulated RT (IMRT) is playing an ever-increasing role in many treatment sites because of its ability to deliver radiation in a highly conformal manner around targets while sparing nearby critical structures. Malignancies of the gastrointestinal (GI) tract are in ideal anatomic locations to apply IMRT and stereotactic body RT (SBRT) techniques because of the proximity of dose-limiting structures. The well-known danger associated with increasing conformality is the risk of excluding tissues at risk of harboring either gross or microscopic disease. Therefore, a more sophisticated understanding of anatomy and patterns of tumor spread is needed to distinguish target tissue from normal structures. However, even with perfect delineation of target structures on a planning study and optimized dosimetry, the actual delivery of the RT plan on a patient poses significant challenges due to the mobility of organs and tissues during both the intrafraction interval and interfraction interval. Because of uncertainty in localization due to patient positioning, respiratory motion, and internal organ filling, the treatment of GI malignancies is also ideally suited for image-guided RT (IGRT).1
Despite this strong justification, so far, the use of IGRT for nonhepatic GI malignancies has been scant when compared to hepatic, lung, or prostate malignancies. Few institutions have published results incorporating novel imaging modalities or daily imaging verification into treatment delivery. These studies are essential to gain a greater understanding of organ motion and quality assurance measurements and allow further reduction in setup uncertainty and improved radiation planning. What follows is a rationale for IGRT studies in GI malignancies, as well as an overview on the current uses of IGRT in nonliver GI malignancies at our institution.
DIFFICULTIES OF IMAGE-GUIDED RADIATION THERAPY STUDY IN GASTROINTESTINAL MALIGNANCIES
If we compare the efforts made in improving radiation delivery to prostate cancer (IMRT), lung cancer (SBRT), and breast cancer (partial breast irradiation), it is remarkable that colorectal and pancreas cancers (representing the third and fourth most common causes of cancer death in both men and women) have undergone few significant advancements with regard to RT techniques. The reasons for the limited use of IGRT in the GI setting are likely multiple, and prior to reviewing the role of IGRT in GI malignancies, it is worthwhile to examine the unique challenges associated with this disease site and why IGRT is underused in this setting.
The successful treatment of many GI malignancies (esophagus, stomach colorectal, etc.) usually relies on combination therapy, often with surgery, chemotherapy, and radiation all being given to enhance survival rates. This paradigm is different than the treatment for early-stage lung cancer, early prostate cancer, or liver tumors, where single-modality therapy is standard. For the latter cases, it is presumed that a large enough dose of radiation (often without chemotherapy) will lead to local disease control. In the GI setting, the use of radiation is often done with the goal of reducing tumor size or sterilizing microscopic disease, and as such, larger, more toxic doses of radiation are not as crucial. However, just as improvements in surgical technique and the emergence of molecularly targeted chemotherapies have contributed to improvements in patient survival, advances in RT techniques should also lead to substantial gains in clinical outcomes following RT.
GI tumors often require radiation to both the primary site and the draining lymph nodes. This not only greatly increases radiation volumes, but also makes the area of treatment more subject to variation among individual radiation oncologists. Because of the imprecision in contouring pelvic lymph nodes, precisely accounting for daily variation is judged as less critical when the variation in target definition is high.
As with the lung and liver, the organs of the upper GI tract tend to move with respiration (esophagus, stomach, liver, and pancreas). The movement of lower GI organs varies daily and often in an unpredictable manner due to rectal filling or bladder filling.
The abdomen is filled with relatively radiosensitive organs. Many of these organs are thought to function in a serial manner. These organs tend to be hollow tubular structures
(esophagus, small bowel, large bowel) requiring continuity for optimal function. Therefore, avoiding radiation doses that exceed the tolerance of these organs is essential to avoid toxicity. Other organs, such as liver and kidney, are composed of independently linked subunits and function in a parallel manner. These tissues have significant reserve, and the volume of irradiated tissue, rather than the absolute dose, tends to be the critical factor. These considerations must be taken into account to optimize RT of the GI tract. The radiosensitivity of these abdominal organs has restricted the use of higher, more ablative doses of radiation with conventional techniques, and the addition of surgical resection has typically obviated the need to pursue dose escalation in most cases.
Referral patterns and treatment standards are also less supportive of IGRT of GI malignancies. For successful treatment, a clear area to be treated must be identified. Postoperative RT for esophagus or stomach cancer involves the treatment of ambiguous nodal volumes and uncertain tumor bed locations. The same is often true for postoperative pancreatic and rectal cancers. Although respiratory gating may help to reduce these volumes in upper GI malignancies and cone beam CT (CBCT) scans may be effective in lower GI malignancies, there will always be a margin of uncertainty with target delineation around the original tumor bed, which diminishes the benefits of IGRT.
Despite these limitations, IGRT has the potential to not only improve upon RT delivery, but also to revolutionize the global therapeutic approach for GI cancers. IGRT has facilitated increased dose-intensity for prostate, lung, and liver cancer resulting in improved local control compared to historical data. As local control is optimized with dose escalation, patients may ultimately be able to avoid traditional surgical procedures of the GI tract.
RADIATION TREATMENT PLANNING AND IMAGE-GUIDED RADIATION THERAPY
Having discussed some of the difficulties with IGRT for GI malignancies, it is worthwhile to examine the preparation and planning needed to achieve optimal delivery of IGRT. The overall goal is to improve accuracy and thereby reduce treatment margins around clinical target volumes (CTVs). Optimizing RT precision is achieved primarily not only by reducing uncertainty in patient positioning and compensating for organ motion, but also by improving the ability to distinguish target volumes from normal tissue. With these goals in mind, IGRT preparation should begin before the treatment planning scan. Although a complete discussion regarding the spectrum of imaging modalities is beyond the scope of this chapter, computed tomography (CT), magnetic resonance imaging (MR), and positron emission tomography (PET) imaging are the most commonly used modalities in RT treatment planning. Often, more than one imaging modality is beneficial for optimal definition of target volumes. Another important decision is whether fiducials are required as a surrogate for tumor position during RT.
Four-dimensional (4D) CT has been shown to be extremely effective in lung cancer to accurately determine the internal target volume (ITV) margin2, 3, 4 and to reduce contouring margins. Similarly, tumors of the upper abdomen will move throughout the respiratory cycle, and this motion should be compensated for during treatment planning. Imaging throughout respiration is preferred rather than considering only the extremes of end expiration and inspiration, which will not account for tumor deformation during respiration or hysteresis. In general, respiratory-gated treatment is typically delivered during expiration phase because patients spend a larger percentage of their normal breathing cycle in end expiration. Furthermore, the greater percentage of time spent in the expiratory phase allows gated treatments to be delivered more quickly.
In the abdomen, day-to-day patient positioning is variable, and tattoos, along with Alpha cradles, Vac-loc bags, or Body-FIX systems (Medical Intelligence, Schwabmuenchen, Germany), are not reliable enough for optimal accuracy. Even with BodyFIX systems, bony landmark setup deviations >5 mm occur in almost 30% of patients.5 Also, it is nearly impossible to visualize GI tumors with orthogonal x-ray films, ultrasound, or fluoroscopy. Daily imaging relies on correctly aligning to bony anatomy, which can be misleading for the setup of mobile internal organs such as lower esophagus, stomach, and pancreas, where tumor motion can be significant. Therefore, substantial ITV margins would be needed. Some investigators have used daily ultrasound for targeting of tumors in the upper abdomen. This technique relies on imaging of nearby guidance structures such as the surrounding vasculature and was reported to decrease daily setup error.6 However, this technique does not compensate for dynamic changes in tumor position that occurs during treatment due to respiratory-associated motion. Using a surrogate for respiratory motion, such as the diaphragm, does not seem to correlate well with lung tumor motion7 or pancreas motion,8 and further investigation in this area is warranted.
CBCT, although clinically useful for some tumors,9, 10, 11 does not provide sufficient image quality to be of benefit for tumors of the upper abdomen. In particular, because of the time required for acquisition, respiratory motion significantly degrades the quality of these scans.12 Although bony and some soft tissue anatomy can be accurately positioned with reference CBCTs, the current technology is not adequate to correct for respiratory-associated tumor motion. Moreover, normal tissue motion, such as peristalsis and transit of bowel contents, cannot be compensated for in any clinically meaningful manner.
In summary, there is no widely accepted standard of practice with regard to IGRT or even image verification in GI malignancies. Although bony landmarks are the most common mechanism used for patient alignment, their position is not constant in correlation with abdominal organs, and future treatments may rely heavily on determining abdominal organ location and movement through the use of tumor-implanted fiducials, CBCT, ultrasound, or other modalities.
Having considered these issues, we devote the remainder of this chapter on how we have chosen to implement IGRT into our daily practice for esophagus, pancreas, and gastric tumors.
ESOPHAGUS
Esophagus cancer has the well-recognized potential of submucosal spread several centimeters along the esophagus and periesophageal lymphatics.13,14 As a result, CTVs typically extend 5 cm inferior and superior to the primary tumor,
causing significant amounts of normal tissue to be irradiated. In addition to the margin required for subclinical disease, further margins might be required to account for setup and motion uncertainty, which contributes even further to potential treatment morbidity.
causing significant amounts of normal tissue to be irradiated. In addition to the margin required for subclinical disease, further margins might be required to account for setup and motion uncertainty, which contributes even further to potential treatment morbidity.
Because the esophagus runs through the thorax, the position of tumors arising from it is influenced by respiratory motion. However, the location along the esophagus is likely an important factor as well. Tumors arising in the cervical and upper thoracic portions are more fixed due to the presence of bone, muscle, vessels, and soft tissue. Inferiorly in the thorax, the tissue space is much less confined, and the distal portion would likely have the most mobility due to its close association with the diaphragm. Yaremko et al.15 used deformable registration on 31 consecutive distal esophageal cancer patients who underwent 4D CT scanning to measure the magnitude of change of the tumor during the respiratory cycle. They found that the tumors move, on average, 0.13 cm laterally, 0.23 cm anteriorly, and 0.71 cm inferiorly.15 More importantly, they found that margins of 0.8 cm radially and 1.75 cm inferiorly would be needed to account for the motion seen in 95% of the study population. These margins would be needed in addition to margin to account for setup error, which could be up to 1 cm or more. Therefore, IGRT may have an important impact in reducing what are already large target volumes and improving treatment-related morbidity.
The first step for treatment planning is accurate delineation of the gross tumor volume (GTV) on the planning scan. Oral contrast (either in combination with fluoroscopy or given immediately prior to CT scanning) may be used to delineate the stricture caused by the tumor. However, submucosal spread above and below the area of constriction is common. PET imaging has now become a common staging procedure to evaluate for metastatic disease. Its role in RT planning has also been investigated. Moureau-Zabotto et al.16 found that incorporating PET in defining GTV resulted in a decrease in size in 35% of cases and an increase in size in 25% of cases when compared to CT alone. Furthermore, they found that treatment planning based on the PET-defined GTV resulted in changes to the percentage of lung volume receiving 20 Gy or more (V20), an important parameter correlating with lung toxicity.17,18 Leong et al.19 also studied the use of PET with tumor delineation and found that using CT alone would have excluded PET-avid disease in 69% of the study population and would have led to a marginal miss in 31% of the study population. The tumor extent in the superior-inferior direction deviated the most between the CT-based GTV and the PET-based GTV, showing a difference of 75% of the time superiorly and 81% of the time inferiorly. In addition, PET scanning found unappreciated lymph node disease in 21% of the patients.
Although the use of PET scanning may facilitate a reduction in the target volume, other IGRT techniques are also important in limiting toxicity. Due to the potential motion of the esophagus with respiration, 4D CT scans can track the tumor throughout the respiratory phase and can be used to define the ITV rather than using uniform expansions. Alternatively, respiratory gating may minimize or eliminate the ITV margins by treating only during specific phases when the tumor is relatively immobile (i.e., respiratory gating).
Our general practice is to use 4D CT scanning for esophageal cancer treatment planning. When treating preoperatively or definitively, we also obtain a PET/CT for optimal target delineation. Although a conventional three- to four-field technique is widely used, our preferred treatment approach is IMRT due to its ability to reduce the dose to the lung, spinal cord, and heart (unpublished data). Because reducing the intrafraction motion is effective in reducing target volumes, we use respiratory gating with the gating window typically centered around the end expiratory phase, particularly for distal esophageal tumors. Figure 14.1 shows the motion of the lower esophagus and upper abdomen at end expiratory and end inspiratory phases on a 4D CT scan. Patients are treated in an Alpha cradle, and verification films are typically done twice a week using orthogonal megavoltage films aligning to bony anatomy. We generally expand the CTV 5 to 10 mm to account for setup variability. The standard dose prescription is 50.4 to 54 Gy at 1.8 Gy per fraction.
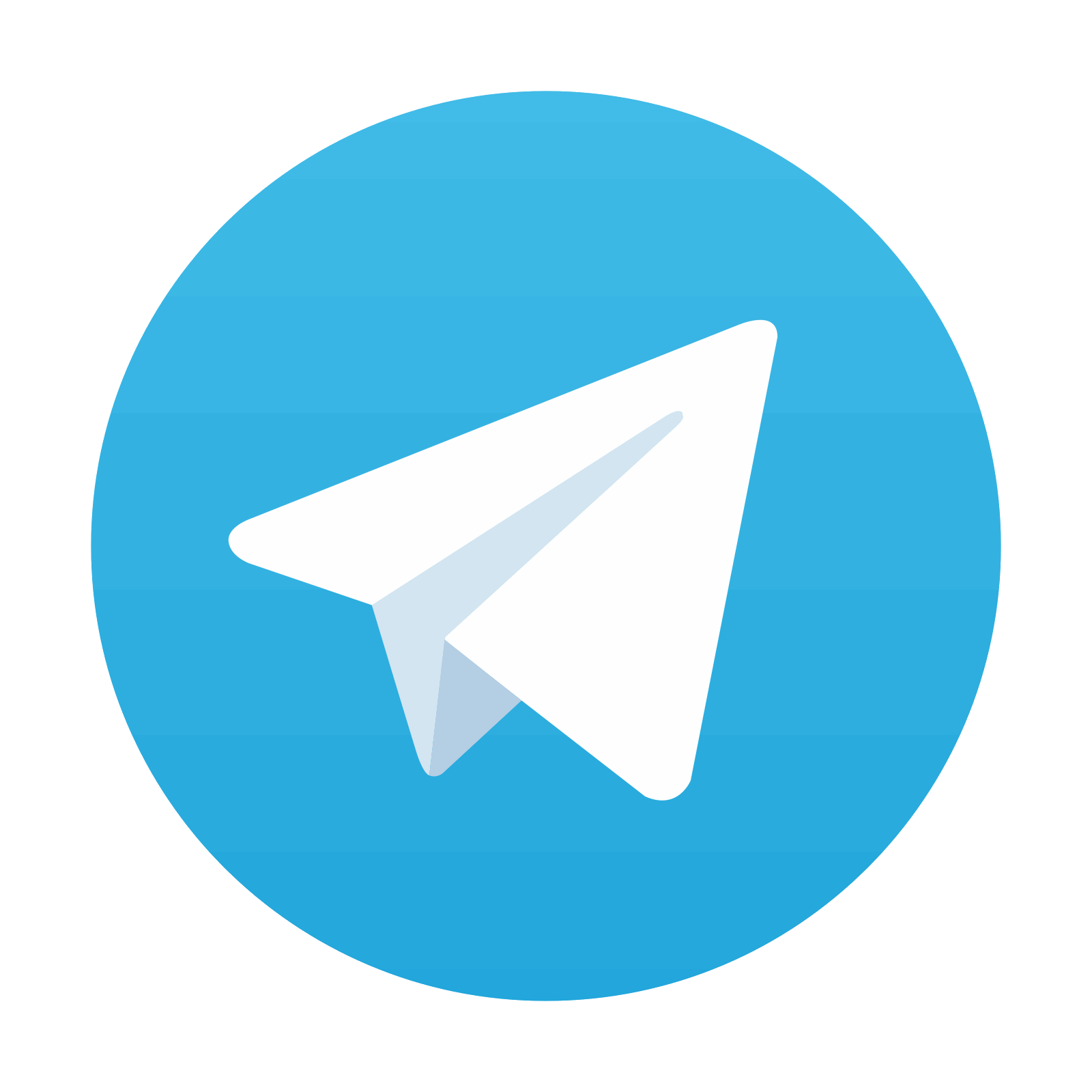
Stay updated, free articles. Join our Telegram channel

Full access? Get Clinical Tree
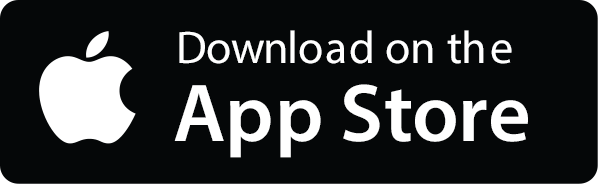
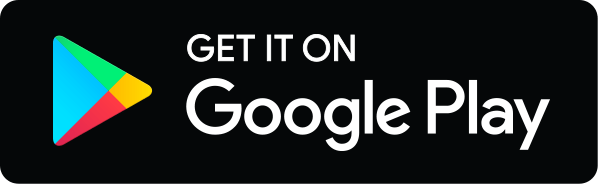