

bottom of the developing third ventricle, GABAergic neurons generated, including cells to the preoptic area, amygdala, posterior globus pallidus, and cortex); (d) dorsal neocortical germinal zone (located in walls of developing lateral ventricles, glutamatergic neurons destined for the neocortex) (1,2,3,4,5,6,7). Neurons migrate from these germinal zones through the developing hemispheres to form the cerebral cortex, initially in an incomplete form that is often called the preplate. As the neurons migrate, they develop axonal connections with other cortical and subcortical (subplate) neurons. The axons form a prospective zone of white matter that is called the intermediate zone because it is in the region intermediate between the ventricular zone and the developing cortex. In addition to afferent and efferent axons, the intermediate zone contains migrating neurons and oligodendrocyte progenitor cells (8). Between the preplate and the intermediate zone is a transient area of loosely packed and loosely organized neurons that form temporary neuronal circuits, particularly with the thalamus (9); this zone is known as the subplate. The subplate is largest at the 30th postconceptional week (10). At that time, it is about four times thicker than the cortex, occupying up to 45% of the telencephalon, and is easily seen on fetal MRI as an area of T1 hypointensity and T2 hyperintensity between the intermediate zone and cortex (10,11,12). It gradually disappears after the 30th gestational week, as definitive cortical connections are established (10,11) but remains functional, in part, possibly for as much as the first half of the first postnatal year (10). Details of the development of the hemispheres are described in more detail in Chapter 5. For the purposes of the discussion to follow, it is sufficient to understand that the occipital pole begins to develop at about the 43rd gestational day and the temporal pole at approximately the 50th gestational day. During the early weeks of gestation, the surfaces of the cerebral hemispheres are smooth. The fetal sulci appear in an orderly sequence; the phylogenetically older sulci appear first, and the more recently acquired sulci appear later. The principal sulci and gyri form the characteristic pattern of the human cortex that can be identified in the full-term infant (Table 2-1). The primitive sylvian fissure, the earliest fetal sulcus, is usually present when the fetus is imaged in the fourth gestational month. The next sulci to appear are the calcarine, parieto-occipital, and cingulate sulci during the fifth month (by 20-22 weeks); the Rolandic (central), interparietal, and superior temporal sulci that appear toward the end of the sixth month (by 25 weeks); and the precentral, postcentral, superior frontal, and middle temporal sulci that appear during the seventh gestational month (24-28 weeks) (13) (Fig. 2-1 and Table 2-1). In the medial temporal lobe, the hippocampal sulci are variable and often asymmetrical; asymmetry is also often seen in the formation of the collateral sulcus (14). Because sulcal formation occurs so late in gestation, imaging studies of premature infants show sulci that are shallow and few in number. It is, therefore, important to know the postconceptional age of a child before assessing the sulcal pattern. Otherwise, a false diagnosis of lissencephaly may be made. In addition, as will be discussed in more detail later in the chapter, sulcation, myelination, and corpus callosum development are often delayed in prematurely born neonates compared to fetuses of the same postconceptual age.
|
about brain myelination. CT allows fairly good information about sulcal development but gives a poor assessment of myelin development and it exposes the baby to ionizing radiation; it is not recommended for brain imaging in the fetus, neonate, or infant unless other methods are unavailable. MR allows excellent assessment of myelination, sulcation, and chemical maturation and is the imaging technique of choice for evaluation of normal development in the neonate and infant.
central germinal matrix (Fig. 2-2C-E). Although previously believed to represent migrating glial cells, this layer has recently been identified as the intermediate zone or the developing fetal white matter (11). Projection and commissural axons are present, as are some late migrating neurons and many migrating astrocyte and oligodendrocyte precursors (42). Immediately peripheral to the intermediate zone and deep to the cerebral cortex is a region of T1 hypointensity/T2 hyperintensity (Fig. 2-2F), known as the subplate, a region composed of loosely spaced neurons where thalamocortical afferent axons, basal forebrain cholinergic afferent axons, and callosal and ipsilateral corticocortical axons accumulate for a variable period before entering the cortical plate to establish definitive thalamocortical and corticocortical synapses (10,43,44,45). These zones are most prominent in younger fetuses (<20 gestational weeks). Before 20 weeks, the sylvian fissures are
extremely shallow and no operculization can be identified. The basal ganglia can be identified and are intermediate in signal between the darker cortex and germinal zone and the lighter white matter (Fig. 2-2D). The cerebellum remains quite small at this age, barely bigger than the pons on axial images and smaller than the mesencephalic tectum on the midline sagittal image (Fig. 2-2A and B).
![]() Figure 2-4 Fetal MR at 23 postconceptional weeks. A. Sagittal SSFSE image shows a thin corpus callosum (black arrows) and an enlarging cerebellar vermis (compare with Fig. 2-2A). |
hyperintensity between the cerebral cortex and the white matter on T2-weighted imaging studies (12,46), but they seem to begin to disappear rapidly after 25 weeks. The lateral ventricles and the cisterns around the brain stem and cerebellum are visible and more prominent at this age than in the mature infant; they are relatively smaller at 23 to 24 weeks (Fig. 2-4) than at 18 to 20 weeks (Fig. 2-3). When imaging at 3T, the third and fourth ventricles are easily visualized at this age unless a lot of motion artifact is present; if difficulty is encountered, waiting a few minutes for the fetus to calm down usually results in good images. The globi pallidi typically appear hyperintense on T1-weighted images starting at about 20 weeks. This likely represents premyelination changes, such as the appearance of proteolipid protein in oligodendrocyte processes (47).
![]() Figure 2-4 (Continued) B-E. Axial T2 FSE images through the cerebrum. Note the complete absence of myelination in the white matter at this age and the smaller germinal zones compared with the 20-week fetus illustrated in Figure 2-3. Note also that the subplate and intermediate zones, although still visible, are less conspicuous at this age than at 20 weeks. |
parietal lobes at about 28 weeks but remains visible in less mature areas such as the anterior frontal and temporal lobes for some time thereafter (12,46). Myelination is seen in some brain stem structures during this period, including the median longitudinal fasciculus (MLF; bright at 25 weeks on T1-weighted images, dark at 29 weeks on T2-weighted images), the lateral lemnisci (bright at 26 weeks on T1-weighted images, dark at 28 weeks on T2-weighted images), the medial lemnisci (bright at 27 weeks on T1-weighted images, dark at 30 weeks on T2-weighted images), and the superior and inferior cerebellar peduncles (bright at 28 weeks on T1-weighted images, dark at 29 weeks on T2-weighted images) (48). The basal ganglia and thalami are better seen at this age on MR imaging and have intensity similar to the cerebral cortex on both T1-and T2-weighted images, although not as hyperintense on T1-weighted images or as hypointense on T2-weighted images (Fig. 2-5I-L). The ventrolateral nucleus of the thalamus becomes hypointense compared with the remainder of the thalamus on T2-weighted images by about 25 weeks and hyperintense on T1-weighted images by 27 to 28 weeks, mostly due to its high cellularity and, possibly, to early myelination. The lateral ventricles, particularly the trigones and occipital horns, are less prominent at this age than at 22 to 23 weeks, probably secondary to both growth of the cerebral white matter and development of the calcarine sulci.
Table 2-2 Normal Fetal Brain Measurements (31) | |||||||||||||||||||||||||||||||||||||||||||||||||
---|---|---|---|---|---|---|---|---|---|---|---|---|---|---|---|---|---|---|---|---|---|---|---|---|---|---|---|---|---|---|---|---|---|---|---|---|---|---|---|---|---|---|---|---|---|---|---|---|---|
|

similar to that of T2-weighted images in adults in that white matter has lower signal intensity than gray matter. As white matter matures, its signal intensity increases relative to gray matter.
in signal intensity proceeds anteriorly; the genu is always of high signal intensity by age 6 months (Fig. 2-11). Typically, at 4 to 5 months of age, the splenium is high in signal intensity, while the genu is low in signal intensity. Maturation of the subcortical white matter, other than the visual and motor regions, begins at 3 months. The deep white matter matures in a posterior-to-anterior direction, with the deep occipital white matter maturing first and the anterior frontal and temporal white matter last. Peripheral extension of increasing hyperintensity of the subcortical white matter continues until approximately age 7 months in the occipital white matter and 8 to 11 months in the anterior frontal and temporal white matter (Fig. 2-12). Only minimal changes are seen on the T1-weighted images after 11 months, consisting of increasing signal intensity in the most peripheral (subcortical) regions of the anterior frontal, anterior temporal, and parietal white matter (23).
Low signal intensity is seen in the subcortical white matter of the cerebellar folia during the fifth to eighth month and the cerebellum reaches an adult appearance at approximately 18 months.
![]() Figure 2-10 MR of the brain of a normal 4-month-old. A-E. T1 images show rostral progression of the maturation of the internal capsule; the anterior limbs of the anterior capsule are now well myelinated. The splenium of the corpus callosum should always have high signal intensity by this age. Notice the isointensity of the cortical gray matter and subcortical white matter, resulting in difficulty in the identification of structural abnormalities at this age on T1-weighted images. F and G. Note the relative lack of change on the T2-weighted image from the neonate (Fig. 2-8). |
months, patches of low signal intensity are seen in the central centrum semiovale; this makes it difficult to distinguish white matter from the surrounding cortex because both have low signal intensity. By age 4 months, the intensity of the pre- and postcentral gyri is indistinguishable from that of adjacent gyri, which have also diminished in signal intensity (Fig. 2-10). Low signal intensity is seen in the optic tracts at birth in some patients and at age 1 month in most; the decrease in signal intensity extends posteriorly along the optic radiations during the subsequent 2 months; by 4 months of age, the calcarine fissure shows some low signal intensity. By age 4 months, the globi pallidi are slightly hyperintense compared to the putamina; they will remain hyperintense until 8 to 10 months, when the globi pallidi and putamina become isointense again.
the perirolandic region and gradually extends into the subcortical white matter in the subfrontal and anterior temporal regions; the process is essentially complete, even on modern images, by 24 to 28 months (Figs. 2-15 and 2-16). Thus, with the exception of the so-called terminal zones (see following section), white matter maturation, as assessed by visual inspection of MR imaging (quantified parameters, such as relaxation times and diffusion metrics, continue to evolve), is complete in the middle of the third year of life. During the progress of the peripheral extension of the low signal intensity within the white matter, the mantle of gray matter gives the appearance of progressive thinning, and the subcortical white matter often has a heterogeneous appearance.
Table 2-3 Ages When Changes of Myelination Appear | ||||||||||||||||||||||||||||||||||||||||||||||||||||||||||||||||||||||||||||||||||||||
---|---|---|---|---|---|---|---|---|---|---|---|---|---|---|---|---|---|---|---|---|---|---|---|---|---|---|---|---|---|---|---|---|---|---|---|---|---|---|---|---|---|---|---|---|---|---|---|---|---|---|---|---|---|---|---|---|---|---|---|---|---|---|---|---|---|---|---|---|---|---|---|---|---|---|---|---|---|---|---|---|---|---|---|---|---|---|
|
by dividing the appearance of the brain on T2-weighted spin-echo images into three patterns: (a) infantile (birth to 6 months), (b) isointense (8-12 months), and (c) early adult (10 months onward). In the infantile pattern, the cerebral white matter is hyperintense relative to gray matter, whereas the adult pattern shows hypointense white matter. The appearance of the isointense and early adult patterns is delayed in patients with developmental delay. A similar, but more complex, staging system, dividing brain maturation into five stages, has been proposed by Staudt et al. (79,80) Bird et al. (81) determined that the gray and white matter should be isointense by age 4 months on T1-weighted images and by 9 to 10 months on T2-weighted images. They considered the age at which gray and white matter are isointense as a critical factor in evaluating patients for developmental delay. Some authors have analyzed the images in terms of patterns and attempted to assess degree of, and delay in, maturation on the basis of the pattern (57,58,82,83,84). We choose to assess maturation through the use of the normal milestones described above, as it is a rapid and reliable method that does not require any postprocessing of the imaging data. Indeed, any of the methods described above are easy to use and reliable.

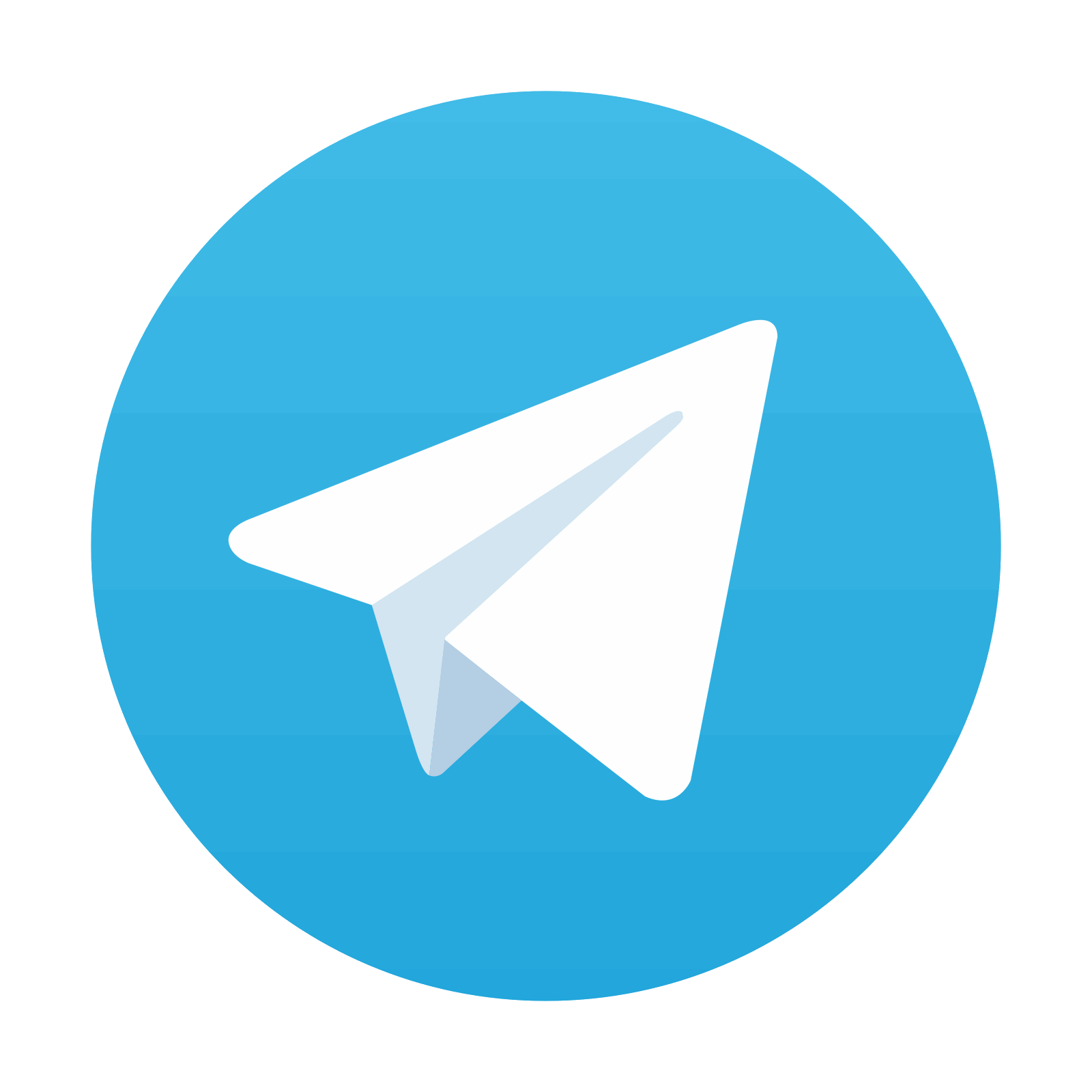
Stay updated, free articles. Join our Telegram channel

Full access? Get Clinical Tree
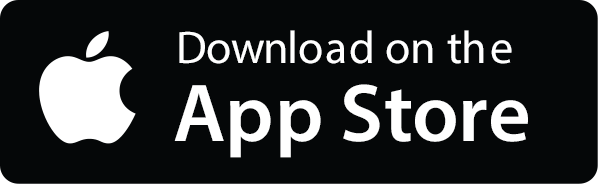
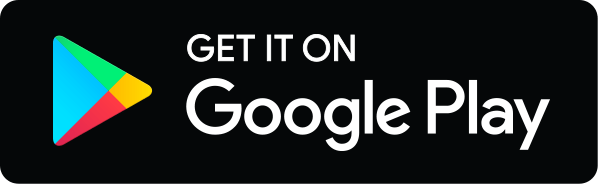